INTRODUCTION
From pigments for the textile industry and even for application in photodynamic therapy, phthalocyanines are among the most synthesized porphyrin derivatives in the laboratory due to the wide range of applications they have. These compounds, although they are macrocycles coordinated with some metal, have a very reasonable structural relationship with porphyrins and porphyrazines (figure 1). Because the three structures contain a tetra-aza compound formed by four heterocyclic pyrrole units. As a consequence, and also due to the particular spatial configuration of all tetra-aza, the nitrogen atoms of the pyrrole units are "found" in the center of the macrocycle. This means that porphyrin, porphyrazine and phthalocyanine can chelate metals and form a coordinated complex, since two of the four nitrogens involved form a coordinated bond with the metal ion in the center of the structure [1].
Despite similarities, there are some differences between these three compounds. For example, both porphyrazine and phthalocyanine have a carbon-nitrogen bond that serves as a bridge for the porphyrin structure in question, instead of the methine group characteristic of conventional porphyrin. However, it can be seen that together with the heterocycle an aromatic ring is added, which corresponds to an isoindole unit, in the phthalocyanine structure and that the other two structures do not contain. Therefore, it can be said that phthalocyanine is a tetra-isoindolic compound while for porphyrin and porphyrazine they are tetra-pyrrolic. In addition, it is noteworthy that phthalocyanine has a much higher and varied metal uptake potential than its analogous structures. Fundamentally because they are being able to coordinate with up to 70 elements of the periodic table and, in a wide manner, the general characteristics of these compounds depend largely on the metal to which they complex [1]. Phthalocyanine is a macrocyclic compound that contains a conjugated system of 42 n electrons, and in whose benzene rings it can present α and β substituents (figure 2) [2].
Thus, the molecule phthalocyanines capable of exhibiting a marked aromatic character of according to Hückers rule (4n + 2), whose number "n" has a value of 10. Therefore, a phthalocyanine structure has characteristics of planarity. This combination of aromaticity and planarity with an extended n system that includes four aromatics fused of benzene origin, it is essential to confer the intense colors characteristic of these compounds in a wavelength range from 650 to 750 nm. In addition,, aromaticity gives the phthalocyanine molecule great thermal and chemical stability which make it a very versatile structure. Due to these chemical peculiarities, phthalocyanines are very widely used as synthetic pigments in the textile industry [3]. Likewise, due to their aromatic nature, phthalocyanines have greater absorption capacity in the Near-Infrared Region compared to other porphyrins [4].
During a good part of the 20th century, the synthesis and characterization of the various phthalocyanines has been an important target of study due to its little information about their chemical behavior. It was not until 1999, where investigations were carried out on the effects of the solvent and the metal used in the synthesis of phthalocyanines at room temperature. This work demonstrated that lithium metals can react with several long chain primary alcohols, most especially 1-octanol. This mixture significantly improves the reaction yield (over 65%) in the synthesis of tetra-substituted phthalocyanines using a nitro phthalonitrile derivative as a reaction intermediate. In addition to this, the formation of phthalocyanines could be observed with moderate yields (around 30%) at room temperature starting from reaction intermediates such as unsubstituted phthalonitrile, 4-neopentoxyphthalonitrile and methoxylated phthalonitrile in ethanol solutions with calcium. The formation of phthalocyanines from phthalonitrile could also be evidenced, with very low reaction yields (below 25%), when they reacted with magnesium, zinc or iron in methanol [5].
Six years later, as a result of improvements in spectroscopic and analytical techniques and the accelerated advance of mechanistic concepts, the synthesis and photophysical characterization of phthalocyanines metalized with zinc, cadmium and mercury could be carried out with low yields. The latter has been studied sparingly for years. In this way, absorption and fluorescent emission data could be obtained in plenty types of solvents such as DMSO, THF, CHCl3, among others. For example, Zinc Phthalocyanine (ZnPc) was observed to have a maximum wavelength in the Q band at 672 nm and a fluorescent emission at 682 nm in DMSO. Furthermore, in this same solvent, cadmium phthalocyanine (CdPc) has an absorption at 679 nm in the Q band and a fluorescent emission at 691 nm, while mercury phthalocyanine (HgPc) has an absorbance at 684 nm and a fluorescent emission at 698 nm. Data on fluorescence quantum yields and singlet oxygen formation were also reported. In the first case, using DMSO as solvent, the order of fluorescence was ZnPc > CdPc > HgPc; while for singlet oxygen in the same solvent it was the opposite (HgPc > CdPc > ZnPc). With this, it was confirmed then that phthalocyanines maintain different characteristics according to the periodic properties of the metal to which they are bound [6].
Subsequently, there was a period of 6-7 years where scientific research on phthalocyanines increased exponentially. All this thanks to the advanced development of the mechanistic and photochemical or photophysical concepts of porphyrin compounds and the great interest in carrying out research in the biomedical area. From then on, phthalocyanines began to show quite significant therapeutic potential. For example, in 2013, Wang et al. synthesized for the first time a water-soluble zinc phthalocyanine, substituted with 3-(4-methoxybenzylamino) propanoic acid (ZnCNPc). As with previous research, absorbance and fluorescent emission data were reported for substituted and unsubstituted zinc phthalocyanine. They pointed out that, for the second case, the Q absorbance band is 680 nm and 341 nm for the B band in dimethylformamide (DMF), which are data very similar to those reported in previous years. From this, studies were carried out on the interaction of substituted zinc phthalocyanine (ZnCNPc) with "Calf Thymus" DNA (CT-DNA), using spectroscopic methods such as the Ethidium Bromide Displacement Assay. It was determined that ZnCNPc has a great affinity with Calf Thymus DNA, coupled with the great ability to damage this DNA in a photoinduced manner due to the large amounts of 1O2 that ZnCNPc can generate. In this way, another investigation was added that showed that phthalocyanines have a favorable effect from a biomedical point of view. However, the reaction yields were again very low [7].
Likewise, a year later, as a reinforcement of the arduous work that had been carried out for more than a decade in the field of porphyrin compounds, an association of Turkish universities investigated the synthesis and thermal stability of different kinds of phthalocyanines (Zn, Co, Cu, among others), which are widely used in the textile industry. It was determined that the Cumetalized Phthalocyanine has greater thermal stability, since it can only decompose at a temperature of 692.05 K. However, good thermal stabilities were also observed in the other compounds, which confirms the great potential that phthalocyanines have as dyes, coupled with the therapeutic potential. The photochemical and photophysical properties were studied. The same fluorescence and absorbance values for Zn Phthalocyanine reported by research in previous years stand out, with a Q band of 672 nm and a fluorescent emission of 682 nm. Again, low reaction yields persisted [8].
Finally, the problem of reaction yields was solved when Dutch researchers in 2019 designed a simpler synthesis of metal-free and metalized phthalocyanines with Zn, Cu, Mn, Fe and Co, by a direct, one-step method and an indirect two-step method.
In the first case, with reaction yields of up to 70%, compounds such as phthalonitrile, n-pentanol and DBU are required, refluxed for two hours. In this way, dissolving phthalonitrile in n-pentanol produces a milky liquid, which turns orange when DBU is added. The solution then turns a dark blue-purple color upon heating, depending on the phthalocyanine, which indicates the formation of the porphyrin ring. At the same time, since this reaction is based on the cyclization of phthalonitrile, the process must be carried out under an inert atmosphere. Basically, because the humidity of the air can hydrolyze the nitrile groups that would form unwanted by-products. Very valuable infrared spectroscopy data were also reported [9].
METHODOLOGY
We have carried out a meticulous search for scientific works related to the subject between 2020 and 2021, the most of them were written in English. Various websites have been used, including Research Gate, American Chemical Society (ACS), Springer, Google Academics, among others. It has been tried that most of the studies that support our work are from specialized and refereed journals in the area of photochemistry, photobiology and pharmacy, for example, the Journal of Photobiological and Photobiological Science, Pharmazie Magazine. Hence, in any scientific journal in the areas of organic chemistry and medicinal-pharmaceutical chemistry. Specialized digital books have also been used in the area, as well as doctoral theses that are related to our study.
As a result, for studying phthalocyanines' properties, the work can be separated into two issues: synthesis of the metalized phthalocyanines and photochemical and photo-biological properties.
Synthesis of the metalized phthalocyanines
In figure 3, it is possible to visualize different ways in which a phthalocyanine can appear according to its content in the center of the molecule. For example, phthalocyanines, in addition to the metallo phthalocyanine form that contains a coordinated metal in the center of the structure, can exist as a phthalocyanate di-anion. In this case the ligand corresponds to a negative charge of -2 and can be oxidized and reduced on various occasions. There is also a free phthalocyanine, where the ligand corresponds to two cations bound by two nitrogen atoms without altering the flat structure of the molecule. This issue must be emphasized since there are certain metallo phthalocyanines that are capable of chelating two cations at the same time, as long as the latter have an oxidation state of+1. The consequence of this is that the molecule stops being flat and becomes a concave structure, causing a distortion and relative decrease in stability in the molecule [10].
This distortion in the planarity of the phthalocyanines as well as the porphyrinics, the central metal has a significant effect on the stability of their excited states. For example, a particularity observed on several occasions is the ability of metallophthalocyanines to form large stacked assemblages with themselves and other compounds. Evidence suggests that, when the metallophthalocyanines of these assemblages have transition metals, a relaxation pathway is observed in the excited state consisting of an interaction of the conjugated n system and the d orbitals of the central metal. This is particularly true for copper and zinc phthalocyanines, since they do not fluoresce or phosphoresce in emission experiments at room temperature. Consequently, an alternative mechanism of ultra-rapid deactivation of the initially excited state is suggested due to the type of coordinated metal, being able to partially inhibit the fluorescent and phosphorescent capacity of some metallo phthalocyanines[10].
The synthesis of phthalocyanines can be achieved in different yields, depending on the reagent, by cyclotetramerization reactions ofdifferent phthalocyanine precursors (figure 3). Commonly, for example, phthalimide, diiminoisoindoline, phthalic anhydride, or phthalonitrile are used in high-boiling solvent and in the presence of a strong base such as 8-diazabicyclo undec-7-ene (DBU) [11].
These reactions require high temperatures, generally above 180 °C, which, depending on the number of synthetic steps, can lead to side products and reduce yields. Therefore, it is preferable to use the synthesis method by cyclization of phthalonitriles in the presence of base (figure 4). Basically, because phthalonitrile is highly reactive and better reaction yields of up to 90% are obtained in a single synthesis step. In addition, the reaction time is much shorter compared to other methods [9, 11].
After a bibliographic study and several tests carried out in our laboratory, we have selected Zn-phthalocyanine as a good candidate to develop our studies. Taking as important characteristics its photo-stability in UV-A and visible irradiation, as well as its solubility in water [12]. In addition, a higher antibacterial effectiveness was preliminarily observed on E. coli compared to those of Cu and Ni. Similarly observed with meso-tetraphenyl-porphine-tetracarboxylic acid and their Ni, Cu and Zn [13].
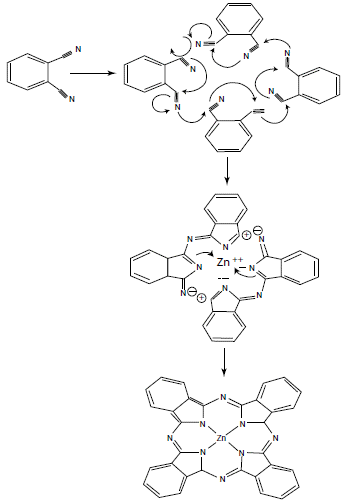
Figure 4 Reaction mechanism of phthalonitrile cyclization for the synthesis of phthalocyanines [14].
In recent years, plenty techniques have been developed in order to reduce inconveniences and increase reaction yields. One of these techniques has been used in the synthesis of porphyrins, phthalocyanines and related compounds: the use of microwave irradiation. This technique is based on non-conventional thermal heating by using microwave, which could perfectly be a substitute for conventional thermal heating that allows the use of ionic liquids instead of common solvents. This methodology is currently receiving special attention, fundamental because better reaction yields and greater purity have been achieved in the products. In addition, as there is the possibility of synthesis in the absence of solvents, interest in synthesis via microwave has increased even more, especially in the field of green and sustainable chemistry [11-16].
Photochemical and photobiological properties
Porphyrin derivatives are very good photosensitizing compounds. Because they are able to produce large amounts of singlet oxygen and other reactive oxygen species (ROS), which are obviously very powerful oxidizing components. As a consequence, the biological or medical utility of porphyrin compounds is very broad. As in the case of phthalocyanines, its use for the treatment of photodynamic cancer, the photooxidation of toxic molecules and the photoproduction of important intermediates for various chemical products, is precisely due to its great photosensitizing capacities. This is due to the fact that phthalocyanines have the capacity to generate large concentrations of ROS with an absorption spectrum equal to or greater than 650 nm, resulting in a great advantage especially in photodynamic therapy against viruses, bacteria and cancer [10].
Photodynamic therapy is a treatment that has shown positive results in the investigation of a variety of oncological, cardiovascular, dermatological and ophthalmic pathologies, as well as in vascular surgical interventions. Currently, it is in clinical use for the treatment of bacterial infections, with the advantage over classical antibiotic treatments that no resistance is generated. In the field of clinical oncology, various PDT protocols are used routinely. Photofrin® was the first to be approved for PDT, and nowadays it is used worldwide for the treatment of lung, esophageal and other tumors. Still, one of the main disadvantages of the therapies currently in use is delayed photosensitivity, which can lead to hyperpigmentation, erythema, and edema. Due to this reason, and the fact that even today a suitable PDT has not been found to treat the diversity of existing tumors, the investigation of new photosensitizer and new therapeutic approaches is a relevant task.
Singlet oxygen is a highly oxidizing species that can be generated within cells through a process of photosensitization. It can generate oxidative stress, that is, a disorder in the balance between the number of oxidants and antioxidants in various biological systems through lipid peroxidation processes, hemolysis of erythrocytes and damage to neutrophils. Furthermore, due to its high reactivity with DNA, the generation of photosensitized singlet oxygen can cause severe damage to the genome. This, as already mentioned, can be useful from a medical point of view since the generation of singlet oxygen and other free radicals cannot only cause damage to DNA but also to viral proteins and cancer cells. In addition, as it is a highly selective species, cell viability in in vitro tests is usually very high, which is very advantageous for its application in photodynamic therapy [17, 18].
Singlet oxygen is a diamagnetic species that can have two paired electrons of different spin in one π* orbital or also in two different π* orbitals. This species is derived from the excitation of another species from its lowest energy state, molecular oxygen, which is oxygen in its triplet form. Other free radicals can be produced from the latter. For example, superoxide anion can be generated by transferring a single electron. In turn, the latter can generate hydrogen peroxide through electronic transfer and the reduction of this generates hydroxyl radicals [18]. However, singlet oxygen cannot be generated directly from molecular oxygen. This is because the direct excitation of triplet molecular oxygen (3O2) to the type 1Ag singlet oxygen form (1O2) is electronically prohibited. As a consequence, singlet oxygen (1Δg) can generally be produced by the transfer of energy from a photosensitizer to 3O2. This occurs through a photosensitizer excited in a singlet S1 state, which becomes a triplet T1 state, which transfers its energy to 3O2 to generate singlet oxygen (1Δg). This process pertains to a type II photosensitizing reaction [2] (figure 5).
Hydroxyl and peroxyl radicals can also be generated from a photosensitizing reaction, in which a type I mechanism is carried out. In this case, an electron transfer occurs between the triplet state photosensitizer and an acceptor, resulting in the formation of semi-reduced and semi-oxidized radical type species. Subsequently, the previously formed radicals can undergo a great variety of reactions with other substrates, solvent molecules or even, and more frequently, with oxygen. It should be noted that, when this reaction involves oxygen as an electronic acceptor, the path followed is the one already shown in figure 6, where peroxyl and hydroxyl radicals are produced from the superoxide anion. The latter produced by the electronic transfer between molecular oxygen and the photosensitizer in its triplet form (figure 6) [18].
There are several direct and indirect methods for the generation and detection of singlet oxygen for its subsequent quantum yield calculation. One of the most important methodologies is the one developed by Kraljic and El Moshni in 1978, which is an indirect photosensitized technique for the generation and detection of singlet oxygen with a mechanism similar to that presented in figure 7, known as the Histidine test. This UV-VIS detection technique consists in the use of a trapping molecule, known as p-nitrosodimethylaniline (RNO), and of a 1O2 acceptor compound that induces the "discoloration" of the trapping molecule. Commonly, the most widely used 1O2 acceptor compounds in this test are imidazole and histidine. These are capable of trapping singlet oxygen derived from some photosensitizing compound. By doing this, they allow the formation of ring peroxide compounds, which are able to cause the "discoloration" of the RNO when reacting with them (figures 7 and 8) [17, 19].
It should be noted that the "discoloration" of RNO can be followed by means of a calibration curve at a wavelength of 440 nm, similar to that shown in figure 8. Furthermore, it should be emphasized that, to increase the reliability of the technique, a standard test of some photosensitizer whose quantum yield results are known should be available. The most widely used photosensitizer for this purpose is Rose Bengal, whose singlet oxygen quantum yield is typically 0.76. In this way, the development of the methodology used for tests with other photosensitizers can be verified, together with the increase in the accuracy of the test [17].
There is another very simple and sensitive spectrophotometric method for the detection of 1O2, which consists of trapping it with diphenylanthracene (DPA). This compound has the particular characteristic of forming peroxide compounds when reacting by cycloaddition with singlet oxygen, once the photolysis of a compound or the type II reaction in a photosensitizer has occurred. The disappearance kinetics of DPA during this reaction can be followed spectrophotometrically, observing the decrease in absorption peaks as a function of irradiation time at a wavelength of 374 nm (figure 9) [20-28].
Hydroxyl radicals have, like singlet oxygen, an oxidizing capacity in DNA. Basically because they are very reactive electrophilic reagents that can undergo three types of reactions: (1) addition to C - C and C - N double bonds, (2) extraction of hydrogens and (3) electron transfer [23]. The quantum yield of hydroxyl radicals is the total number of emitted photons divided by the number of molecules of the reagent consumed by a photo-oxidation reaction. This can be determined by chemiluminescent oxidation of luminol, which can be carried out by two different pathways, each one of those is dependent on the concentration of the oxidizing species present. Experimentally, the amount of hydroxyl radicals produced can be determined by luminal-chemiluminescence, after irradiation (with wavelengths between 400-900 nm) of the oxidizing compounds. To do this, it must be taken into account that the general stoichiometry of the reaction of a molar ratio of two for base, one for oxygen and one for luminol. Thus, a quantity of hydrogen peroxide must be added to a phosphate buffered solution (PBS, KH2PO4 and NaCl) and luminol (prepared with NaOH and diluted in PBS). Then chemiluminescence is photochemically induced with the compound under study and in the presence of reducing power NADPH. Finally, the chemiluminescence generated is measured in a Luminoskan luminometer with a microtiter plate [20].
There are other methods for determining other types of oxygen-reactive species, such as the superoxide anion (-O2), which is known as the NBT (NitroblueTetrazolium) Assay. In this method, the formation of -O2 is studied by monitoring the photosensitized reduction of nitro blue tetrazolium (NBT). This chemical process, which begins with the generation of -O2 through an electronic transfer between a photosensitizer and molecular oxygen, leads to the formation of a blue dye, called diformazan, which can be detected in the spectrophotometer at a wavelength of 560 nm. There it can be seen that the formation of a cationic intermediate (NBT+) is necessarily required for the production of diformazan. Thus, when NBT is irradiated in air-saturated solution in the absence of a photosensitizer, no diformazan is produced. In contrast, in the presence of a photosensitizer, the photoreduction of NBT towards diformazan is evident at 560 nm [27, 28].
It is noteworthy that some phthalocyanines could react and cause DNA cleavage, because interaction with light and ROS generation. These effects could be tested in water soluble zinc (II) phthalocyaninesby using supercoiled pBR322 plasmid DNA on agarose gel electrophoresis. It should be pointed out that phthalocyanines have DNA cleavage properties both without irradiation and under light. As a result, it was noted that the supercoiled form (form I) was transformed into nicked and linear forms (form II and III, respectively) (figure 10). Furthermore, it has been demonstrated that soluble zinc (II) phthalocyanines have significant photocleavage supercoiled pBR322 plasmid DNA properties under irradiation. All of these effects could be caused by oxidative effects mainly because it was showed that soluble zinc (II) phthalocyanines are able to produce big amounts of hydrogen peroxide (H2O2) [29].
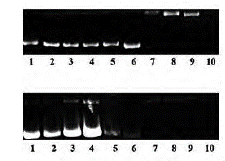
Figure 10 Pbr322 plasmid DNA cleavage mediated by water-soluble zinc (II) phthalocyanines on gel electrophoresis [29].
Additionally, from a spectrometric point of view, phthalocyanines are capable to bind DNA structure. The latter has been probed by carrying out UV-VIS spectrometry assay, where spectral data have shown that soluble zinc (II) phthalocyanines have binding calf-thymus DNA potential [29]. All these results are discussed on the basis of electrophoresis studies. It will serve us to recognize phototoxic effects and relate them with singlet and free radicals' generation, which are able to cause damage to DNA strands. We expect that depending on the metalized-phthalocyanine compound, the possible phototoxic effects into the PBR322 plasmid will be different.
RESULTS AND DISCUSSIONS
The expected results should be similar to those reported by Wadzanai et al. [30]. After carrying out the synthesis of the phthalocyanines, the spectrophotometric identification should be something similar to what is shown in the absorption spectrum of figure 11, where the Q bands for the phthalocyanines of Zinc, Cadmiun and Mercury are 672 nm, 679 nm and 684 nm, respectively.
Likewise, the data on the molar extinction coefficient and the fluorescent emission can provide relevant information for the identification of the compounds. The absorption Q band λmax (Log ε) for ZnPc is 672 nm (5.38), CdPc 679 nm (5.36), HgPc 684 nm (5.25). The fluorescence value λmax (emission/excitation) for Zn-Pc are 682 nm/672 nm, 691 nm/679 nm for CdPc and 698 nm/670 nm for HgPc in DMSO. However, it should be noted that these values depend on the solvent used (DMF, CHCl3, THF for example) [30].
Other photochemical data of interest concern the generation of singlet oxygen and other free radicals. Commonly, 1O2 generation is measured and expressed by its quantum yield (ФΔ), which is referred to the number of 1O2 molecules formed by each absorbed photon. In this sense, the quantum yield measured in DMSO has been found to be higher for HgPc (0.82) with respect to CdPc (0.78) and ZnPc (0.67). Again, it should be highlighted that these results will always depend on the solvent used during the measurements of these photophysical patterns [30].
Thus, considering the differences of absorption spectrum, it should be understood that the shift towards longer wavelengths is due to the increase in the size of the central metal atom. This latter causes an increase in the electron density. In this sense, regarding that mercury has more electrons in its atomic structure (and therefore, it is more reducing), it has the ability to destabilize the HOMO orbital with greater force, even more than cadmium and zinc. Due to this, a shorter "separation" is generated between the HOMO and LUMO orbitals that will provoke the absorption spectrum to be shifted towards the infrared. In addition, it should be pointed out that the differences in the Q bands shown in the different solvents are mainly due to the refractive indices.
The variations related to the molar extinction coefficients (ε) are due to the sizes of the central metals, where the highest value being for the ZnPc and the lowest for HgPc. These results can be explained because of the phenomenon of spin-orbit coupling, which becomes more evident as the size of the central metal ion increases. As a consequence of the latter, the nature of the excited singlet state initially pure will have some excited triplet character. Therefore, the transition S0 --- S1 will become somewhat "forbidden" [30].Additionally, the spin-orbit coupling effect also disturbs fluorescence processes. As a general rule, the phenomenon of spin-orbit coupling tends to favor the generation of molecules in the excited triplet state (c.a. the intersystem crossing is increased). This is a fact that is favored by the increase in the size of the central metal ion of phthalocyanine. As a result, it is expected that the fluorescence values, specifically of their quantum yields and lifetimes, will be higher for ZnPc and lower for HgPc.
Mercury is a heavier and larger atom than zinc and cadmium atoms. Therefore, the spin-orbit coupling process is much greater for HgPc. The probability of intersystem crossing with forbidden spin (Singlet-Triplet) is increased and, as a result, there will be an increase of the species in the triplet state for HgPc. Thus, all these factors together are what will make the fluorescence and its lifetime shorter for HgPc than for ZnPc and CdPc.
Keeping these aspects in mind, it can be said that probably HgPc will show stronger photosensitizing and phototoxic properties than ZnPc and CdPc. However, studies carried out establish that in a higher order ZnPc is considerably more efficient than many other metalized complexes tested [30-33]. The in vitro study of the different metalized phthalocyanines in plasmid PBR322 will give us a good indication about those properties and the effectiveness that these compounds could have as drugs to be utilized in photodynamic therapy (PDT) as well as their phototoxic character on DNA.
Likewise, several other works can be found on the use of phthalocyanines with metallic centers used in cancer cells, postulating different mechanisms of action, such as the action of oxidative stress on these cells or photo-induced apoptosis. Similarly, on the antitumor character that they may have. For example, photoactivation of ZnPc-loaded cells revealed strong phototoxic effects, leading to a dose-dependent decrease of cancer cell proliferation of up to almost 100%, the induction of apoptosis of the cell cycle, which was associated with decrease in cyclin D1 expression. By contrast, ZnPc-treatment without illumination did not induce any cytotoxicity, apoptosis, cell cycle arrest or decreased cell growth [34-36].