Introduction
Drosophila suzukii (Matsumura, 1931), the Spotted Wing Drosophila (SWD) is an emerging and invasive pest, native to south-east Asia, that was first recorded in Europe and continental North America in 2008 and currently affects a wide range of important crops, especially berries (Calabria et al. 2012; Walsh et al. 2011; Cini et al. 2012; Asplen et al. 2015). SWD infests healthy, ripening fruits, inserting eggs with its serrated ovipositor (Walsh et al. 2011) and its larvae feed and develop in fruits, which are rendered unsellable, resulting in a dramatic reduction in fruit production and financial losses in soft skinned fruits (Walsh et al. 2011; Silva dos Santos 2014; Andreazza et al. 2016). Average yield reductions attributed to SWD may range from 33% to 50% depending on which crop is affected (Bolda et al. 2010; Goodhue et al. 2011).
Strawberry, Fragaria x ananassa Duchesne (Rosaceae) is one of the most widely consumed berries worldwide with one of the highest growth rates in consumption among fruit and vegetables in the last decades. World strawberry production exceeded 8 million tonnes in 2018 with China being the world’s largest producer, representing 35.5% of global production followed by the USA (15.6%), Mexico (7.8%), Turkey (5.3%), and Egypt (4.4%). Spain, with a harvested area of 7032 ha, and total production of 3,44,679 tonnes (4.1%), ranks sixth (FAO 2020). More than 95% of Spanish strawberry production is located in the province of Huelva (southwest). The climatic and soil characteristics are exceptionally good in the area for strawberry production. Strawberries in Huelva are usually grown as an annual crop, under unheated, high plastic tunnels (Spanish tunnels), whose sides can be rolled up, on raised beds covered with black plastic and fed by drip irrigation. In this planting system, fresh, short-day strawberry cultivars, plants from high elevation nurseries are used. The production season goes from October to June, with fruits harvested from December. This semi-open cultivation system benefits growers as it helps to produce fresh strawberries from the start of the season, thereby enabling international markets to be supplied at an early stage (López-Aranda 2008). In recent years, there has been a focus on developing new strawberry cultivars better adapted to local conditions in southwestern Spain. Currently, over 40 different varieties of strawberry (L. Miranda, pers.com.) can be found in Huelva. However, studies on susceptibility/resistance to insects (the inability or ability of a plant variety to restrict the growth and/or development of a specified pest; https://www.worldseed.org) are not common in the development, agronomic evaluation and recommendations for these varieties, in which production and marketing criteria predominate. ‘Fortuna’, ‘Rociera’, ‘Rábida’, ‘Primoris’ and ‘Calinda’ are the most used strawberry cultivars in Huelva (Medina et al. 2019). In addition, new varieties are constantly being produced and tested in order to meet consumer demand and optimize production.
Strawberries are among the preferred hosts for SWD (Lee et al. 2011; Walsh et al. 2011; Cai et al. 2019) and significant damage and economic losses are caused by this pest in fruit production (EPPO 2022; Arnó et al. 2016; Orhan et al. 2016). SWD management in strawberries usually entails using adult-oriented chemical treatments (Bruck et al. 2011; Burrack et al. 2015). Organophosphates, pyrethroids and spinosyns have been greatly effective against SWD adults (Bruck et al. 2011; Van Timmeren and Isaacs 2013). However, intensive use of synthetic-organic insecticides and spinosyns may induce pests resistant in them (Bruck et al. 2011) and be detrimental to beneficial arthropods (Roubos et al. 2014) as well as being incompatible with organic management. Moreover, overuse may violate the Maximum Residue Limit (MRL) zero tolerance policy for berry markets. Therefore, alternative methods seem necessary for producing more environmentally-friendly crops (Cini et al. 2012; Asplen et al. 2015). The development and deployment of pest-resistant cultivars or tolerant varieties can be an effective approach for reducing damage caused by SWD and nurturing environmentally-friendly crop management as an alternative to chemical control (Sharma and Ortiz 2002). Moreover, an analysis of cultivar susceptibility to pests can also help improve management strategies for growers, identify trap plants and provide particularly significant alternative techniques for organic producers (Zehnder et al. 2007; Cini et al. 2012; Asplen et al. 2015; Poyet et al. 2015).
The susceptibility of berries and other crops cultivars (blueberries, raspberries, blackberries, strawberries, cherries and grapes) to SWD have been analyzed in previous studies (Lee et al. 2011; Linder et al. 2014; Ioriatti et al. 2015; Gong et al. 2016; Baser et al. 2018; Molina et al. 2020). However, there has been little research on strawberries in which the susceptibility of different cultivars to SWD have been analysed. Lee et al. (2011) carried out choice and no-choice tests with ‘Totem’ and ‘Hood’ cultivars in order to determine susceptibility at different stages of ripeness to egg laying and SWD development. However, they did not compare susceptibility between these two cultivars. Likewise, Arnó et al. (2016) demonstrated that SWD was able to oviposit in red, blush, or green ‘Albion’ and ‘Candonga’ cultivars whose host suitability varied at different stages of ripeness in ‘Candonga’ but not in ‘Albion’. Živković et al. (2019) showed statistically higher susceptibility to drosophilids (including SWD) in the ‘Albion’ cultivar compared to ‘Portola’ and ‘San Andreas’. Finally, there are several known genome sequences of strawberry species and hybrids, which provide the opportunity to correlate data on cultivar susceptibility with genotype variations, which will help develop new cultivars or improve existing ones. Gong et al. (2016) investigated the rate of adult SWD emergence from the fruits of 107 accessions of Fragaria identifying at least three of them with significantly reduced fly emergence. Later, Bräcker et al. (2020) noted that one of these resistant accessions displayed unusually enriched methyl anthranilate and found this compound to be a potent agonist against SWD egg development.
In this study, we screened sixteen strawberry cultivars (the most used as well as those recently introduced in southwestern Spain) for their suitability to SWD. Our aims were to elucidate whether SWD infestation varied among these cultivars, and to corroborate the relationships between some organoleptic characteristics of the fruit and certain SWD performance traits.
Materials and methods
SWD experimental colony.
SWD adult females employed in the tests came from an experimental colony established in the IFAPA Laboratory of Entomology, ‘Las Torres’ (Alcalá del Río, Seville; Spain). The colony originated from larvae collected in field-infested raspberries in Huelva (southwestern Spain). The population was reared on berry fruits (mainly blueberries and raspberries). Adults were kept in 30 cm3 cages (BugDorm® 1; Bio-Quip Products Inc., Rancho Rodríguez, CA; USA) at 22±1 °C, 60±5 % RH, and 16:8 h light: dark cycle, and fed with brewer’s yeast, and 10% w/v sugar-dH2O. To prevent endogamy, and ensure genotypic diversity, individuals emerging from naturally infested fruits were introduced into the colony several times during rearing.
Non-choice tests.
We conducted laboratory no-choice tests on sixteen strawberry cultivars: ‘Calderón’, ‘Calinda’, ‘Charlene’, ‘Flaminia’, ‘Flavia’, ‘Fortuna’, ‘Marisol’, ‘Marquis’, ‘Melissa’, ‘Palmeritas’, ‘Petaluma’, ‘Plared0955’, ‘Primoris’, ‘Rábida’, ‘Rociera’ and ‘Sabrina’. They were maintained in experimental plots at the IFAPA Experimental Station of El Cebollar (Moguer, Huelva, southwestern Spain) from which fully ripe, sellable fruits (BBCH-Scale: 87. Main harvest: more fruits coloured; Meier et al. 1994) for tests were collected. The plot was managed in the typical agricultural method for strawberries used in the area (see above, López-Aranda, 2008). The strawberry fruits were carefully selected in order to ensure the same phenological stage. Fruits were subsequently rinsed with dH2O, and examined before testing under a stereomicroscope (Leica MZ6, Leica Microsystems, Germany) to ensure there was no bruising, nor signs of SWD infestation. Ten replicates per cultivar (a total of 160 tests) were performed where in each replicate, one strawberry fruit was exposed to three SWD females (5-12 days old) randomly selected from the experimental colony, confined in individual 30 cm3 cages (see above). After 24 h the fruits were removed from the cages, and individually held in polystyrene rearing flasks lined with filter paper and covered with a mesh lid. The flasks were kept at 22±1 °C, 60.0±5.0 % RH, and 16:8 h light: dark cycle, and were checked for adult emergence for up to 21 days.
For each replicate and cultivar, we recorded the number of emerged adults, sex and days to complete cycle. The time for adult fly emergence was measured in days post-exposure (DPE), with day 1 being the day of exposure itself. After 21 days, each fruit was dissected and the remaining immature stages of the SWD recovered were counted. Adult emergence and fruit dissection accurately estimates actual SWD infestation when counting eggs directly is unreliable or not possible (Shaw et al. 2019). Thus, our measurements of SWD performance included number of adults, apparent emergence rate and number of developing SWD (larvae + pupae) after 21 days and development time.
Fruit analysis.
Strawberry fruit analyses were carried out on 10 fruits (6 in the case of total phenolic and protein content) from the same sample used in the tests. Weight, firmness, pH, and sugar content (°Brix) were measured. For firmness, a TR penetrometer (FDP 500®, Effegi, Italy) fitted with a 1.0 mm diameter probe was used. Two measurements were taken in the equatorial part of each fruit as they lay on a flat, horizontal surface. The mean penetration force (g mm-²) obtained from both measurements was used in the analyses. Sugar content was determined by squeezing the fruits and placing a drop of the juice on a portable refractometer (Eclipse®, Bellingham & Stanley Ltd., UK). This was expressed as °Brix. Afterwards, each fruit was crushed, and a Crimson pH-meter basic 20 (Alella, Spain) was used to determine their pH.
Total phenolic and protein content were determined. For each cultivar, the fruit extract was prepared with 2g of plant material that was weighed and extracted with a mixture of methanol: 0.1% HCl in 50 mL, homogenized using a Polytron® (PT2000, Kinematica AG, Luzern, Switzerland) and filtered in a vacuum using a Kitasato, Büchner funnel, and Whatman #4 filter paper.
Total phenolic content was determined by the Folin-Ciocalteu method as gallic acid equivalents (GAE)/100 g fw (Allen, 1989). Flavonoids in fruit extracts were determined using the colorimetric method of Dewanto et al. (2002), whose results were expressed as mg of catechin equivalents (CE)/100 g fw. Anthocyanin content was determined by the pH-differential method (Giusti and Wrolstad 2001), whose results were expressed as mg pelargonidin-3-glucoside equivalents (P3G)/100 g fw. Finally, for protein determination, fruit flesh was extracted with 0.1% NaOH, following the method described in Jones et al. (1989), whose results were expressed as g of protein per 100 g fw.
Statistical procedures.
All analyses were carried out using R v. 3.1.3 software (R Core Team 2021). Since the normality and linearity of residuals did not match for number of adults emerged and number of larvae and pupae, generalized linear models (GLMs) were ran to test the effects different cultivars had on fruit infestation. GLMs were carried out separately, including total number of adults, number of developing SWD (larvae + pupae) as dependent variables, and cultivars as the factor fitted to a Poisson distribution with a log link function. Where differences were detected by GLM, multiple comparisons, post-hoc Tukey HSD tests (p < 0.05) were performed using the ‘glht’ function in the ‘multcomp’ package (Hothorn et al. 2008). In addition, we checked the models for overdispersion and residual distribution using the DHARMa package (Hartig 2020). When overdispersion was detected, the models were fitted to a negative binomial with a log link function.
Given that days of development, and texture and chemical traits of berries matched normality and linearity of residuals, lineal models (LMs) were used to determine differences in strawberry traits among cultivars. LMs with interaction terms were performed including cultivars and adult sex as factors, and days of development as the dependent variable using the ‘lm’ function from the ‘nmle’ package (Pinheiro et al. 2021). When statistical differences were detected, the ‘glht’ function was also used, as explained above. The correlation between strawberry attributes and SWD infestation data were conducted using a non-parametric Spearman's rank correlation coefficient (ρ).
Results
Significant differences in the number of emerged adults were found among the tested cultivars (LRT χ2 = 1297.1, df=15, p < 0.001). Post-hoc homogenous groups and levels of infestation of the tested cultivars are shown in Fig. 1. ‘Calderon’ and ‘Plared0955’ cultivars displayed the highest number of adults per fruit with an average of 55.8 ± 8.5 and 62.8 ± 6.3 respectively. In contrast, ‘Sabrina’ and ‘Petaluma’ cultivars showed the lowest levels of infestation with an average of 8.1 ± 1.8 and 10.4 ± 2.1 adults per fruit, respectively. In addition, three cultivars presented less than 20 adults per fruit (‘Charlene’, ‘Flaminia’ and ‘Marisol’), five between 20 and 30 (‘Flavia’, ‘Calinda’, ‘Marquis’, Rábida and ‘Rociera’) and four between 30 and 40 (‘Fortuna’, ‘Melissa’, ‘Palmeritas’ and ‘Primoris’).
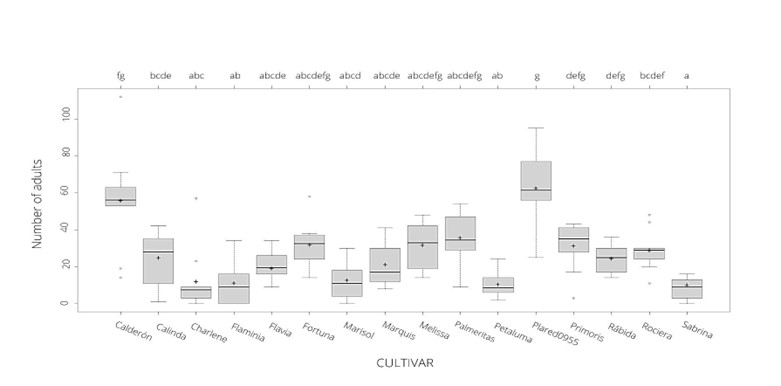
Figure 1 Number of emerged adults in the sixteen strawberry tested cultivars. Cross and line represent the mean and the median, respectively. Outliers are represented by the dots. Different letters denote a significant difference based on generalized linear models (GLMs) among cultivars in which alpha = 0.05.
Significant differences in developing SWD (larvae + pupae) per fruit were also detected among the tested cultivars (LRT χ2 = 85.1, df = 15, p < 0.001). ‘Flavia’ and ‘Melissa’ cultivars presented the highest mean number of developing SWD (7.2 ± 2.7 and 7.3 ± 3.4, respectively) with significant differences in comparison with ‘Charlene’, ‘Flaminia’ and ‘Marisol’ that presented the lowest mean number of developing SWD (0.0 ± 0.0, 0.4 ± 0.3 and 0.8 ± 0.3, respectively) (Fig. 2).
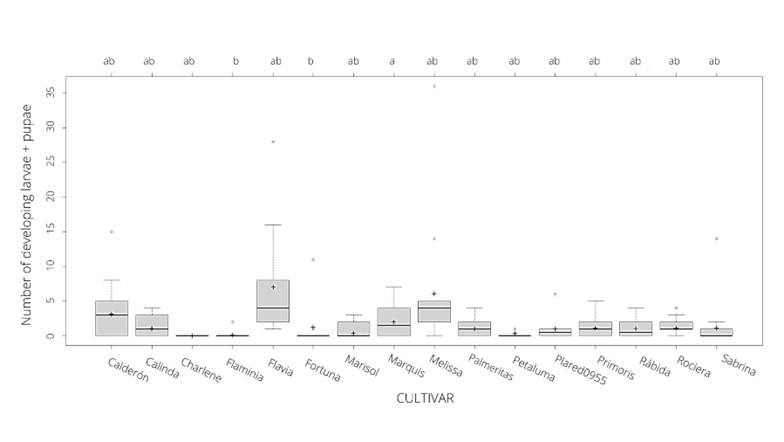
Figure 2 Number of developing (larvae + pupae) detected in the sixteen strawberry tested cultivars. Cross and line represent the mean and the median, respectively. Outliers are represented by the dots. Different letters denote a significant difference based on generalized linear models (GLMs) among cultivars in which alpha = 0.05.
Mean developmental time (expressed as DPE) was 14.00 ± 0.02 days. The LMs revealed significant differences in DPE among the tested cultivars (F15:4110 = 277.3; p < 0.001) between males and females (F1:4110 = 7.4; p < 0.01) and in the interaction between these factors (F15:4110 = 2.8; p < 0.001). In fact, DPE was shorter in males (13.95 ± 0.03 days) than in females (14.02 ± 0.03 days). Consequently, when both sexes were analysed separately, significant differences in developmental time among the cultivars were found for males (F15:2033 = 117.0; p < 0.001) and females (F15:2077 = 166.1; p < 0.001). Regarding the former, ‘Fortuna’ cultivar showed the lowest mean developmental time (12.4 ± 0.05 days) with significant differences with respect to the other cultivars (Fig. 3A). In contrast, ‘Calderón’ and ‘Petaluma’ presented the highest time for males (15.9 ± 0.06 and 15.2 ± 0.2 days, respectively) differing significantly between them (Fig. 3A). In addition, with ‘Calinda’, ‘Charlene’, ‘Flaminia’ and ‘Palmeritas’ there were no significant differences in these times, which ranged from 14.7 ± 0.08 to 14.3 ± 0.09 days (Fig. 3A). Finally, there were no significant differences among the other nine cultivars, whose values ranged between 13.2 ± 0.07 and 13.6 ± 0.1 days (Fig. 3A). A similar pattern was obtained for females. Once again, ‘Fortuna’ displayed the lowest time (12.5 ± 0.05 days) with significant differences with respect to the other cultivars (Fig. 3B). ‘Calderón’ (16.2 ± 0.06 days) followed by ‘Petaluma’, ‘Calinda’ ‘Flaminia’, ‘Palmeritas’ and ‘Charlene’ (15.2 ± 0.09, 15.2 ± 0.02, 15.0 ± 0.1, 14.6 ± 0.08 and 14.4 ± 0.09 days, respectively) presented the highest time for females. Finally, the other nine cultivars presented no significant differences, ranging from 13.2 ± 0.08 to 13.7 ± 0.2 days (Fig. 3B).
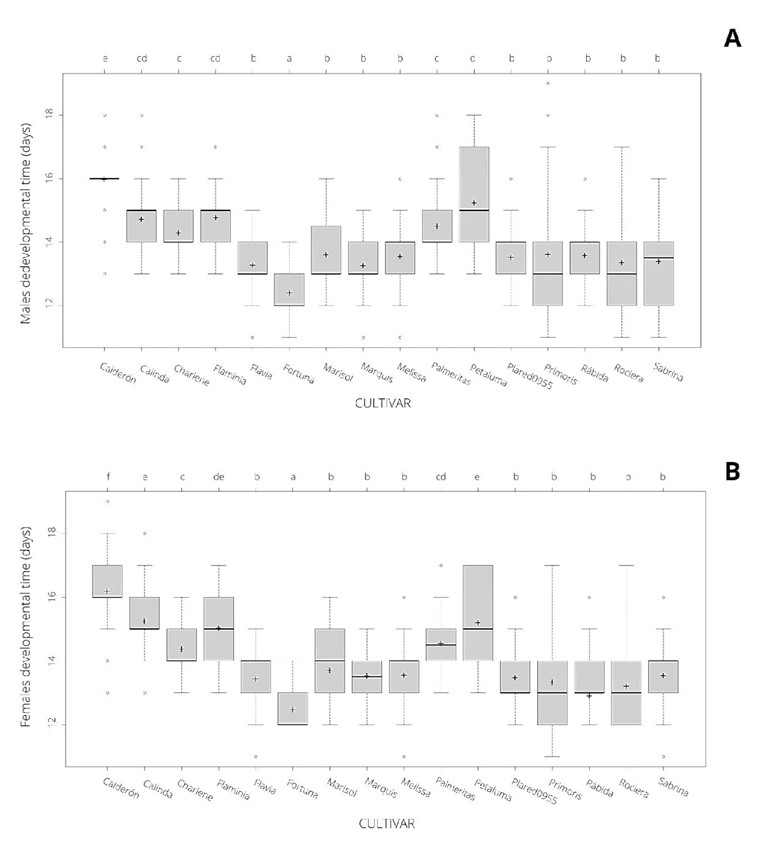
Figure 3 Mean developmental time (expressed as days post exposure) for males (A) and females (B) in the sixteen strawberry tested cultivars. Cross and line represent the mean and the median, respectively. Outliers are represented by the dots. Different letters denote a significant difference based on generalized linear models (GLMs) among cultivars in which alpha = 0.05.
Strawberries from the different cultivars varied in their quality and chemical attributes (Table 1). Fruit size (expressed as g fresh weight) differed significantly (F15,144 = 5.2, p < 0.001) with ‘Calinda’ showing the highest value and ‘Flaminia’ the lowest. Firmness also differed significantly (F15,144 = 8.6, p < 0.001) with the minimum being 171. 9 ± 12.2 g mm-2, for ‘Charlene’, and the maximum, 303.9 ± 10.3 g mm-2, for ‘Sabrina’. Results also showed significant differences in sugar content (F15,144 = 24.2, p < 0.001). ‘Primoris’ and ‘Marisol’ had the highest and lowest values respectively (18.7 ± 0.8 and 7.3 ± 0.4). Similarly, there was great variation in their pH values (F15,144 = 17.4, p < 0.001) which ranged from 2.6±0.04 in ‘Calinda’ to 3.7 ± 0.03 in ‘Calderón’. Protein content and total phenolics also varied significantly among cultivars (F15,80 = 12.0, p < 0.001 and F15,80 = 35.6, p < 0.001, respectively). Protein content ranged from 0.18 ± 0.04 g/100g in ‘Fortuna’ to 0.46 ± 0.08 g/100g in ‘Flavia’. ‘Calinda’ showed the highest total phenolics value and ‘Rábida’ the lowest. Anthocianyns and flavonoids also varied significantly (F15,80 = 246.9; p < 0.001; F15,80 = 31.5; p < 0.001, respectively). Sabrina and Rociera presented the highest and the lowest values of anthocyanins, respectively. Finally, flavonoids ranged from 21.1 ± 1.3 g/100g in ‘Fortuna’ to 56.7 ± 1.1 g/100g in ‘Flaminia’.
No correlation between fruit quality and chemical attributes and infestation parameters (number of emerged adults, developing SWD and DPE) was found (p < 0.05 in all cases). Likewise, there was no correlation between infestation (number of emerged adults and number of developing SWD) and DPE (p < 0.05 in all cases).
Table 1 Fruit traits (mean ± SE) measured from sixteen strawberry cultivars. Size (F15,144=5.2, p < 0.001), Firmness (F15,144=8.6, p<0.001), Sugar content (F15,144=24.2, p < 0.001), pH (F15,144=17.4, p < 0.001), Protein content (F15,80 = 12.0, p < 0.001), Total Fenolics (F15,80 = 35.6, p < 0.001), Anthocyanins (F15,80 = 246.9; p < 0.001) and Flavonoids (F15,80 = 31.5; p < 0.001). Letters denote significant differences by Tukey’s HSD (p < 0.05).
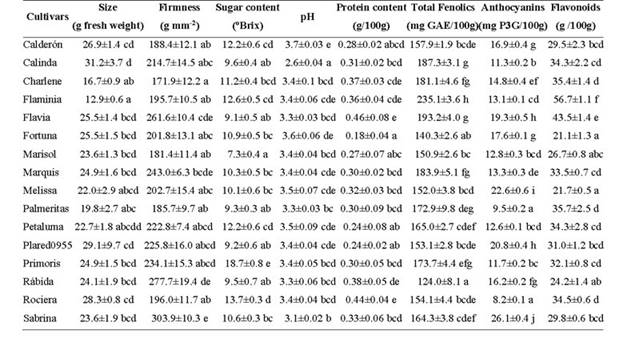
Discussion
The sixteen strawberry cultivars tested in this study showed susceptibility to SWD, and suitability for larval development, although notable differences were found among them. Few previous studies have analysed susceptibility or identified pest-resistance in strawberry cultivars. Lee et al. (2011) and Arnó et al. (2016) carried out choice and no-choice tests with ‘Totem’ and ‘Hood’, and ‘Albion’ and ‘Candonga’ cultivars, respectively, in order to determine susceptibility at different stages of ripeness for egg laying and SWD development. However, they did not compare susceptibility between cultivars. Likewise, Bernardi et al. (2017), in a no-choice bioassay, using the strawberry cultivar, ‘Albion’, reported significant differences in fruit susceptibility to SWD infestation at different stages of ripening, but in a choice bioassay, females preferred to oviposit on ripe fruits. Gong et al. (2016) found great variation in emergence among 107 strawberry accessions ranging from 0 to 16 emerged adults per fruit. In addition, differences in the emergence of SWD adults has been reported for fruit collected in the field from ‘Albion’, ‘San Andreas’, and ‘Portola’ strawberry cultivars (Živković et al. 2019). According to the levels of infestation obtained herein, the most susceptible cultivar (‘Plared0955’) presented 7.75 times more emerged adults than the least susceptible one (‘Sabrina’), indicating notable variation between the cultivars tested. Currently, ‘Fortuna’ and ‘Rociera’ are those most grown in southwestern Spain, comprising over 65% of total cultivated strawberries (Medina et al. 2019). In this study, these two cultivars displayed a mid-level of infestation with at least eight cultivars showing lower values. Thus, it is clear that further research and field observations are required to confirm these differences in susceptibility, especially before new cultivars are established in any given area.
Significant differences were also detected in developmental time between males and females and among tested cultivars. Recently, such differences were also detected among blueberries cultivars, but not between males and females (Molina et al. 2020). In addition, Gong et al. (2016) found differences in adult emergence time ranged from 13 to 17 days after exposure. These variations in developmental time might have been linked to hight density of larvae developing inside the fruit. In this respect, Bezerra Da Silva et al. (2019) found a decrease in egg-pupa development times for SWD in blueberries due to intraspecific competition under laboratory conditions. However, in this paper, no correlation between infestation and developmental time was found. For instant, the most susceptible cultivar (‘Plared0955’) displayed intermediate developmental times. Thus, other factors must explain these differences in developmental times among cultivars.
Although strawberries from the different cultivars varied in their quality and chemical attributes, no correlation between fruit quality, chemical traits and infestation parameters was found. The fruit characteristics were responsible for the differences in preference and performance among fruits remains unclear (Olazcuaga et al. 2019). In previous studies, several of the physical and/or chemical attributes of the berries were related to SWD infestation (Lee et al. 2011; Kinjo et al. 2013). Links were found to fruit firmness or penetration force (Burrack et al. 2013; Ioriatti et al. 2015; Baser et al. 2018; Molina et al. 2020), fruit size (Gong et al. 2016; Stringer et al. 2017) and pH (Lee et al. 2016; Little et al. 2017; Rodríguez-Saona et al. 2018; Molina et al. 2020). Moreover, the brix level of the fruits (Lee et al. 2011, 2016; Stringer et al. 2017) was a contributing factor in the susceptibility of the fruits to SWD infestation. However, other authors yielded variable results, whose conclusions were not always consistent (Little et al. 2017; Pelton et al. 2017; Rodríguez-Saona et al. 2018). In addition, other elements such as proteins and lipids are known to affect development time and reproduction (Royes and Robertson 1964; Tu and Tatar 2003; Olazcuaga et al. 2019). Results obtained herein found no correlation between fruit quality and the chemical attributes measured, and SWD infestation. Therefore, other factors may be key to explaining differences in susceptibility to SWD among the strawberry cultivars tested. For instance, some strawberry compounds (e. g. methyl anthranilate) have recently been identified as limiting factors in SWD development and, to a certain extent, affect the egg hatching rate (Bräcker et al. 2020). Further research and field observations are required in order to shed light on which fruit attributes determine differences in susceptibility to SWD in strawberry cultivars in southwestern Spain. Different levels of infestation may also be due to the repellent effect of ingredients or volatiles of the cultivars or to the different development conditions of the instars in the fruit (with equally strong oviposition). This point could not be elucidated in this work since the proportion of hatched eggs could not be recorded. Therefore, experimental design of future research must take this factor into account in order to determine in detail which processes explain the differences in susceptibility among cultivars. In practice, the significant differences in susceptibility detected herein could help improve SWD management in southern Spain. In fact, the cultivars that have shown a lower level of infestation (‘Sabrina’, ‘Petaluma’) are not among those most used by growers in southern Spain (Medina et al. 2019), which indicates that there is room for improvement in SWD management with the use of the appropriate cultivar. Finally, efforts are necessary to reconcile susceptibility studies with agricultural and socio-economic factors involved in the selection of cultivars by growers.
In summary, the sixteen strawberry cultivars tested in this study showed susceptibility to SWD, with significant differences in the range of infestation. ‘Calderon’ and ‘Plared0955’ cultivars displayed the highest number of adults per fruit, in contrast to ‘Sabrina’ and ‘Petaluma’ that showed the lowest level of infestation. Significant differences were also detected in developmental time between males and females and among the cultivars. Unlike in previous studies, no correlation between quality and chemical fruit traits, and infestation parameters was found. However, it must be stressed that strawberry cultivars in the field should be selected on the basis of an analysis of berry demand and agricultural and socio-economic factors, whose results do not necessarily have to coincide with those reported herein. Since strawberry farming is constantly adapting to market demand, more laboratory and field studies in this area are required in order to analyse how planting strawberry cultivars less susceptible to SWD would help to design IPM programmes.