Introduction
Biodiesel is produced from vegetable oils or animal fats, and Brazil is among the largest producers and consumers of biofuel in the world due to its high production and availability of raw materials (Cremonez et al., 2015). The demand and production of biodiesel has boosted the availability of glycerin, the main coproduct of biodiesel production (Adilson et al., 2011). Among the potential applications, glycerin has been studied as an alternative feed for pigs because it has a palatable sweetness and an energy value similar to corn. Therefore, this ingredient can be used as an source of energy for pigs during different growth stages (Huepa et al., 2015; Madrid et al., 2013).
Glycerides may present variation in composition due to differences in the raw materials as well as the processes employed in the production of biodiesel. All these generate varying degrees of purity (Oliveira et al., 2013) and nutritional value, especially in regards to glycerol and fatty acids content (Kerr et al., 2009).
Groesbeck et al. (2008) recommended the inclusion of 12% crude glycerol in the diet of growing pigs. They reported an increase in daily weight gain and feed intake, as well as improved physical properties of pellets. By contrast, Gallego et al. (2014) reported that dietary addition of semi-purified glycerin up to 14% did not affect feed intake, weight gain, nor feed/gain ratio of growing-finishing pigs. These differences in results show that there is no general consensus in arriving at the optimal dietary level of glycerin that can be recommended for pigs.
In monogastric animals, dietary glycerol is absorbed by the stomach and intestines and transported to the liver to be metabolized. Thus, depending on the nutritional status of the animal, glycerol can be used in lipid formation or energy production. In a situation of surplus energy, glycerol acts as a precursor of triglyceride synthesis, while in an energy shortage situation, it is used to supply carbon skeletons for gluconeogenesis or for energy production through glycolysis and Krebs’s cycle (Lin, 1977). However, few studies (Hansen et al., 2009; Huepa et al., 2015) have investigated how the use of glycerol in pig diets affects metabolism.
This study aimed to evaluate the effects of adding increasing amounts of semi-purified glycerin to the diets of growing pigs, and study growth performance and serum levels of glucose, triglycerides, cholesterol, and urea.
Materials and Methods
Ethical considerations
This study was carried out in April 2015 at the Universidade Federal de Mato Grosso, Campus Universitário de Sinop (Brazil). The study protocol was consistent with the ethical principles for animal experimentation adopted by the National Council for Animal Experimentation Control, and was approved by the Ethics Committee on Animal Use of the Universidade Federal de Mato Grosso (protocol 23108.700673/14-4).
Husbandry and diets
Forty commercial barrows, with an average body weight of 27.30 ± 1.74 kg, were used in the experiment. Pigs were housed in a brick shed divided into 20 pens, with asbestos cement tiles.. The pens had feeders in the front and nipple waterers in the back area. The animals were randomly distributed in a complete block design with four treatments, five replicates per treatment, and two animals per experimental unit (pen). Initial body weight of the pigs was used as the criterion for the formation of the blocks.
The treatments (Table 1) consisted of corn-soybean meal diets containing 0, 5, 10, or 15% semi-purified glycerin. Dietary treatments were formulated following Rostagno et al. (2011) recommendations. The salt content was adjusted to provide the recommended levels of sodium. However, diets with 0 and 5% semi-purified glycerin, were added with more salt than the 10 and 15% diets due to the higher concentration of minerals in glycerin.
Table 1 Composition (dry matter basis) and nutritional value of the diets containing semi-purified glycerin.
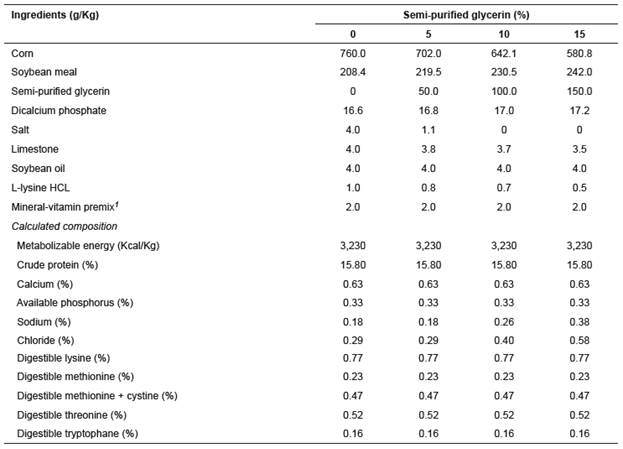
1 Composition per kg of diet: Cu (1,000 mg), Fe (6 mg), Zn (8 g), Mn (3 g), Se (28 mg), I (80 mg), Co (50 mg), cholin (10 g), vitamin A (550,000 IU), vitamin D3 (125,000 IU), vitamin E (3,000 IU), vitamin K3 (250 mg), nicotinic acid (2 g), pantothenic acid (1,200 mg), folic acid (25 mg), biotin (1 mg), vitamin B1 (80 mg), vitamin B2 (250 mg), vitamin B6 (160 mg), vitamin B12 (600 µg), etoxiquin100 mg), BHT (240 mg), colistine (3.2 g).
Semi-purified glycerin (Table 2) was obtained from a soybean oil biodiesel producing company, and had 3,280 kcal/kg metabolizable energy (Verussa, 2015).
Table 2 Chemical composition of semi-purified glycerin.
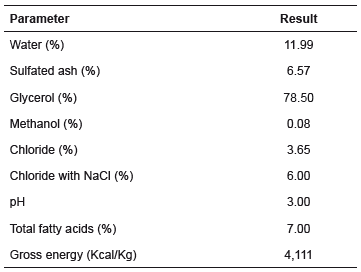
Source: Relatório de ensaio Tanque 344-2-012 de 10 de fevereiro de 2015. Fiagril Ltda, Lucas do Rio Verde, MT, Brasil.
Feed and water were offered ad libitum to the pigs throughout the experimental period. Pen cleaning with scraping of waste was conducted daily; water blasting was performed weekly.
Data collection and chemical analysis
The experiment lasted 27 days and was divided into two periods. Period 1: days 0-20; and Period 2: days 21-27. The animals were weighed at the beginning and end of each period, and the feed quantities and leftovers were recorded. These data were used to calculate the average daily feed intake (ADFI), average daily weight gain (ADWG), and feed/gain ratio (FGR) of the experimental units.
In the morning of the first (initial) and last (final) days of the experimental, 8 mL of blood was collected from the jugular vein of all animals after an8-h fasting. . Then, 4 mL of blood was transferred into vacuum tubes containing gel tab to determine triglycerides, cholesterol and urea levels; other 4 mL of blood was transferred into tubes containing sodium fluoride for glucose analysis.
All samples were centrifuged at 3,000 rpm for 15 minutes immediately after collection. Serum was obtained from the tubes with a gel separator (Vacuette do Brasil Ltda, Campinas, SP, Brasil), and plasma with sodium fluoride was transferred into cryovial tubes, which were then labeled and refrigerated until laboratory analysis on the same collection. LABTEST® diagnostic kits (Labtest Diagnóstica S.A., Lagoa Santa, MG, Brasil) containing reagents for the analysis of urea (sodium salicylate, sodium nitroprusside and urease), glucose (glucose oxidase, peroxidase, 4-aminoantipyrine, phenol, sodium azide and surfactants), cholesterol (phenol, sodium cholate, sodium azide, 4-aminoantipyrine, cholesterol esterase, cholesterol oxidase and peroxidase), and triglycerides (magnesium acetate, 4-chlorophenol, 4-aminoantipyrine, lipoprotein lipase, glicerolquinase and sodium azide) were used, along with a semiautomatic biochemical analyzer (AAKER Solutions Ltda, Porto Alegre, RS, Brasil) for determination of serum parameters.
Statistical analysis
The experiment was carried out according to a randomized complete block design, with initial body weight of the animals used to form the blocks. Analysis of variance was conducted according to the statistical model shown below:
where:
Yijk= is the observation regarding the effect of i glycerin level, j block and k replicate
μ= is the general mean
Gi= is the effect of glycerin inclusion levels
Bj= is the effect of j blocks
εijk= is the random error associated with each observation.
The effects of glycerin levels were evaluated by partitioning the treatment sum of squares into orthogonal contrasts to assess the linear, quadratic and cubic effects. The evaluations had an error probability of 0.05 for type I. The data were subjected to the mixed procedure of the SAS software Statistical Analysis System - Version 6 - (SAS Institute, Inc., Cary, NC, USA), with 5% probability.
Results
Performance
In Period 1, growth performance of the pigs showed no difference (p>0.05) caused by the glycerin level (Table 3). In Period 2, a linear increase in ADWG (p<0.05) was observed as a function of glycerin level. During the total experimental period, a linear increase in ADWG (p<0.05) and an improvement in FCR (p<0.05) were observed in the animals fed increasing levels of glycerin.
Table 3 Average daily feed intake (ADFI), average daily weight gain (ADWG), and feed/gain ratio (FGR) of growing pigs fed diets containing semi-purified glycerin.
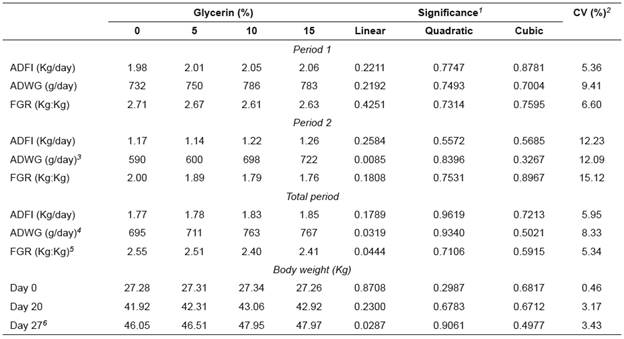
1 Significance level (p<0.05).
2 CV, coefficient of variation.
3 ŶADWGP2 = 0.5780 + 0.0099 X (R²=0.3689).
4 ŶADWGTP = 0.6859 + 0.0059 X (R²=0.1851).
5 ŶFGRTP= 2.5217 - 0.0116 X (R²=0.2701).
6 ŶBody weight TP = 45.1197 + 0.1577 X (R²=0.0787).
There was a linear effect (p<0.05) on the body weight at the end of the experimental period, with those animals fed increasing dietary glycerin levels showing increased final weights. The other performance parameters were not influenced (p>0.05) by the inclusion of glycerin (Table 3).
Serum biochemical indicators
The serum levels of urea, cholesterol, triglycerides and glucose were not affected (p>0.05) by the inclusion of glycerin in the diet (Table 4). There was an increase (p<0.05) in urea and glucose comparing the initial and final measurements, while cholesterol and triglycerides did not differ (p>0.05) between both collections.
Discussion
Performance
Glycerin effects were not observed on feed intake despite the high sodium content in diets with higher inclusion of glycerin. Increase in ADFI of pigs fed diets containing glycerin can be expected due to the sweet flavor of glycerin, which improves palatability (Groesbeck et al., 2008), however, higher sodium content can limit feed intake. The acidification processes (HCl) used for glycerin purification, and the neutralization base (NaOH) (Ooi et al., 2004) used to adjust pH can alter its composition and produce glycerin that is approximately 80% glycerol with low methanol levels and increased NaCl and K levels (Gallego et al., 2014). Carvalho et al. (2013) utilized crude glycerin, while Gallego et al. (2014) and Gonçalves et al. (2013) utilized semi-purified glycerin and achieved similar results, reporting no effects on feed intake.
However, Schieck et al. (2010) observed that pigs fed a diet with 8% crude glycerin had greater ADFI compared to the control diet. A study by Zijlstra et al. (2009), registered that nursery pigs fed wheat-based diets containing 0, 4 and 8% crude glycerin, increased ADFI quadratically. Stevens et al. (2008) also found that crude glycerin had a positive linear effect on ADFI. These different responses in feed intake could be due to the marginal reduction in metabolizable energy of diets containing glycerin when ingredient substitutions were not corrected to achieve similar energy values in the diets. This might have altered feed intake.
The energy value of glycerin is dependent on its composition, particularly its glycerol and fatty acid contents, which are inversely proportional and are related to the production efficiency (Kerr et al., 2009). The commonly called pure glycerin has more glycerol and less fatty acid contents. On the other hand, glycerin with less glycerol and more fatty acids can have higher energy content. In addition, methanol, sodium and mineral matter levels may also impair the quality of the product, which has led to the establishment of a quality standard in Brazil (BRASIL, 2010). Gott and Eastridge (2010), analyzing 16 samples of glycerin obtained from different raw materials and industries, stated that the ash content has a wide variation due to the quantum of catalysts used in each industry. Oliveira et al. (2013), analyzing 41 samples of glycerin from 16 biodiesel plants, registered 0.61-2.82% sodium levels, and 2.3-12.1% mineral matter levels, which demonstrates its high variation.
The ADWG increase in Period 2, and the FCR and ADWG improvements in the total experimental period may be related to a possible improvement in nutrient digestibility due to inclusion of glycerin in the diet. Madrid et al. (2013) stated that crude glycerin can be considered as a highly digestible food, and found a linear increasing effect on nutrient digestibility coefficients in pigs were fed diets containing glycerin. Furthermore, ileal digestibility of glycerol is very high (>99%) for diets containing crude glycerin (Oliveira et al., 2014). In addition, Silveira et al. (2015) evaluated crude glycerin (0, 5, 10 and 15%), and registered that the digestible energy coefficient increased with the addition of glycerin, due to the fact that it is very digestible compared with regular feed. According to Robergs and Griffin (1998), glycerol is absorbed through the portal system and goes straight to the liver; after that, glycerin is transformed into glycerol-3-phosphate by glycerol kinase, and then it is oxidized to dihydroxyacetone phosphate on the outer face of the inner mitochondrial membrane, and goes through the glycolytic pathway for energy production. The results of the present study are in agreement with Orengo et al. (2014), who found higher ADWG values in pigs fed 10% crude glycerin compared with a control diet.
Nevertheless, our results differ with previous works that found no difference in performance of growing pigs fed diets with 10% crude glycerin (Lammers et al., 2008), 12% semi-purified glycerin (Gonçalves et al., 2013); 14% semi-purified glycerin (Gallego et al., 2014); and up to 16% semi-purified glycerin (Gonçalves et al., 2014). However, those studies reported that glycerin can be fed to growing pigs at those levels without adverse effects on animals performance.
By contrast, Madrid et al. (2013) found a decrease in ADWG and ADFI of growing pigs by the addition of 5% crude glycerin in the diet; such decrease adversely affected animal performance. The authors attributed the variability of results regarding the use of glycerin to the precision of each study in detecting statistically significant differences, the purity of the glycerin used, and possible interactions with other ingredients in the diet.
The improvement in gain in Period 2 alongside with the lack of effect on growth performance in Period 1 suggests that glycerin does not affect animal performance in the short term; however, if the length of the feeding period is increased, glycerin may affect the performance of pigs. This is in agreement with Schieck et al. (2010), who evaluated a corn-soybean meal control diet, long-term and short-term crude glycerin diets, and registered that pigs fed a long-term diet had greater ADWG than the control diet, while the short-term diet had an ADWG similar to the control. Berenchtein et al. (2010), who studied pigs fed with up to 9% semi-purified glycerin in different growth periods, also found no difference in the performance in the first period of evaluation (33-65 kg body weight); however, in contrast with the present study, a decrease in ADWG was observed in their second period.
Serum biochemical indicators
In growing swine, 80% of fatty acid is a result of de novosynthesis, using glucose as substrate, because only a low proportion of calories in their normal feed is present as fatty acids, and about 40% body glucose usage is destined for fat deposition in adipose tissue (José et al., 2006). The addition of glycerin reduced corn inclusion in the experimental diets and, therefore, lower the substrate for synthesis and transport of fatty acids by the liver, suggesting a reduction of circulating cholesterol and triglycerides. However, this was not observed in this study, possibly because the diets had the same metabolizable energy, driving the animals at the same energy balance and, consequently, the same potential lipogenic levels.
Lin, (1977) using glycerol, and Gallego et al. (2014) using semi-purified glycerin, studied glycerol as a source of readily available energy. Depending on the nutritional status of the animal, glycerin can be used to form lipids or to produce energy by using glucose. Therefore, in this study, the glycerin added is assumed to affect the serum levels of substances that are involved in metabolism. However, this hypothesis was not confirmed by the experimental results obtained in the present work due to the absence of effect of glycerin inclusion on serum parameters.
These observations are in agreement with Gallego et al. (2014), who found no differences in the serum levels of glucose, cholesterol and plasma triglycerides in pigs fed diets containing up to 14% semi-purified glycerin. With increased dietary glycerol, blood levels of glycerol may also increase (Kijora et al., 1995), but the metabolism of excessive plasma glycerol occurr through gluconeogenesis (Gallego et al., 2014). Similarly, Hansen et al. (2009), Madrid et al. (2013) and Oliveira et al. (2014) found that inclusion of 5, 16 and 18% crude glycerin in the diet of pigs had no effect on plasma glucose. Huepa et al. (2015) also showed that inclusion of up to 12% semi-purified glycerin in pig diets had no effect on blood glucose levels; however, inclusion of glycerin promoted a linear increase in triglyceride content. The authors explained that the increased triglycerides in blood was associated with the increased concentration of free fatty acids and glycerol, with part of the latter driven from the bloodstream to the tissues for energy production. Similarly, Carvalho et al. (2012) registered a quadratic effect on cholesterol levels of pigs fed diets containing up to 12% crude glycerin.
The length of the period of supply may have been insufficient to significantly affect serum parameters. Differing from the present results, Carvalho et al. (2012) observed an increase in plasma triglyceride levels in relation to the sampling period (sample of the 14th and 22nd days), possibly due to the need for a longer period of adaptation to diets containing glycerin.
Regarding urea, the results of the present work indicated that when up to 15% semi-purified glycerin was provided to growing pigs, there is no mobilization of protein from tissues, reflecting an adequate supply of amino acids. These results were similar to those reported by Lammers et al. (2008) using crude glycerin.
Even the levels of glucose and urea in the final collection were higher than those in the initial collection, and all values were within the reference ranges (85-150 mg/dL, and 21.4-64.2 mg/dL for glucose and urea, respectively; Duncan et al., 2003). Because the average urea and glucose contents did not differ across glycerin levels, it can be inferred that glycerin had no effect on these parameters, and the difference between periods of collection resulted from the physiological responses of animals.
Serum biochemistry (no effect) along with ADWG and body weight (linear effect) results of this study suggests that glycerin utilization does not meet the maximum response in growing pigs. This might be correct, because glycerin metabolism is based on the fact that glycerol from the diet is absorbed by passive diffusion (Pluske, 2007), is turned into glucose in the liver via phosphorylation to glycerol-3-phosphate (Mourot et al., 1994), but an excess will be excreted in the urine (Oliveira et al., 2014). This result could be indicative of saturation of the metabolic pathways of glycerol due to the limitation of glycerin conversion to glycerine-3-phosphate by glycerin kinase. However, Papadomichelakis et al. (2012) found that glycerol kinase mRNA expression in the liver of pigs increased linearly when glycerin increased from 0 to 15% in the diet during 42 days of feeding, indicating no saturation effect, which possibly occurred in the present study.
In conclusion, the addition of up to 15% semi-purified glycerin to the diet of growing pigs improved body weight, daily weight gain and FGR without affecting serum levels of urea, triglycerides, cholesterol and glucose.