INTRODUCTION
During the last three decades, coffee (Coffea spp.) production has increased steadily; it went from 93 million 60.0kg bags in 1990 to 165 million bags in 2019. Although coffee is grown today in about 60 countries, 95% of the total volume is produced in 16 of them and 62% in only three: Brazil (35%), Vietnam (18%), and Colombia (9%) (International Coffee Organization, 2021).
There are two ways to satisfy the growing demand for coffee beans; one through incorporating new areas of agricultural vocation, which are becoming increasingly smaller, and the other; through increased productivity on lands currently cultivated (Sadeghian, 2014). According to the above, the production goals proposed in the medium and long term depend on the efficient and effective management of nutrients to achieve the expected economic, social, and environmental benefits (Fixen et al., 2015).
The continued extraction of nutrients by the yield, without the respective return, leads to the depletion of soil reserves and the subsequent reduction in production; conversely, the excessive use of fertilizers and unbalanced nutrition also generates contamination with possible yield consequences (Moody & Bruulsema, 2020). In this sense, nitrogen (N) constitutes one of the two macronutrients with the highest demand and influence on crop production, among which coffee is no exception; hence, it is included in fertilization plans at relatively high rates (Raij et al., 1996; Guimarães et al., 1999; Sanzonowicz et al., 2000; Sadeghian, 2013). Despite the above, more than half of the applied N can be lost in the environment (Lassaletta et al., 2014), wasting the resource, increasing production costs and causing threats to the air, water, soil, and biodiversity, and emitting greenhouse gases, a situation that has increasingly questioned their use in recent years (Dobermann, 2005; Lassaletta et al., 2014; World Meteorological Organization, 2019). The analysis by Lassaletta et al. (2014), based on the information collected by FAO during the last 50 years, revealed, for example, that in Greece, France, and the Netherlands, the nitrogen use efficiency (NUE) has increased in terms of yield of crops and total N inputs. While in India, Egypt, and China, increased fertilization has produced few agronomic benefits and significant environmental losses.
At the same time, large amounts of fertile soil rich in organic carbon (OC) and N are constantly being lost, generating more greenhouse gases, mainly CO2, CH4, and N2O (Lal, 2020), as a consequence of erosion and inappropriate management practices, a situation that affects root growth and increases the need to apply more N to sustain or improve yield. The described panorama turns on the alarms to conserve the soil and seek strategies that contribute to the efficient use of N. At the same time, alternatives are evaluated to increase yield and reduce environmental impacts (Batabyal, 2017). These strategies must integrate nitrogen fertilization with cultivars that are more efficient in their use and other cultural practices within good management practices (Sarkar & Baishya, 2017).
This article reviews the factors that determine NUE in coffee: plant, soil, climate, and management.
Definitions of nutrient use efficiency and NUE. To refer to NUE, it is convenient to previously define the concept of nutrient use efficiency (NUTUE), which is one of the leading indicators of mineral nutrition concerning the processes by which plants acquire, transport, store, and use nutrients to produce biomass when low or high rates of nutrients are applied through fertilization (Mathur & Goel, 2017).
There are different definitions of NUTUE; however, most authors agree on referring to the yield or nutrient absorbed/removed per unit of nutrient or fertilizer applied (Bruulsema et al., 2008), a statement comprising two components: nutrient absorption efficiency (the plant's ability to extract nutrients from the soil) and utilization efficiency (the ability to use nutrients to produce biomass). The evaluation of the NUTUE is helpful in the differentiation of soils for their ability to supply nutrients to plants and differentiate species and cultivars by their ability to absorb and use nutrients to produce maximum yields (Sarkar & Baishya, 2017).
In agronomic research, the following indexes are commonly used to evaluate the efficiency of the applied nutrients: partial factor productivity, partial nutrient balance, agronomic efficiency, apparent nutrient recovery efficiency, internal utilization efficiency, and physiological efficiency (Table 1). It is essential to consider that the NUTUE changes in space and time; therefore, the indicators make more sense when obtained for particular conditions of the cultivation system, soil, climate, and management contexts (Sarkar & Baishya, 2017).
Table 1 Summary of commonly used concepts of nutrient use efficiency.
Definition | Formula |
---|---|
Partial factor productivity (PFP) is units of crop yield per unit of applied nutrient. | PFP = YF/F or PFP = (Y0/F) + AE |
Partial nutrient balance (PNB) is units of nutrient uptake per unit of applied nutrient. | PNB = UF/F |
Agronomic efficiency (AE) of the applied nutrient is units of crop yield increase per unit of applied nutrient. | AE = (YF - Y0)/F or AE = ANR × PE |
Apparent nutrient recovery efficiency (ANR) is an increase in units of nutrient uptake per unit of applied nutrient. | ANR = (UF - U0)/F |
Internal utilization efficiency (IE) of a nutrient is units of crop yield per unit of nutrient uptake. | IE = YF/UF |
Physiological efficiency (PE) of the applied nutrient is an increase in units of crop yield per unit increase in nutrient uptake from fertilizer. | PE = (YF - Y0)/(UF - U0) |
F, amount of nutrient applied (kg ha-1); YF and Y0, crop yield with and without applied nutrient (kg ha-1), respectively; UF and U0, total nutrient uptake in aboveground biomass at maturity (kg ha-1) in fertilized and unfertilized control plot, respectively. Original information from Cassman et al. (2002) and Dobermann (2007), summarized by Sarkar & Baishya (2017).
Based on the NUTUE concept, NUE can be defined as the yield obtained or N absorbed/removed per unit of N or nitrogen fertilizer applied. The two components of NUE would then be the efficiency or capacity of the plant to absorb N from the soil and the efficiency or ability to use N to produce biomass (Moll et al., 1982; Hawkesford et al., 2012). In this regard, it is necessary to consider that a part of the N that enters the system is removed from the field in the form of harvested biomass. At the same time, the amount contained in crop residues is incorporated into the soil and recycled, making part of the organic matter (OM) and inorganic N reserves. However, another component, called reactive N, is not recovered and is lost from the system in inorganic reduced forms (e.g., NH3 and NH4 +), oxidized inorganic forms (e.g., NOx, HNO3, N2O, and NO3 -), and organic compounds (e.g., urea, amines, and proteins) (Cassman et al., 2002).
The EU Nitrogen Expert Panel (EUNEP, 2015) sustains that there is currently no uniform and robust methodology and protocol for estimating NUE. A more accessible indicator is then proposed, applicable to agriculture and production systems and food consumption based on the mass balance principle, using input and output data of N for its calculation: NUE = N output/N input. The NUE values must be interpreted as productivity (N output) and N surplus (the difference between N input and N output harvested).
It is possible to divide NUE studies into two groups: i) researches that generate indexes for one or more crops at specific sites for short periods (usually less than two years), using mainly primary information; for example, Fageria (2014) in cereals and bean and ii) comparative studies that use secondary information to evaluate regional changes (countries and continents) of some indices over more extended periods (more than ten years); for example, the reports for cereals and soybean of Cassman et al. (2002), Dobermann & Cassman (2002), Lassaletta et al. (2014), EUNEP (2015) and Fixen et al. (2015).
Determinants of NUE in coffee crops. N is one of the most required nutrients by the coffee crop (Martinez et al., 2020; Cenicafé, 2020), and, in many occasions, N is the most limiting yield (Sadeghian, 2008; Sadeghian, 2009). Fertilizers that contain N that contribute significantly to crop yield (Fageria, 2014). However, a high percentage of the quantity of N supplied is lost (more than 40%) (Prezotti et al., 2000; Bote et al., 2018b; Bess, 2020), which generates environmental pollution, greenhouse gases, and economic losses; therefore, adopting practices to increase NUE becomes a priority. This objective is not easy to achieve because the N cycle is complex. N is easily lost from agriculture to the environment (EUNEP, 2015).
The same factors that determine crop productivity also influence NUTUE and, therefore, NUE: plant, soil, climate, and management.
Plant. Having efficient cultivars in the use of nutrients contributes to improving the profitability of agricultural activity and reduces the impact on the environment. There are different definitions for an efficient plant in using nutrients, as discussed by Fageria (2014). Soil Science Society of America (2008) defines a nutrient-efficient plant as: "A plant that absorbs, translocates, or uses more of a specific nutrient than another plant under relatively low nutrient availability conditions in the soil or growing media." Regarding N, Graham (1984) refers to the ability of a genotype to produce higher yields under conditions of low nutrient content in the soil compared to other genotypes. The traits that distinguish the efficient species/cultivars in N absorption are related to the morphology of the root and the activity of the transporter; in turn, NUE is a consequence of the metabolic processes that favor the plant's capacity to assimilate and remobilize N towards the organs of interest (Masclaux-Daubresse et al., 2010); in the case of coffee, the bean contained in the fruits.
According to Engels et al. (2012), biomass partition between plant organs and their control mechanisms are crucial in crop production. The partitioning of N refers to the differential distribution of N among different sinks (Bhatla & Lal, 2018; Taiz et al., 2021).
Coffee species and varieties differ in the uptake/absorption and partitioning of N; therefore, genetic variability in NUE is also expected, which can be helpful in crop breeding programs (Cardoso, 2010). For Arabica coffee (Coffea arabica L.), Malavolta et al. (2002), Cardoso (2010), Amaral et al. (2011a), Amaral et al. (2011b), Martins et al. (2015), and Martinez et al. (2020) report differences in NUE between cultivars for Brazilian conditions. Similar results have been obtained for the Conilon coffee clones (Coffea canephora Pierre ex Froehner) by Prezotti & Bragança (2013), Colodetti et al. (2014), and Machado et al. (2016).
Martins et al. (2015) affirm the existence of genetic variability in coffee genotypes of the same species in terms of the absorption and nutrient use efficiency; nevertheless, they consider it necessary to better understand the absorption and utilization mechanisms of nutrients, as well as nature and genetic inheritance concerning efficiency. For these authors, Conilon coffee has a high NUE, which is suggested for selecting effective and responsive genotype indexes. For colombian conditions such differences have not been corroborated, as revealed by the research results carried out with C. arabica L. variety Castillo® under field conditions for 270 days after sowing (López, 2009), probably as a consequence of the small genetic amplitude among the evaluated accessions.
One of the difficulties in the selection of N-efficient genotypes in coffee is related to the relatively long cycle of the crop since the studies on perennial plants require more time to reach conclusive results, in contrast to the ones conducted on corn, wheat and rice. Researchers try to overcome this obstacle by evaluating materials in the seedling state, either using soil (Colodetti et al., 2014; Machado et al., 2016) or nutritive solutions (Cardoso, 2010; Martinez et al., 2020) in the field for two years Amaral et al., (2011a), Amaral et al., (2011b). It is not the most desired procedure, because neither does it represent crop conditions nor the variations in yield exhibited by the plantations between one year to the next. N use efficient genotypes are not yet an option disseminated by researchers and adopted by coffee growers for the reasons above.
Improving NUE is a biological, agronomic, and environmental problem. Advances in understanding the functional biology of N response and NUE have revealed how N source and N concentration have a genome-wide and plant-wide impact, involving genes from multiple biological processes (Sinha et al., 2020).
Several NUE definitions exist, but N uptake and utilization efficiency are the most relevant biological challenge (Mandal et al., 2018). Identifying proper metabolic pathways to genetically improve the NUE of crops is hampered by incomplete characterization of plant phenotype and genotype. (Mandal et al., 2018). Genetic improvement of NUE is often attempted within high-yielding varieties rather than searching for it within the genetic diversity of available germplasm (Mandal et al., 2018; Sinha et al., 2020).
Most of the current varieties accumulate important agronomical traits selected on fertile soils. The allelic diversity for the NUE traits would be shallow and even lack such alleles on nitrogen-limited soils (Sinha et al., 2020). Above is the case for the evaluation of C. arabica genotypes for NUE. Research carried out with this species has been characterized by the use of genotypes obtained in genetic improvement programs aimed at developing coffee varieties with high productive yields in environments that are not deficient in N (López, 2009; Martins et al., 2015). Therefore, it is crucial to identify contrasting genotypes or varieties so that germplasm can be ranked in increasing or decreasing order of NUE and thus make genetic associations using association genotyping techniques (Li et al., 2017). Molecular markers for selection/improvement can also be developed using conventional germplasm screening or mining available genomic sequences (Sinha et al., 2020).
N is mainly taken up through the roots and transported throughout the plant via the xylem. N compounds are also recycled and remobilized from internal stores or senescent tissues through the phloem to demand sites for bean filling, as is the coffee case (Filho & Malavolta, 2003). Genes involved in N uptake, assimilation, and remobilization processes are necessary to determine NUE, making NUE a complex quantitative trait (Mandal et al., 2018).
On a whole-plant scale, the response to N encompasses other genes and biological processes that contribute to NUE, including its regulation of carbon metabolism, redox metabolism, and root architecture. This is how genes involved in N sensing and upstream processes, including epigenetic regulation involving miRNAs, have been identified (Li et al., 2017).
Another major biological challenge for the genetic improvement of NUE is that neither the phenotypic traits nor the genetic alleles that determine it are clearly defined (Li et al., 2017; Mandal et al., 2018). Therefore, there is a great need to use chemical compound analogs from different N sources to carry out phenotypic screening for NUE-related genes/alleles (Mandal et al., 2018).
Soil. The ability of the soil to supply N to plants and NUE depends on its physical, chemical, and biological properties, mainly OM, flora and fauna, pH, texture, topography, aeration, moisture, temperature, and cation exchange capacity/effective cation exchange capacity (CEC/ECEC). These intervene in N cycle processes (fixation, mineralization, immobilization, nitrification, denitrification, leaching, volatilization, absorption, and removal by yield) and, therefore, affect its use efficiency (Havlin et al., 2017). There are countless reports in this regard, of which this item addresses some fundamental aspects.
In Figure 1, a conceptual model is presented, proposed by Balasubramanian et al., (2004). This describes the three main components (demand, supply, and losses) that control the recovery efficiency of N applied in fertilization variables and key processes.
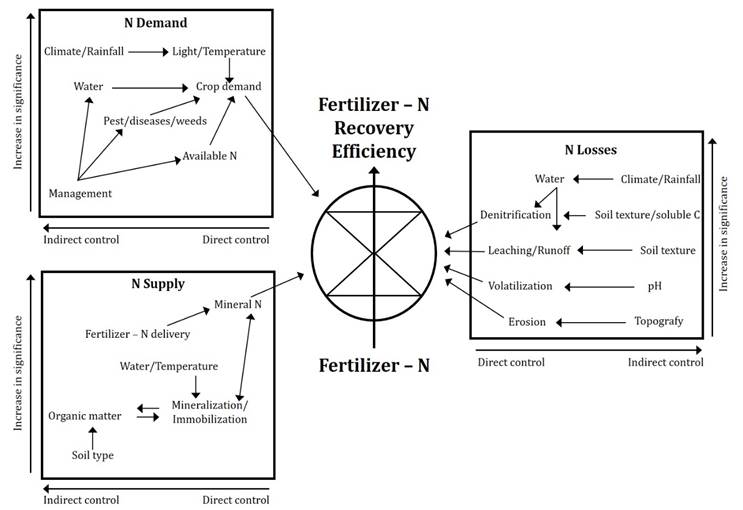
Figure 1 Conceptual model representing the three main control boxes (e. g., nitrogen demand, supply, and losses) and their effective processes and variables regulating fertilizer N use efficiency (NUE) (Balasubramanian et al., 2004).
OM, particularly the stable fraction (humus), is the primary N source for plants (Pilbeam, 2015; Raij, 2011), including coffee (Sadeghian, 2011); therefore, the response of crops to N supply and NUE depends on the mineralization of OM and crop residues. Through the constant growth of the roots and the entry of plant remains and other organisms into the system, residues are permanently incorporated into the soil which replenishes the OM contents, avoiding the depletion of this resource due to mineralization over time (Weil & Brady, 2017). According to Cardona & Sadeghian (2005) reports, more than 4.0Mg ha-1 of organic material annually enter the unshaded coffee monoculture and 8.0Mg ha-1 in shaded plantations of Inga spp., amounts that come to contribute to the system 100 and 200kg ha-1 of N, respectively. Recent reports suggest that the biomass of the vegetative organs of a variety Castillo® coffee crops in Colombia represent 58.0Mg ha-1 at 60 months after sowing, reaching 855kg ha-1 of N (Cenicafé, 2020). On the other hand, the loss of the superficial soil horizon due to water erosion reduces the OM, altering other properties such as bulk density, structural stability, and moisture retention, among others, which can minimize coffee productivity by up to 51.0% (Hincapié & Salazar, 2011).
Soil OM levels can be very contrasting in coffee-producing countries and regions, related to the origin of the soils and environmental conditions. In this regard, it is enough to mention the contents of less than 3.0% in Oxisols from Brazil compared to Andisols from Colombia with 20.0% or more (Sadeghian, 2011).
Due to the activity of heterotrophic organisms in the soil, a fraction of N contained in humus becomes available to plants by mineralizing OM. The magnitude and variations of these N contributions depend on the quantity and quality of OM and organic residues and the environmental factors that affect this process: temperature, moisture, aeration, and pH (Havlin et al., 2017). As an indicator of mineralization, microbial activity may vary between sites over time and, eventually, be higher in unshaded plantations than in shaded ones due to their higher temperature (Cardona and Sadeghian, 2005). This activity can intensify when the pH increases by liming, increasing nitrate levels resulting from more significant mineralization of the soil OM (Sadeghian & Díaz-Marín, 2020). According to Cannavo et al. (2013), the amount of mineralized N in the first 10.0cm of the profile of an Andisol represents up to 200kg ha-1 year-1 of N in coffee plantations under shade, reaching a large part of the crop's requirements. In turn, Babbar & Zak (1994) corroborated that OM mineralization depends on the coffee production system and changes over time, influenced by periods of rain and drought. The results obtained by these authors for conditions in Costa Rica reveal amounts equivalent to 148kg ha-1 year-1 in coffee crops under shade and 110kg ha-1 year-1 in unshaded plantations. Measurements made in two localities in Colombia suggest quantities between 8.0 and 40.0kg ha-1 of N each month during times without water deficit (Cenicafé, 2007).
The fractions of N available for coffee (NO3 - and NH4 +) present considerable variations over time in response to climatic changes and management practices (Ochoa et al., 2003; Sadeghian, 2010), an aspect that leads to the use of OM content as the primary indicator of N levels. In soil analysis calibration research (Figure 2), it was possible to explain relative coffee yield as a function of OM for Colombian conditions (Sadeghian, 2011). The quadratic model suggests that when nitrogen fertilization is suspended for three consecutive years in soils with OM<4.0%, the yield is reduced by 80.0%, and, when the OM>18.0%, the product also decreases because of limitations in mineralization caused by a lower temperature of the sites. Since the highest relative yield value was less than 90.0%, it is possible to interpret that there is always a response to N's supply.
As a process that causes environmental pollution and reduces NUE, N leaching is mainly associated with a nitric fraction (NO3 -). Its magnitude increases in more sandy soils and lower CEC and OM contents (Gaines & Gaines, 1994). Hincapié & Henao (2008) and Fenilli et al. (2008) argue that under field conditions (Colombia and Brazil, respectively), less than 2.0% of the N applied is lost by leaching; in turn, Babbar & Zak (1995) report losses close to 8.0% in unshaded coffee plantations and 3.0% in plantations under shade for conditions of Costa Rica. Cannavo et al. (2013) found higher losses for shaded plantations with Inga densiflora Benth. (157.2kg ha-1 year-1 of N), of which 53.0% (83kg ha-1 year-1 of N) corresponded to applied N (250kg ha-1 year-1). In research carried out in Costa Rica, Tully et al. (2012) found no differences in N losses due to leaching between organic and conventional management; in contrast, N losses decreased linearly as the biomass of the shade trees increased, a result that suggests the ecosystem service of the trees to mitigate N leaching. The application of high N rates (400 and 800kg ha-1 year-1), added to the high rainfall, can contribute to more significant leaching of N (up to 13.0% of the applied fertilizer) in irrigated plantations (Bortolotto et al., 2012). It has been shown that there are differences in losses between the soils of the Colombian coffee zone (Figure 3), in particular, due to their clay mineralogy, OM level, and pH rather than soil texture (Arias et al., 2009). To fertilizer sources, more significant leaching has been recorded under laboratory conditions (90.0% of losses as NH4 +) when using ammonium sulfate (AS), followed by ammonium nitrate and urea (González & Sadeghian, 2012a) (Figure 4).
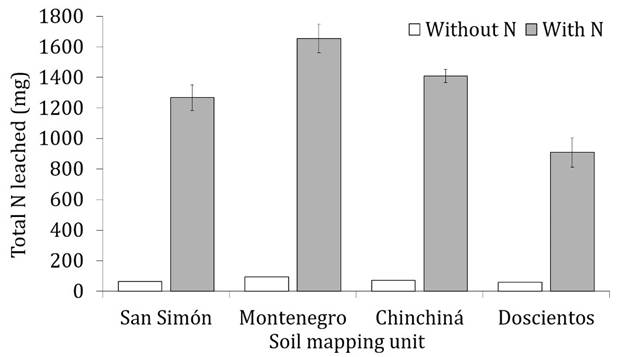
Figure 3 Nitrogen losses due to leaching, with and without N (urea) application in four soil-mapping units in Colombia (Arias et al., 2009). Soil mapping unit: Soil mapping units are defined by a group of soils with similar parental material and genetic horizons, with different weathering processes.
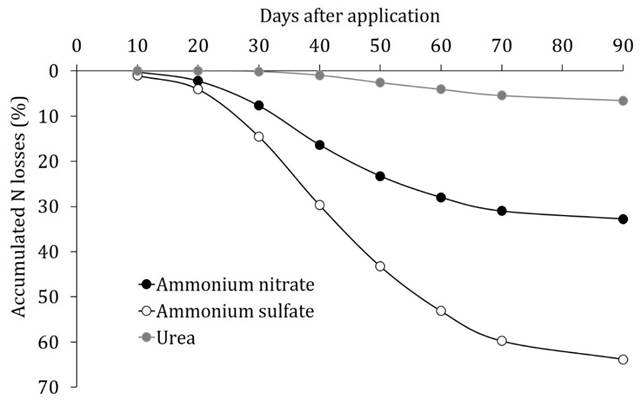
Figure 4 Nitrogen losses due to leaching overtime in an Andisol in Colombia due to the application of N sources (González & Sadeghian, 2012a).
The volatilization of N-NH3 is one of the most studied processes in recent years, an aspect that is more related to NUE than air pollution. Although volatilization occurs when applying any source of N, its magnitude is greater with urea. When this source is exposed to the soil surface, it hydrolyzes to form ammonium (NH4 +) by the action of the enzyme urease. Subsequently, ammonia (NH3) formation is favored, which is then released into the atmosphere, generating the loss of the element. These losses depend on the following soil properties: pH, CEC, OM, temperature, moisture, and buffer capacity (Dari et al., 2019). Shallow values (≤2.0%) are reported for coffee when ammonium sulfate (Fenilli et al., 2008; González & Sadeghian, 2012b) or ammonium nitrate (Dominghetti et al., 2016) are used, and losses between 15.5 and 43.2% with the use of urea (Leal et al., 2007; González & Sadeghian, 2012b; González et al., 2015; Chagas et al., 2016; Dominghetti et al., 2016). The N-NH3 losses are expected to increase with increasing pH, as occurs with the initial urea reaction; the opposite situation occurs when AS is applied, which tends to generate more acidification due to the nitrification of ammonia. For an Andisol from Colombia, González et al. (2015) detected increases of up to 0.5 pH units due to urea application.
Legumes' biological fixation of atmospheric N (N2) also contributes to N's balance in the system and NUE. The factors that influence the fixation of N2 are the supply of soil nutrients, pH, legume management, causes that affect photosynthesis, and fertilization with N (Havlin et al., 2017). For Mexican conditions, amounts set by Inga jinicuil between <1.0 and >40.0kg ha-1 year-1 are reported (Roskoski, 1982). Legume leaves are generally the primary sink for fixed N (Domenach, 1995); in this regard, 22.7kg ha-1 year-1 of N are reported for Inga densiflora (Cannavo et al., 2013), and values between 5.0 and 22.0% for Burundi conditions (Snoeck et al., 2000). The cover legumes that grow in the coffee plantations and protect the soil from erosion provide significant amounts of N, of which part comes from an atmospheric fixation; for example, in the biomass generated by Arachis pintoi, there are about 145kg ha-1 (Arango et al., 2009; Rose et al., 2019) and in Desmodium spp. 65.0kg ha-1 (Arango et al., 2009). In Brazil, A. pintoi in coffee crops was related to an increase in the soil microbial biomass and higher C and N levels, the preceding favored by the low C/N ratio of this coverage, the fast decomposition of its organic residues, and the immobilization of N by microorganisms; A. pintoi was also related to the more significant presence of edaphic fauna of the Isoptera order, linked to the high microbial activity in the soil (Lammel et al., 2015). Additionally, Rose et al. (2019) argue that the coverage of A. pintoi in coffee plantations can offset a part of the external contributions of N while reducing emissions of nitrous oxide (N2O).
N2O emitted into the atmosphere through nitrification and denitrification processes is about 300 times more effective than CO2 in catching heat in the atmosphere; however, the percentage contribution of this gas to global warming, due to the greenhouse effect, is low (IPCC, 2001). N2O formation occurs under anaerobic conditions, and its magnitude depends on drainage, NO3 - and NO2 - levels, pH, temperature, decomposable OM, quality and quantity of organic residues, nutrient reserves, and texture (Rosenstock et al., 2014; Farquharson, 2016). In most studies on coffee, N2O values less than 3.0kg ha-1 year-1 are reported (Babbar & Zak, 1995; Fenilli et al., 2008; Ortiz et al., 2018; Montenegro-Ballestero, 2019; Rose et al., 2019); nevertheless, in some, up to 12.0kg ha-1 year-1 have been detected (Hergoualc'h et al., 2012; Cannavo et al., 2013; Capa et al., 2015). Variations in N2O emissions, among other aspects, have been related to differences in soil N and CO levels (Rose et al., 2019), N rate (Montenegro-Ballestero, 2019), source of N (Montenegro, 2020), fertilizer placement (Rose et al., 2019), the water in the soil and rainy seasons (Hergoualc'h et al., 2007; Fenilli et al., 2008; Ortiz et al., 2018; Montenegro-Ballestero, 2019). Additionally, more denitrification and N2O emissions may occur in coffee agroforestry systems than in unshaded plantations (Babbar & Zak, 1995; Hergoualc'h et al., 2012). All this, due to a higher input of N through litterfall and a higher potential mineralization rate of OM in shaded crops. However, the net negative balance of the soil in agroforestry may be lower than in monoculture when the C accumulation in the biomass of the trees is considered (Hergoualc'h et al., 2012).
Climate. The interaction of the elements or components of the climate (temperature, precipitation, air humidity, solar radiation, cloud cover, atmospheric pressure, winds, evaporation, and evapotranspiration) affects the growth of coffee (Jaramillo, 2018) and many of the processes that ultimately govern the NUE (Fageria, 2014; Sarkar & Baishya, 2017). Rain and soil surface temperature (up to 25.0cm deep), which depends on the air temperature (Jaramillo, 2018), are the elements with the most significant influence on the processes that affect N availability. These processes refer to the activity of the microorganisms that mineralize OM, biological fixation, immobilization, nitrification, denitrification, leaching, erosion, volatilization, plant growth, and N absorption and partitioning (Balasubramanian et al., 2004; Weil & Brady, 2017).
The amount and distribution of rainfall show variations between sites and over time (inter and intra-annual), aspects that must be considered to define fertilization plans, especially regarding application time and the source of N (Sadeghian & Jaramillo, 2016; Sadeghian et al., 2017). Low rainfall is related to limitations in the volume of water containing available N (NO3 - and NH4 +) for coffee (Sadeghian et al., 2017), while excessive precipitation increases leaching losses (Sadeghian et al., 2015). The coffee regions differ considerably in their rainfall even in the same country, as shown in Figure 5 for four localities in the Colombian coffee zone. According to the locality, soil properties, and production system, soil temperature also changes. An example of coffee plantations in full sunlight and with the shade of Inga spp. is presented in Figure 6.
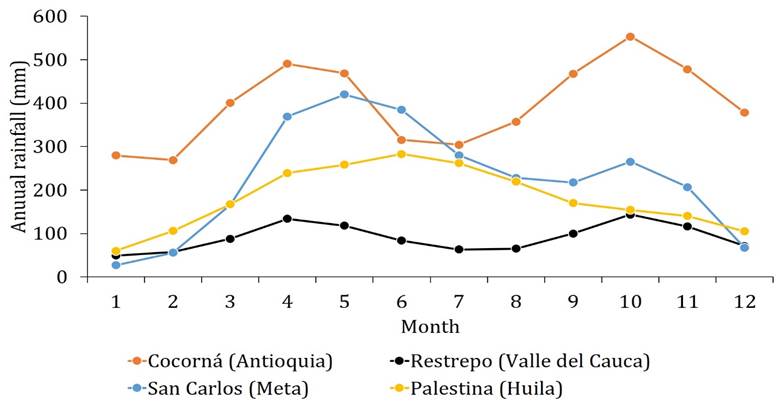
Figure 5 Annual variations in rainfall in four municipalities in the Colombian coffee zone. The parentheses denote the department. Original data from Cenicafé (2021).
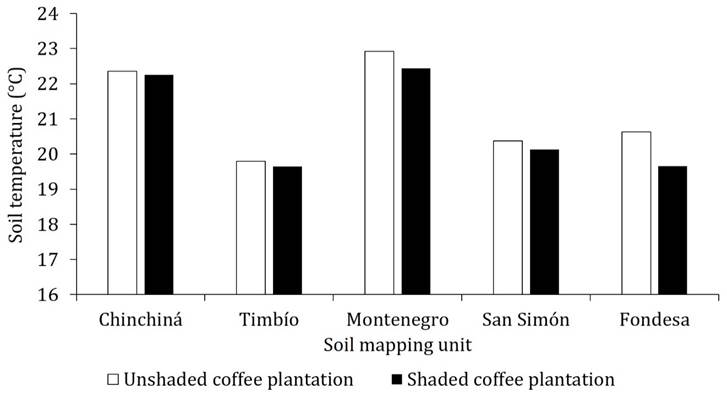
Figure 6 Soil temperature in coffee plantations unshaded and with the shade of Inga spp. in five Colombian soil-mapping units. Higher temperatures are recorded in unshaded coffee plantations than in shady plantations (Cardona & Sadeghian, 2005).
Solar radiation directly affects photosynthesis, which influences the plant's demand for nutrients (Fageria, 1998). In a study developed by Bote et al. (2018a), both solar radiation and N supply positively affected some of the attributes of the coffee plant, with positive and negative interactions between the two variables. Bote et al. (2018b) also recorded more use of N and higher biomass and production when increasing the rate of N for a higher level of radiation. In this case, N's recovery ranged between 7.0 and 17.0% and decreased with a higher supply of N and increased with the level of radiation. Therefore, it is essential to include the physiological traits of plants in crop breeding programs that improve their ability to tolerate multiple climatic stress factors (Sarkar & Baishya, 2017).
Management. Adopting appropriate soil, plant, climate, and fertilizer management practices improve NUE and favor maximum economic crop yield (Fageria & Baligar, 2005). Most of the environmental components are not controllable; instead, the management seeks to reduce the negative effect of some and take advantage of the benefits of others.
Practices to increase NUE should aim to reduce N losses in the system, improve crops' absorption and use and achieve high productivity (Degaspari et al., 2020). Some of these practices are directly related to the supply of the nutrient through fertilization, specifically, rate and source, time, place and fertilizer placement, and the balance of N with other nutrients; meanwhile, different practices have more indirect effects, for example, productive and N use efficient genotypes, erosion control, liming, use of organic fertilizers, association with legume species, management of pests, diseases and weeds, irrigation, planting densities and spatial arrangements, shade level, planting date and renovation systems. One or more of the above practices have been cited by Batabyal (2017), Fageria (1998), and Fageria & Baligar (2005).
Nitrogen rate. It is crucial to consider the difference between N management's efficiency and effectiveness. There are different ways to define the NUTUE and NUE; however, they generally resemble that the highest efficiency occurs when the fertilizer is applied at lower rates than the economically optimal rate (Dibb, 2000; Fixen & García, 2006), as illustrated in Figure 7. This last alternative may go against the economic sustainability of coffee farming, and, therefore, it would be necessary to define the priorities in the two management visions.
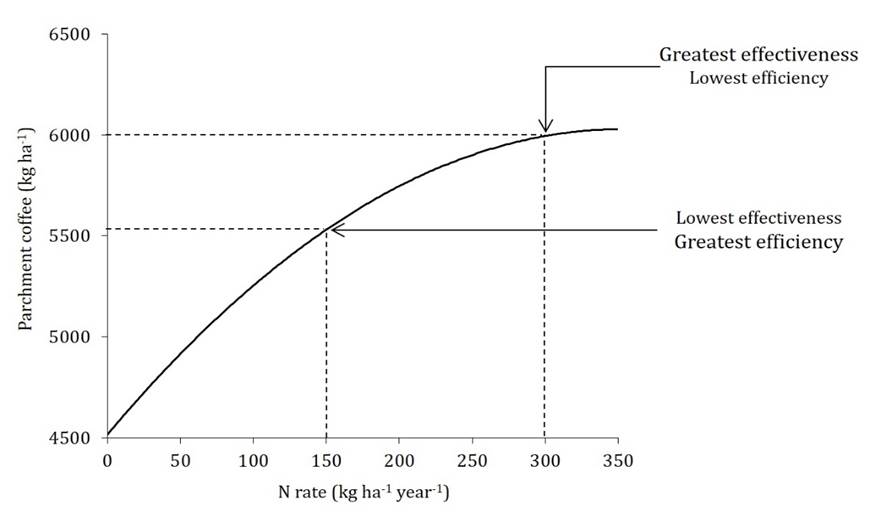
Figure 7 Efficiency vs. efficacy for the response of coffee to nitrogen supply. Adapted from Dibb (2000) and Fixen & García (2006).
There are differences between the recommended N rates and the criteria that support this practice. For Colombia, rates between 120 and 300kg ha-1 year-1 of N are recommended (Sadeghian, 2013), according to the OM content and the production system (affected by planting density and shade level), for Brazil from 50.0 to 450kg ha-1 year-1, according to the productivity and N foliar concentration (Raij et al., 1996) or based on the crop productivity according to the planting density (Guimarães et al., 1999). In this regard, it is necessary to bear in mind that excess N causes environmental losses and contamination, hence, the care required to select the appropriate rate based on the different soil and plant indicators. The use of SPAD-502 has been evaluated as an indirect measure to estimate foliar N through the chlorophyll reading, to generate indicators that allow adjusting the rates of N and increasing its efficiency. In this regard, while in some cases, the use of this instrument has been successful (Netto et al., 2005; Reis et al., 2009), in others, it has not (Rendón & Sadeghian, 2018), which is why it is currently not widely used, despite its comparative advantages.
Nitrogen source. In general, the research results on fertilizer sources do not reveal significant differences in coffee yield, as shown in Figure 8, which is an aspect that is commented on by Malavolta (1993); however, this does not necessarily imply that they cause similar effects on the soil, that their gains and losses are comparable, or that their compatibility in mixtures is the same. To select the appropriate source, it is necessary to jointly consider aspects such as the requirement of the crop, the result of the soil analysis, the interactions of the nutrients and their balance with N, the cost, the residually, and the availability, among others. Currently, many professionals and companies condemn the use of urea due to its volatilization, frequently ignoring that nitric sources (for example, calcium nitrate, magnesium nitrate, and ammonium nitrate) present more losses due to leaching. Another aspect is the residual acidity, in which urea and ammonium nitrate are comparable. At the same time, AS generates more acidity and can be used more frequently in soils with high pH for coffee (Sadeghian & Duque, 2019).
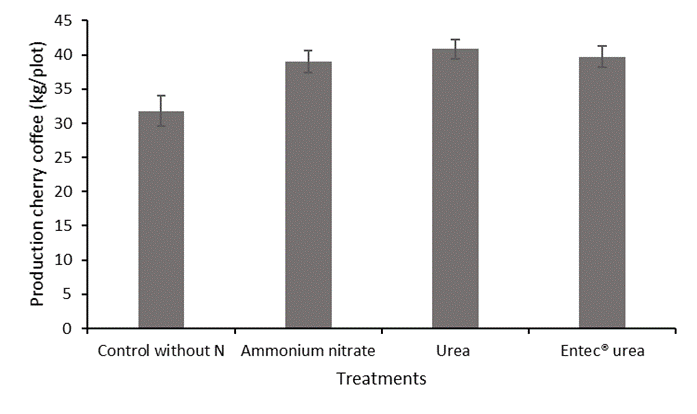
Figure 8 Cherry coffee yields in response to three nitrogen sources in an Andisol fromColombia (Sadeghian, 2022). Average values of three rates of each source (100, 200 and 400kg ha -1 year -1 ). ENTEC® (DMPP (3.4-dimethypyrazole phosphate, is a nitrification inhibitor).
Application time. In order to increase NUE, it is necessary to apply fertilizers at times of most significant demand for the crop and adequate soil moisture conditions for two or three consecutive months (Malavolta, 1993; Sadeghian & Jaramillo, 2016; Sadeghian et al., 2017). Malavolta (1993) and Favarin et al. (2010) are based on the demand for N by the coffee fruit to define the fertilization seasons; in this regard, Sadeghian (2013) draws attention to the issue and clarifies that the condition of soil moisture, governed mainly by rain, prevails over the times of most significant accumulation of N in the fruit if the soil is dry. Furthermore, the requirements of the other organs of the plant in different periods of fruit growth should not be forgotten. In this regard, Fenilli et al. (2008) consider that to estimate the efficiency of the recovery of supplied N, it is necessary to take into account the content of N accumulated in other organs of the plant, in addition to the harvested fruits; consequently, 19.1% are reported in the aerial part of the plant, 9.4% in the roots, 23.8% in the litter and 26.3% in the fruits.
Application of nitrogen fertilizers when the soil remains dry can increase soil salinity and cause toxicity in plants, particularly during the establishment stage (Sadeghian et al., 2017). Taking into account the above considerations and based on soil properties, it is generally suggested to supply N and other nutrients two or three times during the year (Cervellini et al., 1986; Martinez et al., 2020; González-Osorio & Sadeghian, 2020); however, when it comes from fertigation, up to 12 applications per year are suggested (Sobreira et al., 2011).
One of the practices to reduce annual fluctuations in coffee production (biennially) has been the fractionation of nitrogen fertilization. Despite the theoretical bases, the results obtained do not reveal any effect (Sanzonowicz et al., 2000).
Nitrogen placement. It is suggested to place the fertilizer where the roots are, bearing in mind both the horizontal and vertical distribution (Malavolta, 1993; Martinez et al., 2020), which varies with plant growth and renewal systems (Sadeghian, 2013). Since the predominant fraction of N in the soil (NO3 -) is very mobile, it easily migrates in the profile after its application, so it is suggested to apply it in a dispersed way when fertilizers solids are treated (excludes drip or fluid fertilization).
CONCLUSIONS
The factors that determine NUE are the same that define coffee crop productivity, namely, plant, soil, climate, and management. Despite in recent years, significant advances have been made to search for genetic targets to improve NUE plants, it may be a decade or more before improved EUN coffee varieties are available or used in world coffee farming.
The soil's physical, chemical, and biological properties and climate elements, mainly temperature, precipitation, and solar radiation, affect coffee growth and many of the processes that ultimately intervene in the functions of the N cycle and affect NUE; nevertheless, some components of these two factors are not fully controllable.
Management of factors that determine NUE should reduce N losses in the system, increase its absorption and use by coffee plants, and maintain or increase yield.