Introduction
The mosquito Aedes aegypti (Linnaeus, 1762) is a vector of dengue, Chikungunya fever, Zika virus, and urban yellow fever. Mosquitoes undergo complete metamorphosis (i.e. they are holometabolous), passing through egg, larval (4 stages), pupal, and adult stages (Araújo et al. 2015; Soares-da-Silva et al. 2015). A. aegypti belongs to family Culicidae, which has wide global distribution in tropical and subtropical areas, with geographic range being limited by altitude and low temperatures only (Funasa 2001). The adaptation of this organism to man-made environments is likely to have caused this synanthropic behavior (Natal 2002; Costa et al. 2012). Population growth and spread of this organism has been exacerbated by human migration from rural areas to urban centers, as well as the lack of basic sanitation (Tauil 2001; Zara et al. 2016).
Systematic control of A. aegypti by government agencies in Brazil began in the mid-nineteenth century, due to a series of national epidemics that led to the death of thousands of people (Funasa 2001). The main tool still used today for control of adult mosquito populations is ultra-low volume pesticide application (ULV) (Paixão 2007). Pyriproxyfen larvicide (Sumilarv®) is used to reduce larval populations, which is involves the ether group chemical pyridyloxypropyl, a juvenile hormone analog (WHO 2007; Santa Catarina 2012). Biological control using natural enemies is also employed (Panplona et al. 2009; Albeny-Simoes et al. 2011, 2012) as well as use of biolarvicides based on Bacillus thuringiensis var. israelensis (Bti) (Arcy et al. 2014). The use of chemicals has not been effective in controlling vector populations or the incidence of dengue (Beserra et al. 2007), and this results in either immediate damage or future risks to the environment and human health (Gomes 2014). Although past attempts were made to artificially select for Bti resistance in the laboratory (Goldman at al. 1986), these methods have thus far been ineffective in A. aegypti (Boyce et al. 2013). In addition, some gaps in literature exist regarding the product concentrations used for control of A. aegypti. The manufacturer of AM65-52 strain VectoBac® WG, recommends a concentration of 0.002 g/L for larval control. The Bti action efficiency under field conditions can be different from totally controlled laboratory experiments, because it can be affected by several environmental factors such as, organic matter and pollutants input, water quality and sun light exposure (Mulla et al. 1990; Pusztai et al. 1991).
The use of plant-based products in pest control has been widely studied (Valladares et al. 1997; Krinski et al. 2014; Busato et al. 2015), mainly due to the high biodegradability, decreased toxicity for non-target species, and the potential of those substances to serve as alternatives to commercially available synthetic compounds (Ghosh et al. 2012). Melia azedarach L., 1753 is fast-growing plant with worldwide distribution in the Meliaceae family, popularly known as cinnamon, lilac or santa-barbaric lily (Kingsbury 1964). M. azedarach has demonstrated insecticidal activity, making it a promising avenue for biological control of mosquito larvae, and has been proposed as a possible larvicide, especially for mosquitoes in the genus Aedes (Rossi et al. 2007; Coria et al. 2008; Busato et al. 2015). Extracts containing active ingredients are produced from plant material via solid-liquid extraction with organic solvents such as ethanol, methanol, ethyl acetate, chloroform, or hexane (Yunes and Calixto 2001). After the macerated plant material is soaked for some time in the solvent, the solution is then evaporated by distillation to obtain a dry extract through high temperature. The main drawback of this technology is the possibility of decomposition of the thermolabile active principles, as well as contamination of the extract by the solvent and impurities contained therein.
Among the technologies used to obtain the plant extracts, supercritical fluid extraction has advantages over conventional extraction methods, including greater extract purity due to the absence of organic solvents, less waste, and lower processing temperatures. Among the solvents used in a supercritical state, carbon dioxide is generally the most popular (Mendiola et al. 2007). This is an interesting solvent for natural products and foods, as in the process can be operated at low temperatures, which allow the extraction of thermolabile compounds. Another advantage of CO2 is its gaseous state at room temperature and pressure, which makes it a very simple recovery and provides free analyst solvents.
This study has two objectives: i) to evaluate the efficiency of supercritical carbon dioxide (SC-CO2) extraction of M. azedarach on the survival of A. aegypti larvae and ii) to evaluate the larvicidal potential of Bti when used in lower concentrations than those recommended by the manufacturer.
Materials and methods
Egg collection
A. aegypti eggs were collected between November 2014 and March 2015 in Chapecó, Santa Catarina State, Brazil (27º05’48”S 52º37’70”W), in areas with high occurrence of this species (Santa Catarina 2012). We installed 16 ovitraps constructed with black plastic 1 L capacity bottles. Three Eucatex® wooden pallets were placed into each trap and replaced each four to six days. The collected pallets were kept in the laboratory at room temperature, and eggs were counted under electronically stereomicroscope, 40X.
Preparation of plant extracts
Ripe M. azedarach fruit were collected in February 2015 in the municipality of Tigrinhos, Santa Catarina state (26º41’13”S 53º90’25”W). The material was stored at -20 °C until extract preparation. The seeds were ground to about 1 mm particle size and placed in an extractor. Supercritical fruit extract was obtained as described by Scapinello et al. (2014a, 2014b), which suggests a temperature of 40 °C and 250 bar pressure with 90 minutes of extraction. The resulting extract was dissolved in 0.5 ml of Tween 80® per 100 ml of distilled water, diluted to the following concentrations: 250 μg/ml, 500 μg/ml, 750 μg/ml, 1,000 μg/ml, and 2,000 μg/ml. Extracts were maintained at 4 °C until use. These M. azedarach extract concentrations were used to evaluate each concentration effectiveness on A. aegypti larvae mortality. Plant extract concentrations were stablished after a previous pilot test (0.0005 g/L) that indicated the positive or negative activity trend of each concentration.
Bti preparation
Vectobac WG® Bacillus thuringiensis var. israelis (wettable powder) was obtained from an agricultural product distributor. The product was weighed on an analytical balance and solubilized in ultrapure water at concentrations of 0.0005 g/L, 0.001g/L, 0.0015 g/L, 0.002 g/L, and 0.0025 g/L. These concentrations were chosen based on dilutions of the recommended concentration by the manufacturer, which kills the 100 % of the exposed larvae.
Bioassays
These were performed in a climate controlled room in the laboratory at 28 °C to 30 °C with a 12:12 L:D photoperiod. Pallets with eggs were placed in trays with untreated, artesian well water and supplied with aquarium fish food (Alcon Basic Complex® MEP 200) for hatching, and larvae were maintained with ample food until reaching second and third instar (L2 and L3).
Ten L2/L3 larvae were then transferred to 180 ml plastic cups containing 100 ml of either experimental solution (water + Bti or plant extract), or control solution (water only). The numbers of surviving larvae were registered at 24, 48 and 72 hours. Each combination of concentration and compound (Bti or M. azedarach extract) was replicated three times.
Statistical analysis
Mean numbers of live larvae were analyzed by ANOVA with means grouped using Duncan’s test with 5 % error probability. Data were log (x+1) transformed prior to analysis. Treatment efficiency was calculated using Abbott’s formula (Abbott 1925).
The mean number of live larvae was analyzed by one-way ANOVA. The factor was treatments at 72 hours exposure time. Treatment factor was composed by six levels, considering M. azedarach concentrations and the control. Statistical difference between each pair of treatments means were analyzed by Tukey post-hoc test (P < 0.05). To adjust error distribution, the data were log (x+1) transformed prior to analysis. Treatment efficiency was calculated using Abbott’s formula (Abbott 1925).
Results
The M. azedarach supercritical extract showed significant (F = 76.6; P < 0.0001) larvicidal action, with 100 % efficiency after 72 hours of larval exposure at 2,000 μg/ml (Table 1). The 250, 500 and 750 mg/mL concentrations did not differ significantly in efficiency from control (Fig. 1). The 1,000 μg/mL had 33.4 % of larval mortality, with results significantly different from all other treatments (Table 2, Fig. 1). The high efficiency was only reached at the highest concentration (2,000 μg/mL) (100 %) (Table 2) after 72 hours of exposure. The Bti was 100 % efficient in all concentrations and at all exposure periods, larval mortality effectively until 24 h exposure time in laboratory conditions.
Table 1 Analysis of variance comparing larvicidal effects of different concentrations of M. azedarach supercritical extract against A. aegypti larvae after 72 hours of exposure. One-way ANOVA, factor: treatments. Levels: M. azedarach concentrations and the control. Tukey post-hoc test (P < 0.05).
Variation | DF | SM | F | P |
---|---|---|---|---|
Between groups | 5 | 38.3 | 76.6 | < 0.0001 |
Within groups | 12 | 0.5 | ||
Total | 17 |
DF = Degrees of freedom; SM = Square means.
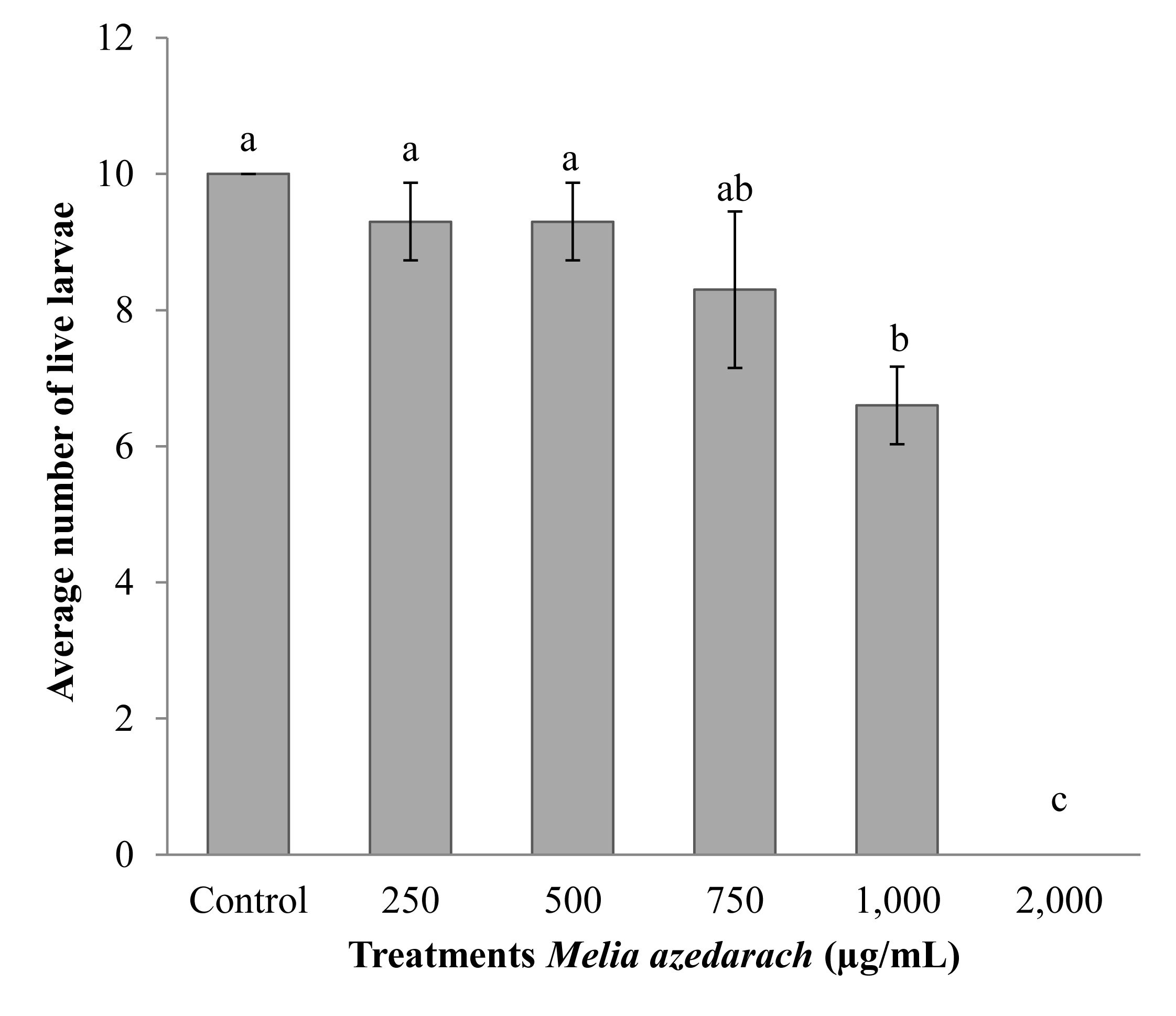
Figure 1 Larvicidal efficiencies of Melia azedarach supercritical extracts at different concentrations. Vertical bars indicate standard error of the mean number of A. aegypti larvae alive after exposure period of 72 hours. Different letters indicate significant differences between treatment groups (Tukey post-hoc test).
Table 2 Average number of surviving A. aegypti larvae after different exposure periods to larvicides and percent efficiency after 72 hours exposure.
Treatment | Mean # surviving larvae | Efficiency (%) | ||
---|---|---|---|---|
24 h | 48 h | 72 h | 72 h | |
Control | 10 | 10 | 10 | 0.0 |
Melia azedarach SC-CO2 | ||||
250 µg/mL | 9.6 | 9.3 | 9 | 10.0 |
500 µg/mL | 10 | 9.3 | 9 | 10.0 |
750 µg/mL | 10 | 9.6 | 8.3 | 17.2 |
1,000 µg/mL | 8.9 | 8.3 | 6.5 | 34.9 |
2,000 µg/mL | 4.9 | 0.3 | 0 | 100.0 |
SC: Supercritical extract.
After exposure period of 72 hours, were observed significant differences between the supercritical extracts of Melia azedarach (M.a.) at different concentrations above the number of A. aegypti larvae alive (Fig. 1).
Discussion
M. azedarach supercritical extract showed larvicidal action. The two smaller concentrations reached 10 % efficiency. At the two highest concentrations, 1,000 and 2,000 μg/mL, larval mortality was observed in 24 hours. However, the average number of live larvae decreased as period of exposure increased in the two highest concentrations (1,000 and 2,000 μg/ml), with 33.4 % and A. aegypti 100 % mortality, respectively. The susceptibility of larvae to this extract may be due to the insecticidal effects of M. azedarach compounds such as azadirachtin (Carpinella et al. 2003). Insecticidal action may also be attributed to other associated compounds, particularly terpenes, sterols and coumarins obtained from M. azedarach during the carbon dioxide extraction (Scapinello et al. 2014b). The Meliaceae family contains plants with the highest known seed terpenoid concentration in the Chapecó region, and these compounds have known larvicidal action (Rossi et al. 2007; Freitas 2008). This result is important and opens a new study window to use M. azedarach on A. aegypti control.
Three of the Bti concentrations used (0.0005, 0.001 and 0.0015 g/L), are lower than the manufacturer’s recommendation (i.e., 0.002 g/L for AM65-52 strain VectoBac® WG) was 100 % efficient in all concentrations and at all exposure periods, larval mortality effectively until 24 h exposure time in laboratory conditions.
Bti is currently the most commonly used bacterium for insecticidal purposes (Silva et al. 2014) and is highly effective in controlling A. aegypti larvae (Lima et al. 2005; Boyce 2013), without provoking toxic effects in humans, either by ingestion or skin contact (WHO 2004). Moreover, it does not show adverse effects to others animal species and is considered non-pathogenic and non-toxic (WHO 1999). Bti has been used in vector control programs for more than 20 years, and there have not so far been records of resistance (Braga and Valle 2007; Boyce 2013).
In this study, a dilution of 75 % of the concentration recommended by the manufacturer was enough to kill all larvae exposed to the product at issue. Generally, the recommended concentrations of a commercial larvicide are several times higher than the lethal concentration obtained under laboratory conditions because in the field there are other factors that may affect the effectiveness of the active compound, such as organic loads, pollution, water temperature and solar light (Mulla et al. 1990; Pusztai et al. 1991; Armengol et al. 2006). Nevertheless, other products are being studied for controlling this vector, such as, for example, vegetal extracts, such as M. azedarach, which is analyzed in this study.
Conclusion
The results herein suggest alternative methods for A. aegypti population control. The extraction method for M. azedarach compounds can be a determining factor to maximize the efficiency of this plant extract as larvicide. In relation to Bti, those results suggest the possibility of this being considered as a powerful tool to be used by the municipality dengue control program.