INTRODUCTION
Soils are indispensable for the provision of several important functions, but agricultural intensification, declining biodiversity, climate variability, greenhouse gases and their focus on increasing primary productivity pose a threat to soil quality (Vázquez et al., 2020), affecting the macro and microorganisms that keep it rich in nutrients. Knowing the soil macrofauna and its distribution is important to predict the degradation state of soil as well as its physical properties and biological components (Navia et al., 2021).
Edaphic macrofauna (e. g., earthworms, termites, ants) have real importance in the transformations of organic matter and physical properties of the soil, establishing channels and pores that favor aeration, drainage, aggregate stability, and water retention capacity, and thus being considered micro- and macro-engineers of the soil and ecosystem (Cabrera-Dávila et al., 2017). The edaphic fauna can be separated into the functional groups of detritivores, fungivores, herbivores, predators, and omnivores according to their feeding habits (Swift et al., 2012).
Soil macrofauna plays a key role in soil processes, regulating the provision of ecosystem services; however, their level of activity depends largely on soil and climatic conditions (Machado-Cuellar et al., 2020). Among their benefits are improving nutrient recycling, decomposition processes, mineralization of organic matter, and improvement of soil structure (Lavelle et al., 2006), of which the diversity, abundance and richness of invertebrates vary depending on the intensity of such agricultural practices (Julca et al., 2006).
Soil macrofauna communities have begun to be considered as bioindicators of soil quality, as they are sensitive to environmental changes and soil conditions that can cause variation in their abundance and composition (Velasquez & Lavelle, 2019). It is understood that the composition of macrofauna depends on climatic factors, plant species and soil properties. In addition, these factors impact the structure, function and composition of soil biota (Karungi et al., 2018).
The combination of trees with crops is a practice with great potential that can provide favorable conditions for soil macrofauna. To this extent, agroforestry systems with greater structural complexity and botanical composition promote the richness and diversity of edaphic macrofauna (Durán-Bautista et al., 2023).
Smallholder agroforestry systems often incorporate features associated with abundant and diverse soil macrofaunal populations (Pauli et al., 2011). Mainly, agroforestry arrangements protect edaphic macrofauna altered by temperature variations and water stress, thanks to the cover they provide to the soil (Lavelle et al., 2003).
In this sense, the implementation of agroforestry systems and the inclusion of promising species represent a sustainable alternative, practices that have been shown to improve the biological properties of the soil (Cabrera et al., 2011).
Multipurpose woody perennials such as wax laurel M. pubecens, with high adaptability in the area and tolerance to external factors, present in their roots the actinomycete of the genus Frankia, which is responsible for nitrogen uptake (Molina et al., 2000), allowing to incorporate this element naturally into the soil and crops (Morales, 2018; Cherubin et al., 2019). For its part, yacon grows well under the shade of trees, shrubs and also in full sun (Seminario et al., 2003); its reservoir roots store high contents of carbohydrates and water that makes them attractive to different species of macrofauna, especially of the order Haplotáxida.
Measuring the abundance, richness, and diversity of edaphic macrofauna in a yacon and wax laurel intercropped cropping system can provide important information for the design and implementation of production alternatives with sustainable practices that generate favorable conditions to improve their ecological functions. Thus, the objective of this research was to evaluate the soil macrofaunal communities in the establishment phase of the silvoagricultural system of laurel M. pubescens intercropped with S. sonchifolius and in degraded kikuyo pasture at the Botana Experimental Farm in the high tropics of Nariño.
MATERIAL AND METHODS
Location. The research was conducted at the Experimental Farm of the University of Nariño, located in Botana. Pasto Altiplano is at 77°18'58''W, 1°10'11.4''N, an altitude of 2820m, an average temperature of 12°C, an average annual rainfall of 800 to 1000 mm, and a relative humidity of 70 to 80% with 900 hours of sunshine per year (IDEAM - Instituto de Hidrología y Meteorología y Estudios Ambientales, 2010). According to the IGAC - Instituto Geográfico Agustín Codazzi (2004), it belongs to the low montane humid forest life zone (bh - MB); taxonomically, this soil is classified as Acrudoxic Fulvudands with an undulating topography.
Soil preparation was carried out manually for the planting of the agricultural component, and for the planting of the tree component, the soil was hollowed out.
Experimental design. It consisted of a Randomized Complete Block design (BCA) with four treatments corresponding to different planting densities of S. sonchifolius intercropped with M. pubescens in an agroforestry system in the establishment phase. T1: S. sonchifolius sown at 1x1m between plants; T2: S. sonchifolius sown at 0.80 x 0.80m between plants; T3: S. sonchifolius sown at 0.50 x 0.50m between plants and a control T4: degraded pasture of C. clandestinum. The treatments were organized in a plot of 60x54m (3.240m2) with three replicates per treatment (12 subplots of 360m2 each); the tree component was sown at 4x4m between seedlings and 9m between rows.
Vegetal material. The vegetal material of S. sonchifolius was acquired from producers linked to the Red de Guardianes de las Semillas, in Nariño. M. pubescens seedlings were acquired from nurseries in the area with an average initial height of 15cm.
Sampling of edaphic macrofauna. For the treatments and control, sampling of edaphic macrofauna was carried out during the productive stage of S. sonchifolius (10 months) after the establishment of the agroforestry arrangement. The Tropical Soil Biology and Fertility (TSBF) methodology described by Anderson & Ingram (1993), modified for this study, was used. In each treatment, including the control, three replicates were selected, and in each one, a sample was taken for a total of nine monoliths. The area of the monolith was 0.0625m² (0.25x0.25m), then this monolith was divided into three strata: 0-10cm, 10-20cm, and 20-30cm deep; the leaf litter stratum was not taken into account due to its minimal contribution in the establishment phase of the system. Macrofauna (diameter ≥ 2mm) was extracted, preserved and labeled. Arthropods were preserved in 70% alcohol and annelids in 5% formalin.
The collected specimens were taken to the specialized Entomology laboratories of the Universidad de Nariño with the help of a Nikon smz 646 stereoscope. The organisms were identified by order and family level using the taxonomic keys of Doreste (1984), Fauchald (1977), and Triplehorn & Johnson (2005). From a functional point of view, the macrofauna was grouped into detritivores, soil engineers, herbivores, and predators according to the functional groups proposed by Cabrera et al. (2011).
Data analysis. Density, richness and diversity analyses were carried out to evaluate the state of the edaphic macrofauna community in the experimental units.
Density. The density of macrofauna associated with each treatment was determined by quantifying the number of individuals/m² (i/m²) of each taxonomic group.
Richness. The richness of the communities found was determined considering the total number of taxonomic groups identified per treatment and depth.
Diversity. Diversity was estimated taking into account the morphospecies of the individuals in each of the treatments evaluated, and the Shannon-Wiener (H) and Simpson's dominance indices were determined (Moreno, 2001).
The information collected in the field was processed in the InfoStat software version 2020. To evaluate the effect of treatment, depth and their interaction on morphospecies density, richness and diversity, a generalized linear mixed effects analysis was performed separately for count variables. Principal Component Analysis (PCA) was performed to determine the taxonomic groups that influence the variability of the data in the treatments.
RESULTS AND DISCUSION
Density. A total of 6,496 i/m² were collected in the sampling carried out (Table 1), composed of eight taxonomic groups with a higher density in T3, corresponding to 48.76% of the total collected, represented mainly by immature Diptera and adult Haplotaxida. T1 with 27.09%, conformed mainly by adult Haplotaxida and adult Coleoptera, followed by treatment T2 with 18.71%, represented mostly by adult Haplotaxida and adult Coleoptera, while T4 (control) presented the lowest density in the study 352 i/m², corresponding to 5.41%, with adult Haplotaxida and adult Coleoptera being the most outstanding.
Table 1 Density of the taxonomic groups by treatment in the agroforestry arrangement of M. pubescens and S. sonchifolius and control at Botana Experimental Farm. Productive stage of S. sonchifolius.
Taxonomic Group | Treatment | Total | |||||||
---|---|---|---|---|---|---|---|---|---|
T1 | T2 | T3 | T4 (control) | ||||||
N° i.p.m² | % | N° i.p.m² | % | N° i.p.m² | % | N° i.p.m² | % | N° i.p.m² | |
Coleoptera (adults) | 320 | 18,2 | 160 | 13,2 | 96 | 3,0 | 112 | 31,8 | 688 |
Diptera (larvae) | 32 | 1,8 | 32 | 2,6 | 1.712 | 54,0 | 0 | 0,0 | 1.776 |
Haplotaxida | 1.296 | 73,6 | 896 | 73,7 | 1.312 | 41,4 | 144 | 40,9 | 3.648 |
Hemiptera | 16 | 0,9 | 0 | 0,0 | 0 | 0,0 | 0 | 0,0 | 16 |
Hirudinea | 0 | 0,0 | 16 | 1,3 | 16 | 0,5 | 16 | 4,5 | 48 |
Milacidae | 64 | 3,6 | 80 | 6,6 | 32 | 1,0 | 32 | 9,1 | 208 |
Polydesmida | 0 | 0,0 | 16 | 1,3 | 0 | 0,0 | 0 | 0,0 | 16 |
Scolopendromorpha | 32 | 1,8 | 16 | 1,3 | 0 | 0 | 48 | 13,6 | 96 |
Total | 1.760 | 100 | 1.216 | 100 | 3.168 | 100 | 352 | 100 | 6.496 |
The data obtained indicate a higher density of macrofauna with respect to land use with agroforestry systems compared to degraded pasture. This agrees with the data obtained by Marichal et al. (2014), where the density of edaphic macrofauna in the studied agroforestry arrangements presented higher values than those of degraded pastures, demonstrating that the negative effects of land use intensification on soil macroinvertebrate communities can be reduced by the implementation of agroforestry practices (Durán et al., 2018).
Linear mixed effects analysis indicated that treatments (F=91.75, P=0.0001), depth (F=664.31, P=0.0001) and interaction (treatment x depth) (F=42.97, P=0.0001) affected the density of individuals. Density of edaphic macrofauna in the soil showed the highest mean values at depths of 0-10cm in treatments T3 and T1, with 928 and 421i/m², respectively, while in the 20-30cm stratum, all treatments, including the control, showed a mean density of 32i/m² (Figure 1). An inversely proportional relationship is observed since at a depth of 0-10cm, the greatest number of individuals is found, and this decreases as the depth increases.
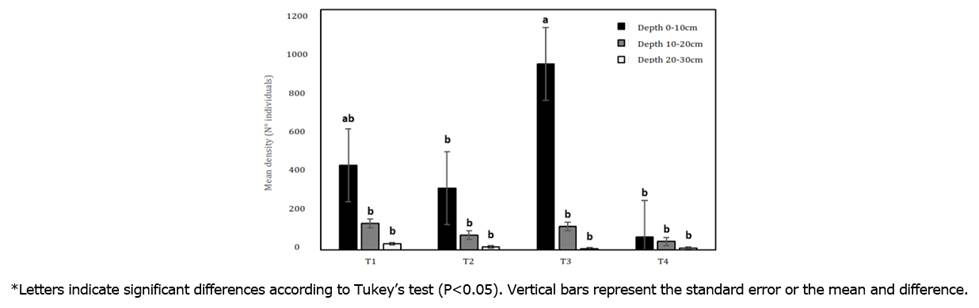
Figure 1 Comparison of the mean density of taxonomic groups between each planting distance treatment and each soil depth at the Botana Experimental Farm.
It can be seen that the agroforestry arrangement of M. pubescens and S. sonchifolius presents the highest density of individuals in strata 1 and 2 compared to the control, Cenchrus clandestinum. A similar situation is reported by Rodríguez-León et al. (2021), where they found that the number of i/m² was higher in forested areas (7202 i/m²) than in degraded pastures (2334 i/m²).
The principal component analysis (PCA) allowed us to identify that the first two components explain 75% of the total variability of the data evaluated (Table 2).
Table 2 Eigenvalues per component of the taxonomic groups.
Components | Eigenvalues | Percentage | Cumulative Percentage |
---|---|---|---|
1 | 3.68 | 46 | 46 |
2 | 2.33 | 29 | 75 |
3 | 1.99 | 25 | 1 |
The first component explains 46% of the variability and is mainly composed of taxonomic groups such as adult Coleoptera, Hemiptera, Hirudinea, and Milacidae (Table 3), which presented a correlation factor between -0.96 to 0.96. The second component showed 29% of the explanation of the total variability, conformed mainly by taxonomic groups such as Haplotaxida (0.98), Diptera (0.68) and Scolopendromorpha with a correlation of -0.78.
Table 3 Contribution of the taxonomic groups for each of the principal components (PC) selected.
Taxonomic Group | PC1 | PC2 |
---|---|---|
Coleoptera | 0,52 | 0,05 |
Diptera | -0,28 | 0,53 |
Haplotaxida | 0,19 | 0,60 |
Hemiptera | 0,50 | 0,11 |
Hirudinea | -0,50 | -0,11 |
Milacidae | 0,29 | -0,03 |
Polydesmida | -0,04 | -0,10 |
Scolopendromorpha | 0,17 | -0,57 |
On PC1 (Figure 2), the projection of Hemiptera, Coleoptera, and Milacidae vectors were related at T1 S sonchifolius sown at 1x1m, while Hirudinea is characterized at T2, T3, and T4; on PC2, Diptera makes the greatest contribution at T3 and Haplotaxida is characterized at T3 and T1, Scolopendromorpha makes its greatest contribution to variability at T1 and T4.
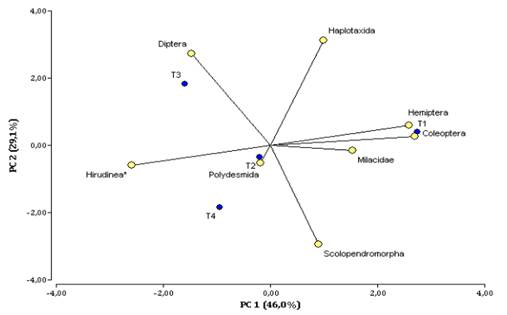
Figure 2 Biplot of the Principal Component Analysis (PCA) for the distribution of the density of taxonomic groups by treatment in the agroforestry arrangement of M. pubescens and S. sonchifolius and control at the Botana Experimental Farm.
In general, a higher occurrence of individuals was found in the treatments with the implementation of the agroforestry system compared to the control treatment (T4). The occurrence of numerous individuals may be due to the presence of strata (herbaceous, agricultural, and arboreal), being a favorable habitat where the different species will fulfill different ecological functions related to the soil cover, the contribution of leaf litter from the trees, the microclimate that maintains appropriate values of temperature, and soil moisture (Granados & Barrera, 2007; Urbano et al., 2019).
In the 0-10cm stratum, the highest density of individuals was found. These results were consistent with Urbano et al. (2019), where the effect of seven green manures on the ecological structure of soil invertebrates was evaluated, finding that these were affected by soil depth and that the highest values in abundance and species richness were found at the soil surface (0-10cm). These results can be explained by the decrease in oxygen and organic matter with increasing soil depth characteristics that provide habitat, hindering the decomposition of humus and food for their development (Villalobos et al., 2000; Castro et al., 2007; FAO, 2019).
The results of this study for the establishment phase of the agroforestry system may be influenced to a greater degree by the agricultural component (S. sonchifolius), which is characterized by a tuberous root reservoir rich in carbohydrates and water, which may explain the greater presence of individuals in the first layer of soil. The arboreal component, on the other hand, over time can favor leaf litter inputs necessary for decomposition processes and biological activity, as reported by Sánchez & Milera (2002), where there was an increase in the density of individuals over nine years, from 194i/m² to 383i/m² due to the incorporation of the tree layer.
According to Wang et al. (2013), N-fixing trees contribute a large amount of organic matter, presenting a total N concentration of up to 50% in the top layer of the soil from 0-5cm. Soil arthropods and Leaf litter are some of the most abundant and diverse communities in forests and provide myriad ecosystem services from decomposition and mineralization to pollination and predation (Cole et al., 2016; Hartshorn, 2021).
In the study by Tapia et al. (2019), they found that soil macrofauna was predominant in the first soil horizons. Leaf litter and 0-10 cm depth in all forest fragments were evaluated, indicating that the social insect groups, Isoptera and Formicidae, were the most predominant in the preserved forest fragment (FFC). The study by Durán & Suarez (2013) for the agroforestry arrangements evaluated indicated that the development of a leaf litter layer in the season of minimum precipitation resulted in a higher density of fauna, showing the importance of this layer to maintain a favorable microclimatic condition.
Richness. The linear mixed effects analysis indicated that depth affected morphospecies richness (F=3.77, P=0.0418), with depth 1 (0-10cm) having the highest values in treatments T2, T3 (3.67) and T1 (3.33), while depth (20-30cm) had the lowest values (1.0) in treatments T4 and T1, respectively. In general, it can be observed that as soil depth increases, the richness of morphospecies decreases. There was no effect of treatments or interaction treatment x depth.
T2 and T1 comprise the seventh and sixth taxonomic groups, respectively, in which Haplotaxida, Coleoptera and Milacidae stand out; treatments T3 and T4 with fifth taxa are mostly represented by Haplotaxida and Hemiptera (T3) and by Haplotaxida and Coleoptera in T4.
The richness of families in the study was higher in the treatments with presence of the arboreal and agricultural stratum and lower in the degraded pasture treatment (T4), coinciding with that reported in other investigations where higher values of family richness were found in polyculture areas and pastures associated with leguminous tree cover, compared to a monoculture of grasses of 30, 26, and 22 families, respectively (Cabrera-Dávila et al., 2017). Tree and/or shrub cover in ecosystem management is of utmost importance as it guarantees the continuous and abundant input of organic plant material and a more favorable microenvironment for the establishment of soil macroinvertebrates (Rodríguez et al., 2002).
The presence of P. clandestinum as an invasive arvense in T4 could have caused the low presence of morphospecies compared to the establishment stage of the agroforestry system. Suárez et al. (2015) reported low values of taxonomic richness of edaphic macrofauna (±3.81 p<0.0001 for the 0-10cm stratum and ±3.87 p<0.0001) due to the presence of monoculture of Dichanthium caricosum L., in addition to the loss of tree cover, which limits the contribution of good quality leaf litter, and the lack of shade on the soil.
Functional groups. The morphospecies of the families identified correspond to different functional groups (Table 4), which are classified according to their impact on the soil and feeding habit: Soil engineers, which constituted the most abundant group in this study, were the Lumbricidae family as the most representative in T3.
Table 4 Functional group composition of macrofauna in the agroforestry arrangement of M. pubescens and S. sonchifolius and control at Botana Experimental Farm.
Taxonomic Group | Family | Treatment | Functional group | ||||||
---|---|---|---|---|---|---|---|---|---|
T1 | T2 | T3 | T4 (control) | ||||||
i/m² | i/m² | i/m² | i/m² | Det | Her | Dep | IdS | ||
Coleoptera | Amphizoidae | 16 | X | X | X | ||||
Carabidae | 32 | 80 | 32 | X | X | X | |||
UF | 16 | X | X | X | |||||
Curculionidae | 32 | 16 | X | X | X | ||||
Chrysomelidae | 16 | 16 | X | X | |||||
Elateridae | 16 | 32 | X | ||||||
Heteroceridae | 16 | X | X | X | |||||
Ptilodactylidae | 32 | 32 | X | X | X | ||||
Scarabaeidae | 112 | 16 | 16 | X | X | X | |||
Staphylinidae | 96 | 16 | 16 | X | X | X | |||
Tenebrionidae | 32 | X | X | X | |||||
Diptera | Bibionidae | 1.680 | X | X | |||||
Cecidomyiidae | 16 | X | X | ||||||
UF | 16 | 16 | X | X | X | ||||
Tipulidae | 32 | 16 | X | X | |||||
Haplotaxida | Lumbricidae | 1.296 | 896 | 1.312 | 144 | X | |||
Hemiptera | UF | 16 | X | ||||||
Hirudinea | UF | 16 | 16 | 16 | X | ||||
Stylommatophora | Milacidae | 64 | 80 | 32 | 32 | X | |||
Polydesmida | UF | 16 | X | ||||||
Scolopendromorpha | Scolopendridae | 32 | 16 | 48 | X | ||||
Total | 1.760 | 1.216 | 3.168 | 352 |
Functional group: Det: Detritivorans, Dep: Predators, Her: Herbivorans, IdS: Soil engineers, U.F = unidentified family.
Soil engineers stand out as the most abundant group in forests and agroforestry systems in environments with greater vegetation cover, richness of exudates, and shade, conditions that benefit these organisms (Morán & Alfaro, 2015). This functional group has a specific impact on the soil interior from the transformation of physical properties, which favor aggregates and structure, movement and water retention, as well as gas exchange (Lavelle et al., 2006).
According to Cabrera-Dávila et al. (2017), the detritivores are responsible for the shredding of plant remains that make up the litter, intervening in the decomposition of organic matter and the recycling of nutrients. Brévault et al. (2007) affirm that the abundance and diversity of this group are associated with the abundance of tree species, greater vegetation cover, high availability, and quality of organic matter, coinciding with the results of this study, where a greater density was found in the silvo-agricultural system treatments compared to the control.
The detritivores were represented by the Bibionidae family in the immature stage, most of which were found in T3 (planting distance of 0.50m) due to a higher density of available plant material. According to Ibáñez (2006), the individuals of this family behave as decomposers, feeding on abundant plant matter and sometimes on the root, as in the case of this study, where the individuals found were mainly in roots of S. sonchifolius, which has fibrous and reservoir roots as a source of food for its exudates and decomposition (Seminario et al., 2003).
Predators were found in all treatments and were represented by the families Carabeidae and Scarabeidae. This group indicates the existence of complex trophic networks (Gullan & Cranston, 2000; Urbano et al., 2019), which are responsible for controlling the availability of habitat resources (FAO, 2019). A high abundance of detritivores, or earthworms, may represent systems with little or no disturbance and a favorable state of fertility. An inverse result evidences more intense conditions of soil disturbance and degradation (Cabrera-Dávila et al., 2017).
Diversity. Linear mixed effects analysis for Shannon's evenness index and Simpson's dominance index showed no significant differences between treatments (p< 0.05).
According to the results, there was a low diversity of morphospecies in the different treatments, including the control, a situation that may be associated with the soil and climatic conditions of the plot, characterized by a clayey texture, low organic matter input from the tree component for the establishment stage and high rainfall during the evaluation period.
This is in agreement with Machado-Cuellar et al. (2020), who state that the composition of soil macrofauna depends on climatic factors, plant species, and soil properties. The organic matter content and the carbon/nitrogen ratio contained in the soil are the conditions that most influence the diversity and functionality of edaphic macrofauna groups (Díaz-Porres et al., 2014). The vegetation community influences the macroinvertebrate community, which may be influenced by specific species that enhance macroinvertebrate diversity (Lavelle et al., 2006).
Different authors affirm that inadequate management practices how logging, intensive tillage, use of agrochemicals, and change of cover and inadequate soil use affect the diversity and density of soil macrofauna communities since they cause abrupt variations in microhabitat conditions (Mathieu et al., 2005).
CONCLUSIONS
The density and richness of edaphic macrofauna in the study were higher in the treatments of the agroforestry arrangement evaluated in the productive phase of S. sonchifolius intercropped with M. pubescens than in the control degraded pasture of Cenchrus clandestinum.
The treatments, depth, and the interaction treatment x depth affected the density of individuals, being higher in the agroforestry arrangement with a higher density of S. sonchifolius in the 0-10cm stratum represented by the taxonomic groups Diptera immatures and Haplotaxida adults.
Depth affected morphospecies richness, with stratum 1 (0-10cm) having the highest values for a number of morphospecies in treatments T1 and T3, while depth (20-30cm) had the lowest values in treatments T4 and T1.
The treatments, depth and their interaction did not significantly affect the diversity of morphospecies, considering a low diversity in both the agroforestry arrangement and the control.