INTRODUCTION
Extraction methods for the recovery of polysaccharides and lipids from Ganoderma lucidum have been of growing interest because this mushroom contains active biomolecules that treat several diseases, such as arthritis, bronchitis, gastric ulcers, hyperglycemia, insomnia, nephritis, inflammatory diseases, and cancer [1, 2]. In addition, G. lucidum is known to have high antioxidant activity [3] and contains triterpenes and ganoderic acids A, B, H, T, C2 and Me, which have hepatoprotective, antihypertensive, hypochondrial, anti-HIV-1, and antihistamine actions [2, 4, 5]. G. lucidum is known in traditional Chinese medicine and has been used in the form of extracts and spores for various medical treatments. The spores of this Basidiomycota fungus, which belongs to the Ganodermataceae family [4], have a ductile structure; this property makes them resistant, and they are considered one of the hardest structures to break in nature [6, 7, 8].
Due to these characteristics, several methodologies have been applied to break mushroom spores, such as micronizers, air currents, knife mills, presses, enzymes, sonication, mechanical chemical extraction by mill, and reagents such as sodium hydroxide (NaOH), sodium bicarbonate (NaHCO3), and sodium carbonate (Na2CO3) [3, 9]. However, according to several studies on the breaking and description of biomolecules derived from G. lucidum spores, mechanical, chemical, and enzymatic breaking can produce variations in the release of compounds. Such variations are not well known in the literature. Therefore, it is important to conduct a study to analyse which alterations can cause the fragmentation of the spore walls and which molecules can be extracted. Thus, the aim of this research was to compare three breaking techniques for G. lucidum spores. A manual breaking method, an assisted mechanical breaking method using different types of spheres, and a microwave breaking method were employed. The best isolated and combined spore-breaking techniques were then evaluated by comparing the extracted proteins, carbohydrates, lipids, and antioxidant activity using Fourier-transform infrared spectroscopy (FTIR), light microscopy, scanning electron microscopy (SEM), and chemical characterization techniques.
MATERIAL AND METHODS
Breaking of Ganoderma lucidum spores
Various spore-breaking approaches were compared using 1 g of spores in each assay. In the first technique, the spores were macerated manually for 20 min. In the second, the spores were macerated with 20 g of spheres of different materials (steel/chrome, polylactic acid, polyvinyl chloride, glass, silica gel, and ceramic) for 20 min. In the third technique, the spores were exposed to the microwaves for 10 min at 1200 W, 127 V, 60 Hz, and 2450 MHz (model HMO22E-13-127V).
An optical microscope and a Neubauer chamber (American Optical Co.) of 0.1-mm depth were used to analyze spore breakage. For each breakage analysis, 0.1050 g of spores were subjected to the breaking method and then, diluted in 30 mL of distilled water. Counting was performed under augmentation of400x in five small squares, and the samples were evaluated in quadruplicates. The equation 1 was used to calculate the breakage percentage:
After this analysis, one portion of the sample was analysed by SEM to evaluate the changes occurred in the spore walls. For this process, the G. lucidum samples were dried in air stoves at 80 °C for 24 h. The samples were coated superficially with gold for microscopic analysis using a TESCAN VEGA3 LMU.
Preparation of G. lucidum extracts
Besides evaluating various spore-breaking techniques for G. lucidum, extraction was performed by diluting the spores in 16 mL of water pH 7 and incubating for 24 h at 30 °C and 120 rpm. Table 1 summarizes the breaking and extraction procedures.
Table 1 Methodologies used to prepare the Control, RM, BR and MBR1 Ganoderma lucidum extracts.
Extract | Breaking methodology | Extraction technique |
Control | Unbroken spores | The spores were diluted in 16 ml of pH 7 water and incubated for 24 h at 30 °C at 120 rpm |
RM | Manual maceration 1 g of spores for 20 minutes | |
BR | 1 g of spores macerated with the aid of spheres, for 20 minutes | |
MBR1 | 1 g of spores exposed to microwave for 10 minutes at 1200 W, 127 V, 60 Hz and 2450 MHz |
Evaluation of functional groups by FTIR
Sample p reparation
The functional groups present in the unbroken spores and in the aqueous G. lucidum extracts were determined using medium infrared spectroscopy (MIS) with VERTEX 70 (Bruker) equipment and the DRIFT accessory. A total of 64 scans were completed at 4 cm-1 resolution, without losing atmospheric compensation in the region between 4000 and 400 cm-1. The samples were crushed, pulverized, and oven dried. Approximately 20 mg of dried samples were mixed and homogenized with 100 mg of spectroscopic potassium bromide (KBr) to conduct the measurements. The FTIR analysis for proteins, carbohydrates, and lipids regions was performed [4, 5].
Spores rupture analysis using FTIR
The extracts of intact spores, manually macerated (RM) spores, steel/chrome sphere macerated (BR) spores and microwave plus steel/chrome sphere macerated (MBR1) spores were analysed with FTIR using stretching bands to describe changes in protein, carbohydrate and lipid extraction [10]. Extracts were evaluated using five regions: 3000 to 2800 cm-1 (asymmetric stretching of CH3 and symmetric stretching of CH2), 1660 to 1600 cm-1 (Amide I), 1400 to 1200 cm-1 (α-Helix in Amide III and unordered structures in Amide III), 1100 to 1000 cm-1 (C-O stretching if sugars), and 1750 to 1700 cm-1 (C= O stretching and α /β unsaturated carboxylic acids).
In each region, a bandwidth peak was analyzed. The first region received contributions of carbohydrates, lipids, and proteins, which appeared in bands at 2925 (asymmetric stretching of CH2) and 2855 cm-1 (symmetric stretching of CH2). For the second and third regions, the protein structure appeared at 1641 cm-1 (Amide I) and 1240 cm-1 (unordered structures in Amide III). The fourth sample showed the structure of sugars related to glucopyranose group at 1078 cm-1 (polysaccharide band C - O) [11, 12] and the fifth region received fatty acids and lipid contributions at 1746 cm-1 (C = O stretching) [10, 13, 14].
Chemical characterization
The total soluble protein (TSP) concentration was determined using the Bradford technique and the reading was conducted in the wavelength (λ) region of 595 nm of UV-VIS spectroscopy (SP2000 Visible SP 1105UV Spectrophotometer) with bovine serum albumin (BSA) as a standard [15].
The total phenolic compound content (TPC) in each extract was determined using the Folin-Ciocalteau method [16]. The results were expressed in mg of gallic acid equivalent per liter of sample (R2 0.9947).
The carbohydrate content was measured using the Antrone's method [17]. The concentration was determined via UV-VIS spectroscopy with λ = 620 nm (SP2000 Visible SP 1105UV Spectrophotometer). For composition analysis, 2 mg of extract was freeze-dried and hydrolyzed in 2 M of trifluoroacetic acid (TFA) at 110 °C for 2 h [18]. The determination of monosaccharide composition was conducted via reductive hydrolysis [19] using an extra reducing agent (borane 4-methylmorpholine complex or 4-MMB) before and after the pre-hydrolysis and hydrolysis steps [20]. The hydrolytic process was performed [21]. After acetylation [19] the alditol acetate derivatives were analyzed by gas chromatography-mass spectrometry (GC-MS)[21] and identified using their typical electron-impact fragmentation profiles and GC retention times [22]. A double hydrolysis reductive amination method was used to determine the absolute configuration of the monosaccharide constituents [23]. Chiral 1-amino-2-propanol was used to determine the ratio of d- and l-galactose and their 6-0-methyl derivative, whereas the configuration of 2-O-methylgalactose, 3,6-anhydrogalactose, and their 2-O-methyl derivatives were determined using chiral α-methylbenzylamine. The resulting alditol acetates derivatives were analyzed using GCMS [22].
The determination of antioxidant activity was conducted using 2.2-diphenyl-1-picryl-hydrazyl (DPPH). The DPPH method is based on determining the ability to scavenge an antioxidant compound using the stable DPPH free radical in the ethanolic medium. The DPPH method focuses on the UV-VIS region of l = 515 nm [24]. The DPPH solutions (0.004 mg.100 mL-1) were prepared and used immediately. They reflected the standard curve and were prepared with ascorbic acid solution in ethanol, with concentrations ranging from 0 to 200 µM. For each point of the standard curve, triplicate samples of absorbance were determined every 5 min until a regression coefficient (R2 = 0.991) was obtained. Each sample's radical scavenger activities were calculated according to the inhibition percentage (% Inb) of the DPPH radical, as shown in equation 2:
Where AC represents the absorbance values of the DPPH solution in ethanol, and AR represents the absorbance values of ascorbic acid + DPPH (reaction), respectively, at the end of the reaction.
The antioxidant activity value is expressed in µM (equivalent ascorbic acid-AA) from the regression coefficient of the calibration curve (equation 3):
Micro - and macronutrient contents were measured using metallic atomic optical emission in inductively coupled plasma (ICP-OES). The glassware used was placed in a solution of nitric acid (HNO3) 20% (w/v) for 24 h and subsequently washed with deionized water. All reference solutions were prepared with ultrapure water with a resistivity of 18.2 MΩ cm-1 (Millipore, Berford, MA, USA) coupled to a model 534 glass water distiller. One gram of material was transferred to a 250-mL volumetric flask, to which 5 mL of concentrated HNO3 and 4 mL of H2O2 (30%) were added. After reaching the boiling point, the system was kept hot for 40 min. Then, the solution could cool to room temperature, and it was filtered quantitatively with a filter paper. Finally, it was completed to 100 mL in a volumetric flask. The same procedure was performed for all samples. The three samples from the control group were prepared in the same way. The levels of metals (micro- and macronutrients) were measured using ICP-OES on a VARIAN model 720 ES in conjunction with the axial arrangement and solid-state detector.
To determine total lipid content in G. lucidum spores, 1.0 g samples of broken and unbroken spores were assayed in triplicate. In a conical tube, 5 mL of CHCl3, 10 mL of CH3OH and 4 mL of water were combined. The tube was placed in a shaker for 30 min at 120 rpm. Then, 5 mL of CHCl3, 5 mL of Na2SO4 (1.5 %) and the samples were agitated in a vortex for 2 min and centrifuged at 1500 g for 2 min. After extraction, the solvent was evaporated in an oven at 50 °C for 8 h [25].
RESULTS AND DISCUSSION
Breaking of Ganoderma lucidum spores
Manual maceration of G. lucidum spores produced no visible breakage when evaluated by optical microscopy (400x). The maceration with the aid of 1-mm diameter steel/ chrome spheres produced a breakage rate of 97.58 ± 1.41%. Other types of spheres (polylactic acid, polyvinyl chloride, glass, ceramic, and silica gel) produced no breakage. After obtaining mechanical breakage with the steel/chrome spheres, three maceration times (5, 10 and 15 min) were tested. For each time, the breakage percentage was 89.10 ± 6.66%, 97.48 ± 3.61%, and 98.58 ± 2.89%, respectively. Ten min of maceration were enough to obtain almost 100% breakage.
Microwave exposure at 1200 W for 10 min produced no ruptures observable with optical microscopy (400x). However, with SEM, it was possible to observe slight fissures in the spore walls (figure 1). Similarly, when images of the maceration techniques with and without the aid of steel/chrome spheres were analysed, the best breakage was obtained using the spheres because they fragmented the entire spore wall.
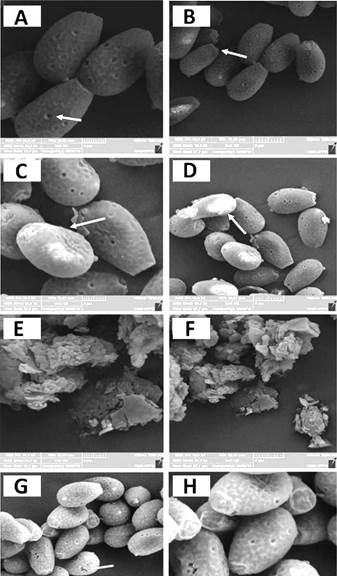
Figure 1 Spore wall breakage analyzed by SEM. A-B) Control spores with no breakage. C-D) Mild fractures and spore wall sinking after using the maceration technique for 20 minutes. E-F) Complete fragmentation of the spore wall when macerated with steel/chrome spheres. G-H) Fissures and sinking all spore walls exposed to microwaves for 10 min.
Ganoderma lucidum spores have two internal caps: the exospoirum and the myxosporium [8, 10]. These structures have high ductility, a property that provides the spore with resistance [7]. For this reason, various methodologies have been developed to break G. lucidum spores, including ultrasonication, ultra-fine grinding, high-speed centrifugal grinding, shear grinding, and enzymatic grinding.
These have provided between 80 and 100% breaking efficiency [6-8, 26-29]. Nevertheless, they require complex equipment, whereas maceration with steel/chrome spheres provides an easier, more economical way to fragment G. lucidum spores, as reported in the present study.
The FITR breakage analyses showed that the BR samples obtained with the aid of steel/ chrome spheres underwent changes in the structure of fatty acids, lipids, and proteins.
This occurred due to differences in the asymmetric stretching of CH3 at 2955 cm-1 band found at 2967 cm-1, indicating a difference in the extracts' general structure. The spectral vibration from unordered structures (Amide III at 1240 cm-1) changed to 1231 cm-1. Contributions of C=O spectral vibration from fatty acids and lipids at 1746 cm-1 were found at 1738 cm-1. For microwave-exposed spores, the more important changes were observed in the spectral vibration of proteins. This can be attributed to stretching vibration from unordered structures such as Amides I and III, for which spectral vibrations were found at 1630 cm-1 and 1629 cm-1, respectively. The change in wavelengths showed that there was a rupture in the chemical structure of the G. lucidum spores' walls (table 2).
Table 2 Differences among wavelengths in the walls of unbroken spores, spores broken with steel/ chrome spheres, and spores exposed to microwaves.
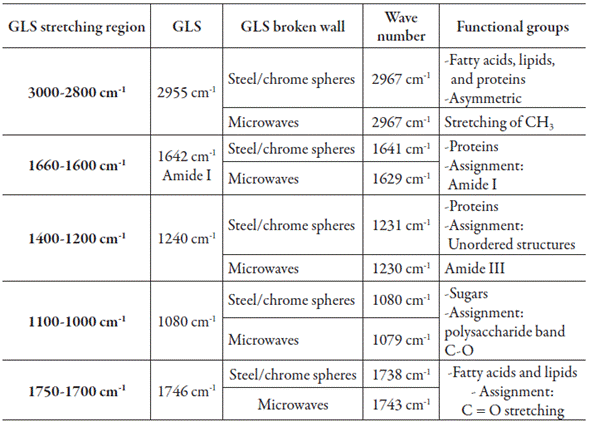
Data compared with Zhu et al. [9], Wang et al. [13] and Wang et al. [14]. GLS Ganoderma lucidum spores.
After evaluating the breaking techniques, it was necessary to assess the biomolecule extraction capacity. For the FITR analysis of the control extract, the proteins, fatty acids, and lipids were observed at 2932 cm-1, proteins were observed at 1641 and 1228 cm-1, and sugars were observed at 1079 cm-1. For the RM the extract, proteins were detected at 1635 cm-1, and carbohydrates were detected at 1079 cm-1. For the BR extract, lipids, fatty acids, and proteins were detected at 2929 and 2852 cm-1, proteins were detected at 1641 and 1235 cm-1, and sugars were detected at 1078 cm-1. Finally, for the MBR1 extract, the contribution of fatty acids, proteins, and lipids was 2933 cm-1. For proteins, it was 1647 and 1227 cm-1, and for carbohydrates, it was 1078 cm-1 (table 3).
Table 3 Presence ofvarious extracted biomolecules by bands in Control, RM, BR and MBR1 extracts.
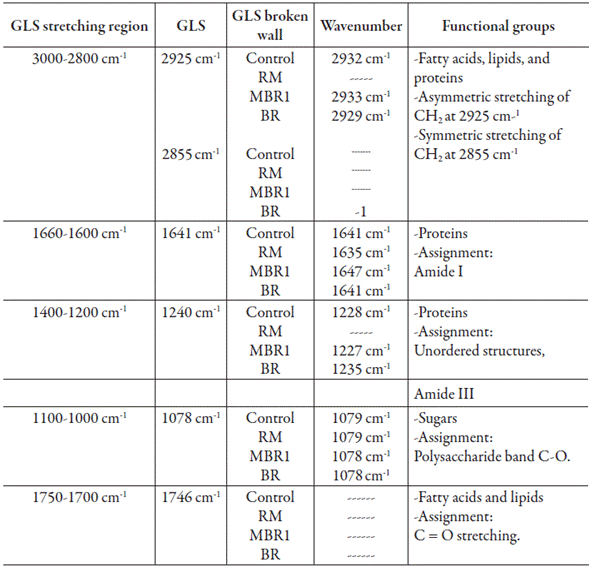
Data compared with Zhu et al. [9], Wang et al. [13] and Wang et al. [14]. GLS Ganoderma lucidum spores.
In addition, by identifying stretching bands for various compounds, the FTIR technique makes it possible to compare the proportions of carbohydrates, lipids, and proteins to better understand which compounds were extracted using each methodology. For this purpose, the band heights (H) of the stretches were analysed at 1748 cm-1 for α and β lipid esters (C=O, baseline: 1771-1723 cm-1), at 1642 cm-1 for Amide I proteins (baseline: 1700-1581 cm-1), and at 1079 cm-1 for carbohydrates (baseline: 1130-951 cm-1) as proposed by Wang and Yano [13, 14, 30], who evaluated the proportions by comparing proteins with lipids (H1642/H1748), carbohydrates in relation to proteins (H1079/H1642), and carbohydrates between lipids (H1079/H1748). We found a significantly higher proportion of proteins than lipids in the control extract, a higher proportion of carbohydrates than proteins in the MBR1 extract, and a higher proportion of carbohydrates than lipids in the control extract (table 4).
Table 4 Mean values of the ratio chains H1642/H1748, H1079/H1642, and H1079/H1748 in Ganoderma lucidum spores in various extracts.
Ratios | ||||
---|---|---|---|---|
Control | BR | MBR1 | RM | |
H1642/H1748 | 01.18 | n.d. | n.d. | n.d. |
H1079/H1642 | 01.23d | 00.83C | 40.51E | 00.26B |
H1079/H1748 | 01.46 | n.d. | n.d. | n.d. |
Different letters in rows indicate statistically significant differences at p<0.05. n.d: not determined. 1748: C=O stretching, 1642: Amide I, 1079: C-O polysaccharide band.
The most soluble compounds under the conditions studied were carbohydrates and some proteins. It is known that small molecules combine with macromolecules to form aquo-complexes with different biological functions [14]. The MBR1 extract contained a major proportion of carbohydrates depending on the regions studied, which indicates that the FTIR approach can be used to study the extracted biomolecules. This result also indicates that various methodologies for breaking G. lucidum spores must be studied since different biomolecules are extracted from the spores. The overall process for the extraction of bioactive molecules is represented in figure 2.
Protein, polyphenol, carbohydrate, and antioxidant content
The concentrations of extracted proteins were between 12.24 and 15.55 mg per 1g of spores and showed no statistical difference (table 5). For polyphenols, the MBR1 extract presented the most significant quantity (2.21 ± 0.01 mg per 1g of spores), followed by the BR extract (1.79 ± 0.01 mg per 1g of spores), whereas the control and RM extracts contained no detectable levels. For polyphenols, the process used in the MBR1 and BR techniques facilitated the recovery of a greater quantity than the processes described in the literature: 0.61 ± 0.05 mg.100g-1 of dry weight [3, 26]. Polyphenols are important because they have lanostane-type triterpenes that function against several types of cancerous cells.
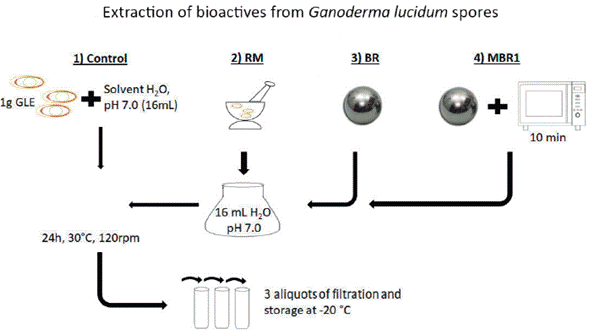
Figure 2 Extraction process of bioactive molecules. GLE: Ganoderma lucidum spores; RM: Manual maceration; BR: Maceration with spheres; MBR1: Microwave exposure plus maceration with steel/chrome spheres.
For carbohydrate analysis, the MBR1 process presented 19.80 ± 0.03 mg per 1 g of spores. The extraction of carbohydrates from the MBR1 extract was higher than the reported yield of 8.65 ± 0.46 g per 100 g of dried spores [3]. Ganoderma lucidum carbohydrates are important in antitumor therapies due to their polysaccharide content, which includes β-D-glucan chains [18]. For the monosaccharide analysis, the results depended on the methodology used. Glucose was abundant in all extracts, but the BR and MBR1 extracts contained other abundant monosaccharides, such as galactose and mannose, respectively. In the BR extract, galactose was the second most abundant. The MBR1 extract contained the same proportion of arabinose and mannose. Proportions of monosaccharides in RM extract were not equal, but xylose was the second most abundant. These two extraction methods were more effective in isolating a major heterogeneity of monosaccharaides (table 5).
Table 5 Content of proteins, polyphenols, carbohydrates, antioxidant power, and monosaccharides in the Control, RM, BR, and MBR1 extracts.
Extracts | Proteins | Polyphenols | DPPH | Carbohydrates |
---|---|---|---|---|
mg/1 g GLS | mg/1 g GLS dw | %Inb | mg/1 g GLS dw | |
Control | 12.24 ± 00.09 A | n.d. | 46.83 ± 00.08 AB | 01.24 ± 00.03B |
RM | 15.55 ± 00.05 A | n.d. | 47.85 ± 00.07 AB | 11.92 ±00.01 A |
BR | 13.08 ± 00.00 A | 01.79 ± 00.01A | 57.22 ± 00.09 B | 11.26 ±00.06 A |
MBR1 | 14.03 ± 00.06 A | 02.21 ± 00.01B | 45.13 ± 00.03 A | 19.80 ±00.03 C |
Monosaccharides | BR (%) | MBR1 (%) | RM (%) | Control (%) |
Rhamnose | 01.76 | n.d. | n.d. | 04.91 |
Fucose | 04.19 | n.d. | n.d. | n.d. |
Arabinose | n.d. | 11.72 | 01.33 | n.d. |
Xylose | 03.90 | 01.41 | 09.88 | 10.92 |
Mannose | 10.15 | 12.07 | 04.39 | 05.83 |
Glucose | 62.19 | 73.54 | 77.73 | 67.74 |
Galactose | 17.21 | 01.23 | 06.33 | 09.36 |
n.d: not determined; A and B show statistical differences between samples; dw: dry weight. GLS: Ganoderma lucidum spores.
The monosaccharides found corresponded to those reported by Yue et al. [28] and Soccol et al. [29], the majority being identified as glucose, mannose, and galactose. Fucose and arabinose were absent in the control extract. The BR extract contained the most significant antioxidant activity, with 57.22 ± 0.09 % (table 5). The BR extraction process had the best results for antioxidant recovery, as the spores had the highest concentrations of antioxidants compared to other parts of the fungus [3]. Various molecules, such as carbohydrates, phenols, and proteins, also have antioxidant activity. Therefore, breaking the spores increases the release of antioxidant compounds.
The MBR1 and BR extracts' micro- and macronutrients presented less than 0.01 mg.kg-1 of V, Se, Pb, Ni, Mo, Cd, Cu, and Co. The MBR1 extract had the lowest B content (9.95 mg.kg-1) between the two extracts. High metal values in the broken spores are presented in table 6. Spores broken with steel/chrome spheres had slightly higher Cu (17.34 ± 00.01 mg.kg-1) content compared to the unbroken spores (16.08 ± 00.01 mg.kg-1). Na values (1053.15 ± 00.06 mg.kg-1) were higher for intact spores and lower for MBR1 spores (189.91 ± 00.04 mg.kg-1). The spores' integral and broken walls had less than 0.01 mg.kg-1 of Co, Cd, Mo, Ni, Se, and V.
Table 6 Heavy metal content in G. lucidum extracts and spores.
Metal | BR * (mg/kg) | MBR1 * (mg/kg) | Spores of G. lucidum broken (mg/kg) |
Al | 23.82 ± 00.01A | 30.18 ± 00.02A | 1,071.53 ± 00.14B |
B | 24.92 ± 00.01A | 09.45 ± 00.01B | 57.75 ± 00.01C |
Ba | 24.92 ± 00.00A | 00.90 ± 00.00A | 57.75 ± 00.00B |
Ca | 270.05 ± 00.03A | 162.99 ± 00.01A | 3,009.31 ± 00.19C |
Co | < 0.01 | < 0.01 | < 0.01 |
Cu | < 0.01 | < 0.01 | 17.34 ± 00.01A |
Cd | < 0.01 | < 0.01 | < 0.01 |
Fe | 13.30 ± 00.00A | 06.85 ± 00.00A | 2,321.24 ± 00.09B |
K | 152.70 ± 00.04A | 114.44 ± 00.03A | 2,349.59 ± 00.39C |
Mg | 42.40 ± AB | 21.32 ±A | 899.30 ± C |
Mn | 02.79 ± 00.00A | 02.16± 00.00A | 41.28 ± 00.00C |
Mo | < 0.01 | < 0.01 | < 0.01 |
Na | 233.52 ± 00.04A | 189.91 ± 00.04A | 780.01 ± 00.05B |
Ni | < 0.01 | < 0.01 | < 0.01 |
P | 199.24 ± 00.03A | 153.78 ± 00.03A | 1,886.87 ± 00.08C |
Pb | < 0.01 | < 0.01 | < 0.01 |
Se | < 0.01 | < 0.01 | < 0.01 |
V | < 0.01 | < 0.01 | < 0.01 |
*Note: a and b show statistical differences between extracts. A and B show statistical differences between samples.
All spore and extract samples had low contents of Co and Pb (< 0.01 mg.kg-1). Spore breakage using the steel/chrome spheres does not present a risk to public health because the metals' concentrations were below the recommended levels. The toxicity of these elements was described by European Commission 1881/2006 [30-31] where Pb content of edible fungi is set at a minimum of 0.10 mg.kg-1, and Cd levels are set at 1.0 mg.kg-1. In both extraction processes, the heavy metal quantity was below that of unbroken spores.
Lipid determination was performed for broken and unbroken G. lucidum spores; these values were 25.09 ± 3.65% and 7.0 ± 1.41%, respectively. These results indicate a greater release of lipids after spore rupture due to assisted maceration with steel/chrome spheres. This lipid level of 25% is within the range of 26.9% to 23.3% previously reported for G. lucidum [32]. Although the rupture process used in this study yielded a high lipid concentration, the extraction process did not allow for their recovery. Other methods can be used to obtain lipids. For example, lipids can be extracted using sonication at a concentration of 23.74% by Soxhlet, and at 29.50% by supercritical CO2 at 35 MPa [33].
CONCLUSIONS
The most effective methods for breaking the walls of G. lucidum spores were the BR and the MBR1 processes. The FITR analysis of the chain lengths was an easy tool for the preliminary qualification study and for monitoring the methods used to extract the different chemical compounds present in the G. lucidum spores and extracts. This method showed that protein structures were modified during the MBR1 process, whereas they were preserved along the BR process. The MBR1 process showed the highest proportion of carbohydrates and polyphenols, thereby maintaining antioxidant potential. Finally, the rupture and the chemical extraction methods used maintained antioxidant activity and extracted significant amounts of biocompounds.