INTRODUCTION
In 2021, Colombia's citrus production reached 1,450,071 tonnes, with Tahiti lime accounting for 26% (MADR- Ministerio de Agricultura y Desarrollo Rural, 2021). By 2022, Tahiti limes had risen to become the third most exported fruit after bananas and Hass avocados, witnessing a 90% surge in international sales between January and April compared to the same period in 2021 (Procolombia, 2022). These figures underscore the economic significance of Tahiti lime in contemporary Colombia and the promising opportunities they present for producers and traders eyeing the export market. In light of this, it is crucial to identify regions with potential for this species' cultivation. It is noteworthy that some orchards situated at higher altitudes might demonstrate different behaviors compared to those in warm and temperate areas, with possible implications for the fruit's organoleptic quality (Naryal et al., 2020; Fischer et al., 2022).
Citrus predominantly thrives in various tropical humid, tropical dry, and Mediterranean climatic conditions (Goldschmidt & Koch, 2017; Luro et al., 2020). Under subtropical conditions, temperature and water availability pose the most significant environmental factors impacting citrus water relations (Dutta et al., 2022). In the tropical climate zone, the vigor displayed by citrus is a drawback for orchard development, restricting yield expansion in the cultivated area (Mademba-Sy et al., 2012). Sour lime, being highly sensitive to cold (Barry et al., 2020), sees its cultivation limited to the world's warmer citrus belts. As a case in point, small-fruited sour limes, such as Mexican limes, are cultivated near the equator (Khan et al., 2017). In colder regions, trees tend to extend their phenology. If the fruits remain on the tree for extended periods, rising sugar levels and falling acidity could result in bland fruit (García-Muñoz et al., 2021).
In the main producing regions of the Tahiti lime subtropics, the annual cycles of plant growth and development are regulated by the climatic modifications presented by the succession of the seasons (winter, spring, summer, and autumn). In these seasonal changes, increases in average temperature and radiation occur between winter and summer, decreasing subsequently (Dutta et al., 2022). What determines the growth and development behavior of citrus plants; as well as the quality characteristics (internal and external) of the fruits. On the other hand, the varieties and patterns used in the subtropics are the result of a long research process and the citrus fruits obtain excellent quality (Ríos-Rojas et al., 2020a). The production of these regions is oriented toward high-income markets and consumers (Ríos-Rojas et al., 2020b). The scientific information on citrus fruits has been generated for these geographical conditions and these markets, and it is not possible to extrapolate them to tropical conditions.
A thorough understanding of the ecophysiology of Tahiti limes in Andean tropics conditions is vital for crop management and for devising the necessary conditions to boost productivity (Maia et al., 2015). This knowledge also aids breeding programs in the selection of cultivars better suited to adverse environmental conditions (Silva et al., 2016). Therefore, this study seeks to document the floral phenology of Tahiti lime and examine the physical and chemical characteristics of the fruit in two contrasting environments in the Andean region of Caldas, Colombia.
MATERIALS AND METHODS
The study was conducted in two commercial orchards situated in varying altitudinal zones: Manizales at 2,280 m above sea level (5° 1’47’’N), characterized by a cold humid (CH) climate (Vélez-Upegui et al., 2015), and Santagueda at 1,010 m above sea level (5°4’30’’N), classified as temperate semi-humid (Tsh) (Vélez-Upegui et al., 2015). Both orchards are in the Department of Caldas, Colombia. We selected eight Tahiti limes trees, aged between 8 and 10 years, from each orchard and marked reproductive buds and fruits on each tree. The soils of both orchards possess a loamy texture (USDA - United States Department of Agriculture, 2023), and the agricultural management adhered to the guidelines of the ICA (Colombian Agricultural Institute) in resolutions 448 and 30021 for export-oriented properties. The study spanned from August 2021 to July 2022.
The trees chosen showed homogeneity in their growth and production metrics, being their distribution in the orchard random, given the flat topography of both zones. In each tree, two reproductive buds per quadrant were marked, the floral phenology was monitored every 15 days, and the fruits were retained throughout the time until harvest. The inflorescences were marked with plastic strips, and we marked the fruits with tweezers at their marbled size stage. The phenological scale considered in the study was the BBCH for the citrus genus.
To record climatic variables in each area, we used conventional meteorological stations operated by the Institute of Hydrology, Meteorology, and Environmental Studies (IDEAM), which logged daily air temperature, precipitation, and sunshine values throughout the study period. To calculate accumulated heat units (AHU), we considered the maximum and minimum temperatures recorded during the span from reproductive bud stage to flowering and from anthesis to harvest. We employed the following Equation 1 for the calculation:
Where Tb= 12.5 (Hernández et al. 2015)
For the physical and chemical analysis of the fruits, we collected 64 fruits at their harvested state from each orchard in 8-year-old trees. From pre-flowering to harvest in Manizales and Santagueda was 279 days and 150, respectively. We measured the physical variables including fruit weight using a digital balance, the polar and equatorial diameter and skin thickness using a manual caliper, as well as the fruit color using the color chart of the Colombian Technical Standard 4087 (Icontec - Instituto Colombiano de Normas Técnicas y Certificación, 1997a). The chemical variables measured included juice content expressed as a percentage, titratable acidity according to the protocol of the NTC 4623 of 1999 (Icontec, 1997b), ascorbic acid determined by the iodometric technique, and soluble solids content expressed in Brix degrees by the refractometric method (Icontec, 1999).
A completely randomized design (CRD) was applied, with each tree as the experimental unit and eight replications corresponding to the trees. We assessed the results through analysis of variance and Duncan's mean comparison (p<0.05). The statistical software utilized was S.A.S (SAS Inst, Cary N.C. Version 9.4).
RESULTS AND DISCUSSION
Flowering phenology. This study's findings underscore differences in flowering phenology between the two environments analyzed. Drawing on the primary stages of the BBCH phenological scale, we devised a scale using imagery. Observations suggest that trees in the semi-humid temperate zone (Santagueda) advanced to harvest stage '83' (denoting ripe fruit ready for harvest) in 150 days, starting from stage '51' (where buds are swollen, closed, and scales are visible). Conversely, trees in the cold humid zone (Manizales) achieved harvest at 279 days, beginning at the same developmental stage (Figure 1).
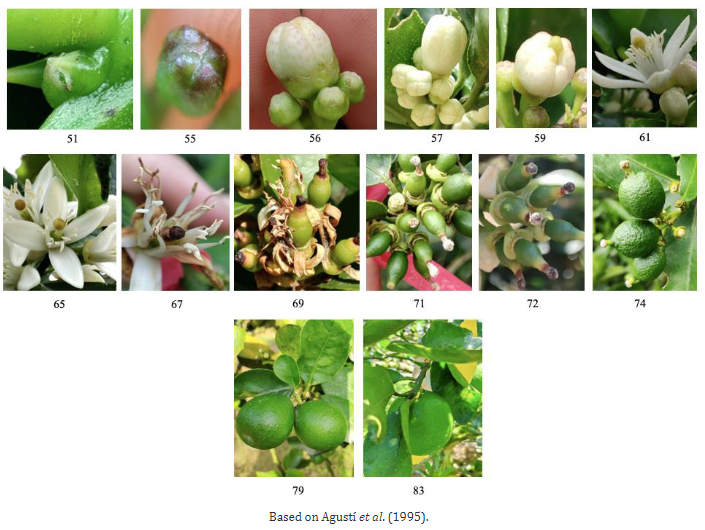
Figure 1 Flowering phenology of Tahiti acid lime in the Andean tropics of Caldas. Based on Agustí et al. (1995).
Description of different stages. 51. The buds swell: they are closed and the scales become visible, slightly green. 55. The flowers become visible: they are still closed (green button) and are distributed singly or in clusters in inflorescences with or without leaves. 56. The petals grow; the sepals envelop half of the corolla (white button). 57. The sepals open: the ends of the petals become visible, still closed, white or purple. 59. Most of the flowers, with the petals closed, acquire the shape of a hollow, elongated ball. 61. Beginning of flowering: about 10% of the flowers are open. 65. Full bloom: about 50% of flowers are open. First, petals begin to fall. 67. Flowers fading: most of the petals are falling off. 69. End of bloom: all petals have fallen. 71. Fruit set: the ovary begins to grow; the young fruits begin to fall. 72. The fruit, green, is surrounded by sepals in the form of a crown. 74. The fruit reaches about 40% of the final size. They acquire a dark green color. The physiological fruit drop is complete. 79. The fruit reaches about 90% of its final size. 83. The fruit is ripe for harvesting, although it has not yet acquired its characteristic color.
Given that environmental factors directly shape plant phenology (Seghieri et al., 2012; Tang et al., 2016; Piao et al., 2019; Harris et al., 2022) and that temperature is a dominant influence (Bahuguna & Jagadish, 2015; Zhang et al., 2022), the temperature differentials between the two zones in this study suggest a plausible reason why the lower-altitude zone reaches harvest 129 days earlier than its higher-altitude counterpart. The average temperatures recorded during the study were 23.6°C in the lower zone and 15.1°C in the upper zone.
In citrus, the estimated interval from anthesis to a harvestable fruit typically ranges between 4 and 10 months (Maia et al., 2015; Bettini-Tambur, 2022). However, this study indicated that the lower zone transitioned from flower to fruit in 129 days. This result aligns closely with the findings of De Souza et al. (2005), who reported durations of 139 and 148 days in Brazilian production areas, yet deviates from those of Milla et al. (2009). The latter researchers observed periods of 165 days from open flower to harvestable fruit in Venezuela and 96 days from open flower to harvest in Monagas, Venezuela, respectively. In the study's high-zone orchard, this period extended to 230 days, likely a result of the recorded temperature conditions; in areas where average temperatures fall below the tree's minimum requirements, metabolic processes could decelerate, leading to protracted phenological development.
This study also found that fruit development adhered to the sigmoid growth pattern from anthesis to ripening, as noted by Shakya et al. (2018). In this pattern, the transitional phase (69) to the harvest-ready fruit stage (83) was the most protracted and slowest period of fruit growth in both zones. According to Orduz & Mateus (2012), the climatic elements of importance for the cultivation of citrus fruits in tropical conditions are frequency and distribution of precipitation, followed by radiation, temperatures (total averages, maximum and minimum averages), relative humidity, and wind speed. Annual behavior and its variability condition the phenology of plants and the development of crop management practices such as irrigation, fertigation, fertilization, pruning, and phytosanitary management. The sum of these climatic variables, together with crop management practices, determines the productive behavior and quality of the fruits.
Accumulated heat units (AHU). The Accumulated Heat Units (AHU) recorded for the two study zones were strikingly different (Table 1). In the zone of lower altitude, we documented an accumulation of 1559.7 AHU from pre-flowering to harvest, while the higher altitude zone amassed 605.1 AHU. During the period between anthesis and harvest in the lower zone, 1309.8 AHU accumulated. These findings closely align with those reported by Bettini-Tambur et al. (2022) in Brazil, where they recorded 1226.5 AHU over 134 days for the same evaluation period. Similarly, Hernández et al. (2014) reported higher AHU values for a comparable period across four different locations in Colombia.
Table 1 Accumulated Heat Units (AHU) in flowering phenology in two contrasting zones of the Andean tropics of Caldas, Colombia.
Stages of development until flowering | Days elapsed | Accumulated heat units (AHU) | |||
---|---|---|---|---|---|
a Manizales | b Santagueda | a Manizales | b Santagueda | ||
Pre-flowering | 49 | 21 | 131.8 | 249.9 | |
Anthesis | 32 | 27 | 86.5 | 263.3 | |
Fructification | 198 | 102 | 386.7 | 1046.5 | |
Total | 279 | 150 | 605.1 | 1559.7 |
a2280 m asl - b1010 m asl. Manizales between October 2021 and July 2022; Santagueda between November 2021 and April 2022
The limited heat accumulation in the high zone can be understood when considering that plants thriving near sea levels tend to grow more slowly in higher-altitude regions (Fischer, 2022). This is because an increase in altitude is associated with reductions in temperature. Occasionally, elevated average temperatures can result in lower heat accumulation but an earlier phenological period (Bhattacharya, 2022), which contrasts with the observed conditions in the higher altitude zone of this study, where heat accumulation was minimal, yet the phenological period was extended. Regions with higher average temperatures (above 27°C) have greater accumulation of AHU and, therefore, greater respiration, which reduces the concentration of soluble solids and acidity of the fruit (Hernández et al., 2015). The localities present the following average annual AHU: Santagueda (1559.7) and Manizales (605.1). In Manizales, where AHU is low, fruits slowly lose their acidity. In Santagueda, the metabolic processes are more accelerated, there is a higher respiration rate, and the life of the fruits is relatively shorter.
Climatic behavior in the study areas. The climate in the two study zones demonstrated distinct differences in air temperature, precipitation, and sunlight. The maximum and minimum temperature values in the low-altitude zone (Figure 2) were more closely aligned with the optimal range for citrus production than the temperatures observed in the high-altitude zone. Optimal temperatures for citrus cultivation range from 21 to 34°C, while temperatures exceeding 37°C or dropping below 10°C can impede growth (Balfagón et al., 2022). Tahiti lime trees, particularly, are sensitive to both freezing temperatures and extreme heat (>45-50°C). The Tahiti lime variety exhibits greater cold hardiness than the Mexican lemon, capable of withstanding temperatures as low as -2°C (Khan et al., 2017).
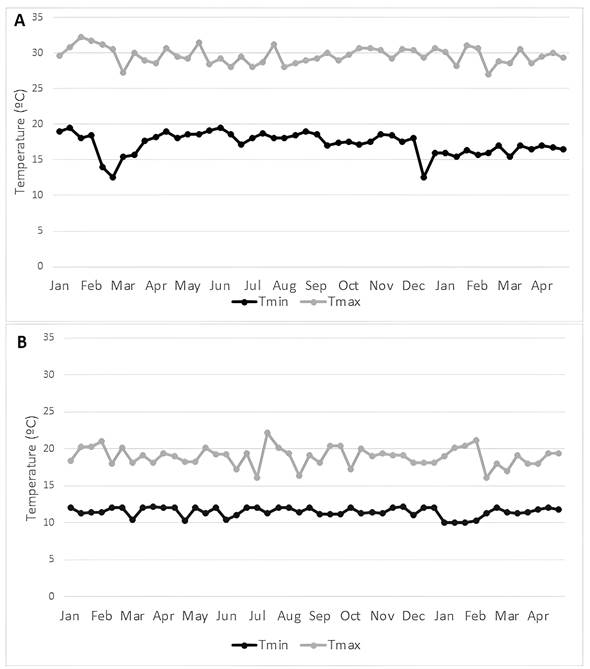
Figure 2 Maximum and minimum temperature in the period January 2021 and April 2022 in the study areas. A. Santagueda (1,010 m asl) B. Manizales (2,280 m asl).
It is worth highlighting that, in Colombia, this species is cultivated from sea level to 2,100 m asl (Hernández et al., 2015). For commercial purposes, it is recommended to do so up to 1,600 m asl (Hernández et al., 2014). On average, the citrus zone has temperatures between 18 and 30°C, appropriate for the development of the crop. According to Balfagón et al. (2022), the ideal temperature range for citrus fruits is between 12.5 and 39°C, with an optimal level of 23.4°C. Regarding precipitation, the country's rainfall regime determines one or two harvests per year (Ríos-Rojas et al., 2020a). In the case of the Tahiti lime, the aforementioned regime defines the flowering and the maximum levels in the production curve, given that it is harvested throughout the year.
Figure 3 shows that rainfall occurred throughout all months of the study period in both zones, although the lower altitude (Santagueda) zone recorded higher cumulative values than the upper zone (Manizales), with 4,417 mm and 2,799 mm, respectively (Figure 3). Although continuous rainfall is beneficial in regions without irrigation systems (Orduz & Mateus, 2012), and the ideal is to maintain the soil at field capacity (Rios-Rojas et al., 2020a), soil infiltration properties are crucial to avoid possible waterlogging caused by excess rainfall (Duan et al., 2020), which occurs in both zones during certain months of the year. On the other hand, it is also important to consider that some pre-harvest and post-harvest fruit damage may be greater during the rainy season (Romero, 2019), so maintaining a constant rainfall regime is not the most appropriate for this fruit tree.
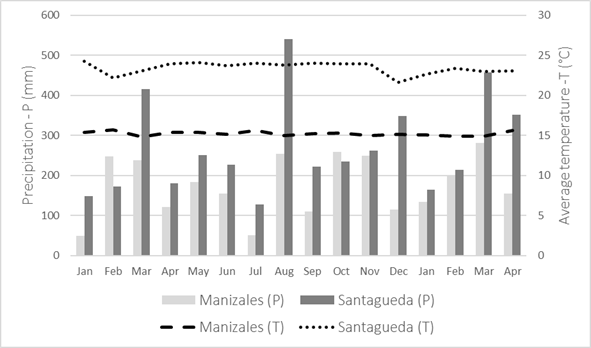
Figure 3 Precipitation and average temperature values in the period January 2021 to April 2022 in the study areas.
Sufficient sunlight in each zone is vital to meet the Tahiti lime tree's needs for effective photosynthesis. However, in regions with consistently clear skies and high radiation, a photoinhibition process can occur, which may harm the tree (Gullo et al., 2020). Under this criterion, both study zones are located in places where the constant presence of clouds reduces the risk of photoinhibition, although prolonged events of rain and high cloudiness can reduce the radiation balance (Silva et al., 2023). Nevertheless, the lower altitude zone presents higher insolation values (Figure 4), indicating better light availability for Tahiti lime tree growth (Ríos-Rojas et al., 2020a).
Physical and chemical characteristics of fruits
As can be observed in the summary table of the analysis of variance (Table 2), all the chemical variables measured showed statistical differences, while the physical variables, fruit weight, and equatorial diameter did not show statistical differences between the study zones.
Table 2 Analysis of variance for physical and chemical characteristics of Tahiti and lemon fruits in two contrasting environments in the Andean region.
Variable | Source | DF | Type III SS | Mean square | P-Value | Pr > F |
---|---|---|---|---|---|---|
Fruit Weight (FW) | Location | 1 | 111,33 | 111,33 | 0,19 | 0,66 |
Equatorial diameter (ED) | Location | 1 | 0,41 | 0,41 | 1,66 | 0,19 |
Polar Diameter (PD) | Location | 1 | 6,33 | 6,33 | 13,42 | 0,0004 |
Shell Thickness (ST) | Location | 1 | 0,18 | 0,18 | 22,95 | <,0001 |
Soluble Solids (SS) | Location | 1 | 35,40 | 35,40 | 36,12 | <,0001 |
Titratable acidity (TA) | Location | 1 | 355,27 | 355,27 | 787,03 | <,0001 |
Ascorbic acid (AA) | Location | 1 | 0,009 | 0,009 | 60,35 | <,0001 |
Juice yield (JY) | Location | 1 | 1711,83 | 1711,83 | 15,62 | 0,0001 |
Fruit weight at the two altitudes of 1010 m asl (Santagueda) and 2280 m asl (Manizales) displayed no statistically significant differences (p<0.6503), weighing 108.88 and 106.92 g, respectively (Table 3). According to the technical standard - NTC (Icontec, 1997a), these weights fall within the permitted ranges (60 to 165 g). The same was true for equatorial diameters, which were found to be 54.9 and 53.8 mm (p<0.1995) for the lower and higher altitude zones, respectively. The NTC states that the allowed ranges are between 49 and 60 mm. These results demonstrate that fruit weights in the two locations are higher than those reported by other exporting countries, such as Brazil (Silva et al., 2016) and El Salvador, which report weights of 78.8 g (Silva et al., 2023).
Table 3 Physical and chemical characteristics of Tahiti lemon fruits in two contrasting environments in the Andean region.
Site | Physical characteristics | |||||
---|---|---|---|---|---|---|
FW (g) | ED (mm) | PD (mm) | PD/ED | SM (g) | ST (mm) | |
Santagueda | 108.88 a | 54.90 a | 62.00 b | 1.21 | 75.42 | 2.30 b |
Manizales | 106.92 a | 53.80 a | 66.50 a | 1.15 | 80.80 | 3.10 a |
CV (%) | 22.6 | 33.95 | 10.69 | 32.8 | ||
Site | Chemical characteristics | |||||
SS | TA* | SS/TA | AA** | J (g) | JY (%) | |
Santagueda | 7.29 a | 6.18 a | 1.18 | 0.064 a | 33.46 a | 30.73 |
Manizales | 6.25 b | 2.84 b | 2.20 | 0.046 b | 26.12b | 24.43 |
CV (%) | 14.61 | 14.94 | 23.18 | 35.17 |
WF= fruit weight; ED= equatorial diameter; PD= polar diameter; PD/ED= allometric growth; SM= shell mass; ST= shell thickness; SS= soluble solids; TA= titratable acidity; SS/AT= consumption rate; AA= ascorbic acid; JY= juice yield. Means followed by the same letters are not significantly different at the 0.05% level of probability of error. * expressed as citric acid (g) in 100 g juice; and ** expressed as ascorbic acid (mg) 100 g-1 fresh weight of juice.
The results obtained showed that the juice weight for the lower-altitude of “Santagueda” zone was 33.46 g with a juice yield of 30.73%, complying with the technical standard that establishes a minimum value of 27% juice content (NTC). Conversely, in the higher-altitude zone “Manizales”, the juice weight was 26.12 g with a juice yield of 24.43%, below the commercial requirements of the standard. This implies a heavier peel weight and a larger diameter for fruits at higher altitudes “Manizales” (Table 3). Thus, for the aforementioned characteristics at 1,010 m asl “Santagueda”, these results align with those obtained by Romero (2019), with mean values of 32% juice weight.
The results also revealed that the citric acid percentage for fruit in the higher-altitude zone was 2.84%, indicating compliance with the standard (less than 5% citric acid). Conversely, fruit from the low-altitude zone registered at 6.18%, above the standard requirements. The soluble solids (SS) content was higher in the lower-altitude zone, recording a value of 7.29 °brix, while the higher-altitude zone achieved a lower value of 6.25 °brix. Additionally, it was observed that the shell thickness of the fruits harvested in the low-altitude zone (1,010 m asl) was 2.30 mm, while the fruits from the higher-altitude zone (2,280 m asl) exhibited a shell thickness of 3.10 mm, marking an increase of 34.78% (Table 3). Previous studies suggest that rootstocks used in citrus farming can influence fruit size and weight, while chemical characteristics such as soluble solids and citric acid seem more connected to environmental conditions or agronomic management of the crop (De Castro et al., 2014; Aregay et al., 2021; De Almeida et al., 2021).
The temperature fluctuation influences the content of total soluble solids and acidity. In relation to acidity, the higher the day/night thermal delta, the lower the concentration of acids (Hernández et al., 2015). According to this, in the case of Tahiti lime, the data suggest that in the locality of Santagueda (1010 m asl), where the variation in the day/night thermal regime is less than the other localities, the fruits could have a higher acidity (Table 3 and Figure 2).
For the study in the two locations, the rootstock used was Sunki x English (SxE), and the fruits showed comparable results between locations for the variables of weight and equatorial diameter (Table 3). However, statistical differences were evident in peel diameter, juice yield, titratable acidity, and citric acid percentage (Table 2). All these variables fall within the classification framework of the NTC 4087 Standard (Icontec, 1997a). According to Arévalo (2017), fruits of the Sunki x English rootstock maintain "extra quality" parameters for weight and size, with a weight between 77.60 ± 6.40 and 102.05±6.52 g and a diameter between 5.03±0.15 and 5.53±0.08 cm. Yet, the variation in citric acid content appears to be more influenced by the altitude at which the crop is grown and less by the rootstock. Hernández et al. (2015) found that localities had climatic differences that must have an influence on the quality of the fruit, a determining aspect for the orientation of the markets. Among the most important preharvest factors that affect fruit quality are edaphoclimatic conditions. Possibly, the most significant of them is temperature, but relative humidity, luminosity, precipitation, type of rootstock and cultural management (pruning and fertilization) are also important for quality (Ríos-Rojas et al., 2020a).
In general, in tropical climate regions where the daily range of temperature variation is low, the fruits tend to be larger, with a greener and thinner yield and a higher percentage of juice, but with a lower content of total soluble solids and acid concentration in the juice of fruits produced in subtropical regions (Hernández et al., 2015). These quality characteristics, determined by the climatic supply, will define the market to which the crops can be directed in each location.
Color of fruits. Concerning fruit color, in the higher altitude zone (2,280 m asl), 62.5% of the harvested fruits were categorized as color 1 (green fruit), 35.9% as color 2 (light green fruit), with a mere 1.5% matching color 0 (well-developed green fruit) based on the 4087 standards (Icontec, 1997b).
At the lower altitude zone (1,010 m asl), a larger proportion, 71.8% of the harvested fruits at 150 days, fit into color 1, with 26.5% falling into color 2 and only 1.5% into color 0. In addition to color, the citric acid content is a helpful indicator of fruit maturity. In the lower altitude zone, this content was observed to be above 5% for colors 1 and 2. This implies that fruits harvested at color 0 may possess lower citric acid levels, thus aligning with the standard requirements. Similarly, the coloring of citrus peels varies among countries and regions due to the significant influence of local climate conditions such as light, ambient environment, temperature, and the type of rootstock utilized on color development (Raddatz-Mota et al., 2019). According to Ríos-Rojas et al. (2020b), the ripening of the fruit on the tree is not uniform, so you can find fruits with yellow and overripe tones or tones, which should be discarded since they are not accepted in international markets.
Temperature fluctuations between day and night can cause the deterioration of chlorophyll and favor the appearance of carotenoids, which affects the homogeneity of the intensity and tone of the color of Tahiti lime. On the other hand, the color of the fruit is also affected by luminosity, which achieves an intense and bright color when it is high (Ríos-Rojas et al., 2020a), while low luminosity induces yellow fruits with less firmness and greater susceptibility to pests and diseases (Romero, 2019).
This study enhances the understanding of the ecophysiological and reproductive behavior of the Tahiti lime across two distinct production zones. It also revealed shifts in the physical and chemical characteristics of the fruits grown in varied environments. It's recommended that future research identify regions with higher production aptitude for Tahiti lime in Colombia.
CONCLUSIONS
At an altitude of 1010 m asl, the floral phenology of the Tahiti lemon presented a shorter time between the buds swollen and anthesis (150 d) compared to the floral phenology at 2280 m asl, which was greater (279 d). There was less accumulation of heat units in the area of the highest altitude (2280 m asl) with 605.1 AHU compared to 159.7 at 1010 m asl.
At an altitude of 2,280 m asl, the Tahiti lime fruits show an increase in polar diameter of 4.50 mm (+6.45%), shell mass of 5,38 g (+7.13%) and shell thickness of 0,80 g (+34.78%) compared to the fruits at an altitude of 1010 m asl.
The fruits at an altitude of 2280 m asl decrease the soluble solids by 1.24°brix (-14.26%), the titratable acidity by 3.34 g of citric acid (-54.04%), the concentration of ascorbic acid by 0.018 mg of ascorbic acid (-28.12%), and the juice yield by 6.3% (-20.50%) compared to the fruits at 1010 m asl.