Introduction
Photoperiod provides essential information about temporally spaced environmental signals such as dawn and dusk that can be easily observed in the daily behavior of insects including motor activities, e.g., mating behavior and oviposition (Beck, 1980).
The peaks of activity are driven by the circadian clock and can be synchronized by light, which is the predominant Zeitgeber. Light is considered one of the most important external factors for synchronization (entrainment) and is best characterized at the molecular level (Lazzari, 1992; Meireles-Filho & Kyriacou, 2013; Özkaya & Rosato, 2012).
The circadian clock of vector insects controls several aspects of behavior and physiology essential for their survival (Meireles-Filho & Kyriacou, 2013). These insects adapted their circadian rhythms to explore specific environmental conditions and have activity periods that correspond to the rest periods of hosts and predators (Barrozo et al., 2004; Clements, 1999).
In disease-vector insects, photoperiodism and the amount of light affect patterns of motor activity, e.g., mating behavior and oviposition. Particularly, in mosquitoes, a behavioral activity often includes mating swarms at dusk, consuming sugar and blood diets, oviposition, and interaction with parasites (Barrozo et al., 2004).
Studies on the direct effect of different photoperiod regimes on the biology of anopheline immatures, including an important malaria vector in Northern Brazil especially in the Amazon region Anopheles darlingi Root, 1926 (Costa et al., 1988; OPAS, 2018) were not found. But several adult variables, e.g., fat body size in Anopheles crucians Wiedmann, 1828 (Lanciani, 1993), size and longevity of Anopheles colluzi Coetzee & Wilkerson, 2013, and Anopheles arabiensis Patton, 1905 (Huestis et al., 2017) from larvae reared in different photoperiods are usually investigated.
Although An. darlingi was recently colonized Brazil (Araujo et al., 2019), no studies have been conducted on the photoperiod conditions and their effect on the rearing of this species in the laboratory. More importantly, Gil et al. (2015) reported that Aedes aegypti (Linnaeus, 1762), which are frequently found in breeding sites submitted to natural photoperiods in our region, i.e., 12 hours of light and 12 hours of dark, successfully colonized cesspits under darkness conditions in urban areas of Northern Brazil.
Therefore, this study investigated the use of three different photoperiod conditions, i.e., 24 L:0 D (constant light), 0 L:24 D (constant dark), and 12 L:12 D (12 hours of light and 12 hours of dark) on several biological variables of A. darlingi, including survival and larval development, adult production, sex ratio, and longevity.
Materials and methods
Capture and rearing.
Anopheles darlingi females were collected on a rural property 31 km from Porto Velho, RO (08°39′S, 063°56′W), using protected human catches with the help of a handheld aspirator from 6 p.m. to 8 p.m.
The mosquitoes were placed in screened polyvinyl chloride (PVC) cages adapted from Arruda et al. (2017) and fed in the field with human blood for 15 minutes using an artificial feeder with polytetrafluoroethylene membrane (PTFE) (Teflon®) adapted from Siria et al. (2018).
In the laboratory, mosquitoes were maintained in screened PVC cages with 10 % sucrose, identified at the species level using dichotomous keys (Consoli & Lourenço, 1994), and then induced to oviposit by removing one of the mosquito’s wings (Lanzaro et al., 1988) using an entomological forceps and a stereomicroscope. After wing removal, females were kept in screened plastic cages lined with moistened filter paper for oviposition for 48 hours. The eggs obtained were placed in water for larval hatching. The larvae were fed with ground fish feed (TetraMin® Tropical Flakes - Zanin et al., 2019), and adults were fed with 10 % sucrose. The insectary conditions were set to 26 °C -28 °C with relative humidity of 70 %-80 %.
Photoperiod manipulation and Anopheles darlingi rearing.
Plastic trays used for rearing immatures and screened cages for adults of A. darlingi were positioned about 20 cm from two 40-watt fluorescent lamps (Atman, 15,000 K, tri-power light), which provided about 3,000 to 5,000 lux of light intensity. Three different experimental conditions were set up: (i) 12 L:12 D (12 hours of light and 12 hours of dark), (ii) 24 L:0 D (constant light), and (iii) 0 L:24 D (total dark), in which the trays were covered with a blackout, except during daily maintenance and feeding (about 10 minutes/day). The photoperiod was controlled by an analog timer, and the trays or cages were rotated daily.
Larval rearing was performed with 50 second-instar larvae placed in a plastic tray (25 × 15 × 6 cm) containing 300 mL of dechlorinated filtered water. Larval feeding was controlled by providing 0.6 mg to 30 mg of feed/day/50 larvae, according to their stage of development (Araújo et al., 2012).
The trays were cleaned daily, and the water (300 mL) was changed after checking the temperature with a thermometer and the light intensity with the free application Lux-o-Meter v1.10.2 via smartphone.
Pupae were transferred to plastic cups containing 50 mL of filtered water and placed in screened polyvinyl chloride (PVC) cages, and adults were kept in screened cages and fed with a 10 % sucrose solution using cotton pads.
Experiments were performed with four replicates and repeated twice using larvae obtained from females collected on different field captures.
Biological variables.
Daily larval survival, development time, pupation rate, emergence rate, male/female ratio, and adult longevity were recorded. The larval survival rate of the different treatments was observed daily by counting dead larvae in each tray. Larvae that did not move after a major disturbance in the water were considered dead. Development time was estimated by the time each larva was required to reach the pupa stage. The pupation rate was obtained by the ratio between the number of pupae obtained divided by the number of initial larvae. The emergence rate was obtained by the ratio of the number of adults collected divided by the number of pupae. The ratio between males and females obtained from each treatment was estimated by dividing the number of males by the number of females obtained in each experimental condition. Adult longevity was obtained by the difference between the dates of emergence and death of 10 males and 10 females in screened cages fed with 10 % sucrose solution.
Statistical analyses.
Larval survival comparisons were analyzed by the Mantel-Cox test; development time was analyzed by the Kruskal-Wallis test (nonparametric ANOVA) and comparisons using Dunn’s test; pupation rate, emergence, and male/female ratio were analyzed by one-way ANOVA and comparisons using Tukey’s multiple comparisons tests; and the effects of treatments and the sex of adults on longevity were analyzed by two-way ANOVA and comparisons using Tukey’s multiple comparisons tests. All analyses were performed in the Prism 9 program (Graph Pad Inc.) at a significance level of 5 %.
Results
Significant differences were observed between the different photoperiods in larval survival (χ2 = 33.3; P < 0.0001). Overall, at the end of development, about 73 % of the larvae survived when exposed to 12 hours of direct light, 60.2 % when exposed to constant dark, and 56.6 % when exposed to constant light (Figure 1).
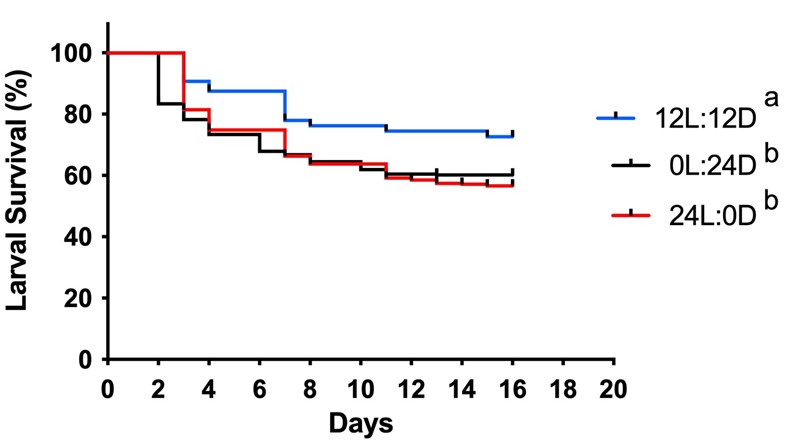
Figure 1 Larval survival of Anopheles darlingi (Diptera: Culicidae) in different photoperiodic conditions. 12 L:12 D = 12-hour photoperiod; 0 L:24 D = constant dark; 24 L:0 D = constant light. Different letters indicate significant differences between treatments (P < 0.05) (N = 8 trays).
Larvae reared with light for 24 hours took one more day to develop than those with other treatments (H = 128.3; P < 0.0001) (Figure 2). Overall, except for the constant light treatment (8.6 days), larvae under other types of treatment took about 6.9 to 7.1 days to pupate.
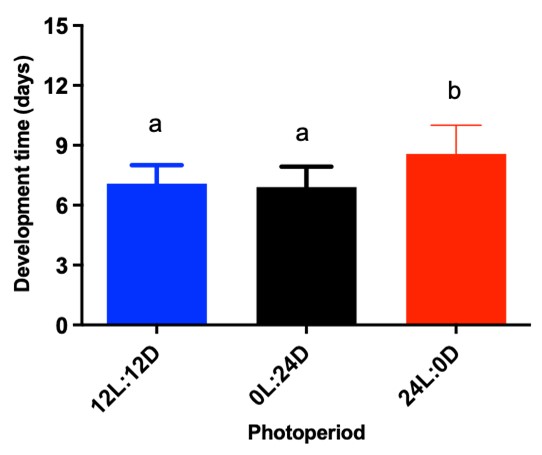
Figure 2 Larval development (mean ( standard deviation) of Anopheles darlingi under different photoperiodic conditions. 12 L:12 D = 12-hour photoperiod; 0 L:24 D = constant dark; 24 L:0 D = constant light. Different letters indicate significant differences between treatments (P < 0.05). (N = 190 larvae/treatment)
The pupation rate was not significantly affected (F = 2.11; df = 2; P = 0.14) but tended to be lower in groups under constant light (58 %) and in the dark (60 %), ranging from 77 % to 73 % (Figure 3).
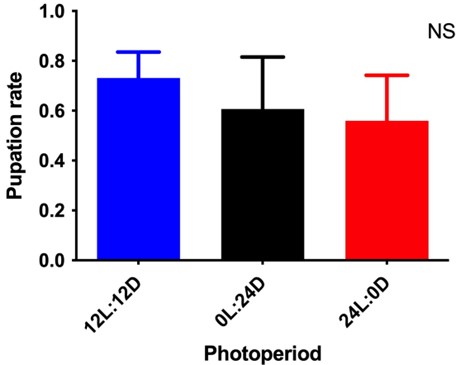
Figure 3 Pupation rates of Anopheles darlingi (mean ( standard deviation) under different photoperiodic conditions. 12 L:12 D = 12-hour photoperiod; 0 L:24 D = constant dark; 24 L:0 D = constant light. NS = no statistically significant difference (P > 0.05) (N = 8 trays).
Anopheles darlingi’s emergence rate was higher in the treatment with a 12-hour photoperiod (92 %) than in the constant light group (79%) (F = 5.68; df = 2; P = 0.012) and even the constant dark group (81 %) (Figure 4).
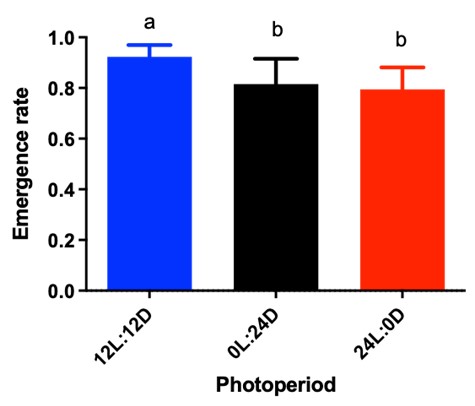
Figure 4 Emergence rates of Anopheles darlingi (mean ( standard deviation) under different photoperiodic conditions. 12 L:12 D = 12-hour photoperiod; 0 L:24 D = constant dark; 24 L:0 D = constant light. Different letters indicate significant differences between treatments (P > 0.05) (N = 8 trays).
The male/female ratio of A. darlingi was also affected by the various light regimes (F = 7.3; df = 2; P = 0.0007) from the different photoperiods. The male/female rate was greatly lower in constant light (0.3) than in the 12-hour treatment (0.95) and constant darkness (0.81) (Figure 5).
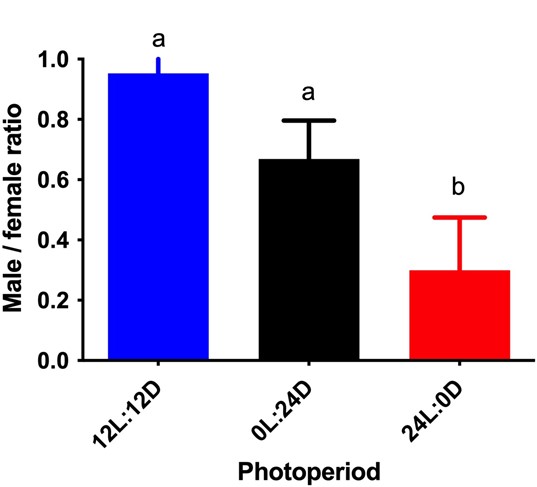
Figure 5 Male/female ratio (mean ( standard deviation) of Anopheles darlingi larvae reared under different photoperiodic conditions. 12 L:12 D = 12-hour photoperiod; 0 L:24 D = constant dark; 24 L:0 D = constant light. Different letters indicate significant differences between treatments (P > 0.05) (N = 8 trays).
Adult longevity was not affected by the photoperiod treatments (F = 0.14; df = 2; P = 0.84) (Figure 6). In general, females (20.8 days) had longer life spans than males (17.2 days) (F = 9.1; df = 1; P = 0.003) (Figure 6).
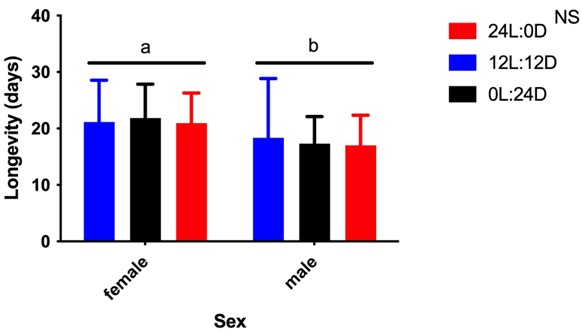
Figure 6 Longevity (mean ( standard deviation) of male and female Anopheles darlingi larvae reared under different photoperiod conditions. 12 L:12 D = 12-hour photoperiod; 0 L:24 D = constant dark; 24 L:0 D = constant light. Different letters indicate significant differences between treatments. NS = no significant differences (P > 0.05). N = 20 mosquitoes per sex and treatment.
Overall, 12 hours of light was the most appropriate condition for A. darlingi rearing regarding the parameters of survival, adult emergence, and the male/female ratio (Figures. 2, 5, and 6, respectively).
Discussion
Rearing A. darlingi under constant light or in the dark significantly reduced larval survival (13 %). Similarly, Ukubuiwe et al. (2018) reported a significant reduction (50 %) in the survival of immature Culex quinquefasciatus Say, 1823 (Diptera: Culicidae), exposed to light regimes of 24 hours. On the other hand, many immatures of this species (98 %) survived in total darkness, which may be an adaptation to the nature of some of its natural breeding sites, including cesspits, drains, and cisterns (Consoli & Oliveira, 1994), with almost no incidence of light. Interestingly, despite a 10 % reduced larval survival under constant dark compared to 12 hours of photoperiod, A. darlingi collected under natural photoperiod conditions in the field in the present work completed their development in the dark, suggesting that photoperiod is not a determinant condition to the survival of this mosquito species.
Studies with other arthropods, including crabs, Pseudocarcinus gigas Lamarck (Decapoda: Menippidae) (Gardner & Maguire, 1998), hemipterans, Orius niger Wolff (Hemiptera: Anthocoridae) (Bahşi & Tunç, 2008), and mollusks, Dysopeas muibum Marcus and Marcus (Mollusca: Subulinidae) (Pilate et al., 2014), suggested that the photoperiod does not affect the survival of these organisms but could affect other biological parameters.
Anopheles darlingi had a longer development time under constant light than under other treatments, which corroborates data on another mosquito species, Culex quinquefasciatus (Ukubuiwe et al., 2018) and suggests that reduction of teneral reserves of mosquito larvae reared under constant light extends the larval development time. Regarding other arthropods under the same light condition, Camus and Zeng (2008) reported that immature copepods of Acartia sinjiensis Mori (Calanoida: Acartiidae) had a shorter development time than those raised in total darkness or under 12 hours of light and 12 hours of darkness. In vertebrates, Gutierrez et al. (1984) used tadpoles of the Mediterranean painted frog, Discoglossus pictus Otth (Anura: Discoglossidae), in an experiment with increasing photoperiods, i.e., from 1 hour to 24 hours of light exposure, finding that longer photoperiods or constant light prolonged development time and decreased the size of the tadpoles’ head and tail. A similar study conducted with the American bullfrog, Rana catesbeiana Shaw (Anura: Ranidae), showed that 24 hours of light increased development time and decreased the metamorphosis rate (Bambozzi et al., 2004).
Present data on the pupation rate of A. darlingi corroborate those of Hoffman and Subramanian (2005), who studied the effects of light exposure in the final stages of the development of Anopheles stephensi Liston, 1901 (Diptera: Culicidae), larvae and found that larvae reared in 12 L:12 D alternation or the dark had no significant changes in pupation rate, this may have resulted from the increase in larval development time to compensate for the decrease in general reserve of mosquitoes reared in the constant light regime as reported by Ukubuiwe et al. (2018). Besides, the rearing of Aedes taeniorhynchus Wiedemann, 1821 (Diptera: Culicidae), in the dark compared to 12 L:12 D photoperiods resulted in smaller pupation peaks but did not affect the pupation rhythm, i.e., the number of pupation peaks in 24 hours (Nayar, 1967).
The reduced adult emergence of A. darlingi from immatures reared under dark condition also corroborates the results of Hoffman and Subramanian (2005), who found greater differences in the emergence of adult A. stephensi but reported an even higher effect of a photoperiod differing from 12 L:12 D, since 94.4 % of adults emerged in the 12 L:12 D regime and only 60.8 % emerged in the group without light.
Photoperiods differing from the 12 L:12 D regime greatly affected the sex ratio of A. darlingi, reducing male production under the constant light condition and might resulted from sexual dimorphism across photoperiods different from 12 hours. Yee et al. (2012) reported that female Aedes albopictus (Skuse, 1894) took longer to develop and were heavier than males when reared in stable longer photoperiods. On the other hand, the use of constant light or dark did not affect the sex ratio of the parasitoid wasp Campoletis perdistinctus Viereck (Hymenoptera: Ichneumonidae) (Hoelscher & Vinson, 1971) or of the copepod Acartia sinjiensis Mori, 1940 (Camus & Zeng, 2008).
The longevity of A. darlingi was not affected by the photoperiodic conditions used for rearing this species, but present data corroborate the results of Araújo et al. (2012), in which A. darlingi females had greater longevity (15.6 days) than males (11.8 days). However, the response to the photoperiod seems to depend on the species, since shorter photoperiods increased longevity for the mosquito Anopheles coluzzii (Diptera: Culicidae) but not for Anopheles arabiensis (Diptera: Culicidae) (Huestis et al., 2017).
Anopheles darlingi larvae usually develop in environments well exposed to sunlight or in partially shaded areas (Deane et al., 1986). The length of the photoperiod in the region where this study was conducted, i.e., at low latitudes, varies little and lasts around 12 hours. This indicates that major changes in the photoperiod, with the potential induction of a free-running period by constant light or dark, are not suitable for A. darlingi rearing, since these treatments negatively affected the assessed parameters the most (Figures 2, 4, and 6) and were the experimental groups with higher larval mortality (Figure 2). Interestingly, A. darlingi completed its development under these unusual photoperiodic, e.g., constant darkness. Moreover, Dias et al. (2019) reported that this species develops under chronic exposure to pollutants such as ammonia, suggesting that this mosquito species might adapt to new breeding site conditions such as dark and polluted cesspits as reported for another mosquito vector, e.g., Ae. aegypti (Gil et al., 2015).
Conclusions
In general, the 12-hour photoperiod was the most appropriate for A. darlingi rearing among all experimental conditions used. Despite that, A. darlingi was able to complete its development under unnatural light conditions that might be found in unusual breeding sites for this mosquito species. This study is the first report of the effects of different photoperiodic conditions on A. darlingi development.