Introduction
Phosphorus (P) is an essential mineral for the metabolism, growth and development of broilers, and is especially important for bone structure. According to Scholey et al. (2018), broilers currently experience a substantial incidence of bone abnormalities and lameness that hinder performance and cause economic losses to the poultry industry. They attribute these unwanted characteristics to genetic selection for rapid growth. In addition, malabsorption of minerals such as calcium (Ca) and P can also contribute to the incidence of diseases related to bone tissue and performance loss of broilers.
Broiler feeds based on corn and soybean meal have high levels of P (about 0.25% in corn and 0.56% in soybean meal). However, up to 70.0% of this mineral may be present as phytic P, which is poorly absorbed by non-ruminant animals (Karimi et al., 2011). Broiler digestion of phytate is low since they have low endogenous phytase activity for hydrolyzing the phytate complexes present in feed (Woyengo and Nyachoti, 2011). Thus, adding phytase to the diet can improve P digestibility, performance and bone quality of broilers.
Exogenous phytase has become an alternative to feed supplementation with P because it can hydrolyze phytic acid and its salts (phytate), thereby releasing inorganic P and other minerals (Fukayama et al., 2008). Thus, phytase is commonly included in diet formulations to reduce P supplementation, improve diet use and, consequently, reduce excretion of this and other minerals into the environment.
Phytase efficiency can be increased when used in diets with reduced levels of P (Souza et al., 2015). Nonetheless, the use of unconventional doses of phytase to achieve nearly complete phytate hydrolysis resulting in improved performance depends not only on the release of phosphate, but also on the type of phytase and whether or not there is enough P and energy in the diet (Wu et al., 2015).
Thus, this study aimed to evaluate the effects of including two bacterial phytases (Escherichia coli) in diets with normal and reduced levels of P on growth performance and bone quality of broilers.
Materials and methods
Ethical considerations
The local Ethics Committee for Animal Research approved the experimental protocol (Protocol 240/2013).
Location and experiments
This study was conducted in the city of Igarapé, Minas Gerais, Brazil (20°4'20" S, 44°18'12" W). Two experiments were conducted each using 720 male broiler chicks (Cobb). Chickens were housed in 24 experimental boxes (12 birds/m2) with pendular drinkers and tubular feeders. The birds received 24 h of artificial light (250 W heating lamp) until 14 d of age, after which they received natural light until the end of the experiment. The chicks had ad libitum access to feed and water throughout the experiments. Both experiments were analyzed using a completely randomized design, with four treatments and six repetitions with 30 birds. The treatments were defined by the level of available phosphorus (AP) and the inclusion of one of two commercial phytase enzymes (Table 1). The chicks were reared from age one to 35 days.
Table 1 Treatments used in Experiments I (Normal available P levels) and II (Reduced available P levels).
Experiment I | Normal available phosphorus levels | ||
---|---|---|---|
Treatment | Available P (starter feed) | Available P (grower feed) | Enzyme |
Positive control | 0.45% | 0.40% | Without |
Phytase X | 0.35% | 0.30% | With |
Phytase Y | 0.35% | 0.30% | With |
Negative control | 0.35% | 0.30% | Without |
Experiment II | Reduced available phosphorus levels | ||
Treatment | Available P (starter feed) | Available P (grower feed) | Enzyme |
Positive control | 0.35% | 0.30% | Without |
Phytase X | 0.25% | 0.20% | With |
Phytase Y | 0.25% | 0.20% | With |
Negative control | 0.25% | 0.20% | Without |
Enzyme X - Escherichia coli derived 6-phytase.
Enzyme Y - Escherichia coli derived 6-phytase, produced by the yeast Pichia pastoris in a submerged fermentation.
The commercial enzymes tested were of bacterial origin: phytase X is an Escherichia coli-derived 6-phytase developed specifically for use in swine and poultry feeds, while phytase Y is an E. coli-derived 6-phytase produced by the yeast Pichia pastoris in a submerged fermentation process. 500 units of phytase-FTU- per kg of feed were included, with the nutritional value of the enzymes being 0.10% AP. In addition to the contribution of phytase to AP, the experiments also considered an apparent metabolizable energy of phytase (AME) of 33 kcal/kg, crude protein (CP) of 0.22% and calcium (Ca) of 0.1%.
Diets were formulated using corn, soybean meal and meat and bone meal, and were divided into starter (one to 21 days) and grower diets (22 to 35 days). The nutritional levels of the diets were calculated using the nutritional values of ingredients according to Rostagno et al. (2011). Diet composition, with their respective nutritional values, are provided in tables 2 and 3 for experiments I and II, respectively.
Table 2 Composition and calculated nutritional values of the diets according to the treatments and phases of Experiment I.
Ingredients (%) | Positive control | Enzyme X | Enzyme Y | Negative control | ||||
---|---|---|---|---|---|---|---|---|
1 to 21 | 22 to 35 | 1 to 21 | 22 to 35 | 1 to 21 | 22 to 35 | 1 to 21 | 22 to 35 | |
Corn 7.26% | 59.50 | 63.50 | 60.40 | 64.50 | 60.40 | 64.50 | 60.40 | 64.50 |
Soybean meal 47% | 31.20 | 27.00 | 32.30 | 28.00 | 32.30 | 28.00 | 32.30 | 28.00 |
MBM 41.86% | 5.00 | 4.30 | 3.20 | 2.60 | 3.20 | 2.60 | 3.20 | 2.60 |
Soybean oil | 2.70 | 3.50 | 2.10 | 2.90 | 2.10 | 2.90 | 2.10 | 2.00 |
Limestone 37% | 0.33 | 0.49 | 0.74 | 0.80 | 0.75 | 0.80 | 0.75 | 0.80 |
Common salt | 0.40 | 0.38 | 0.42 | 0.40 | 0.42 | 0.40 | 0.42 | 0.40 |
DL-Methionine 99% | 0.29 | 0.25 | 0.28 | 0.24 | 0.28 | 0.24 | 0.28 | 0.24 |
L-Lysine 98% | 0.18 | 0.19 | 0.17 | 0.18 | 0.17 | 0.18 | 0.17 | 0.18 |
Mineral vitamin supplement1, 2 | 0.20 | 0.20 | 0.20 | 0.20 | 0.20 | 0.20 | 0.20 | 0.20 |
L-Threonine | 0.09 | 0.08 | 0.07 | 0.06 | 0.07 | 0.06 | 0.07 | 0.06 |
Choline chloride 70% | 0.05 | 0.04 | 0.05 | 0.04 | 0.05 | 0.04 | 0.05 | 0.04 |
Salinomycin* | - | 0.05 | - | 0.05 | - | 0.05 | - | 0.05 |
Nicarbazine+ | ||||||||
Maduracimin* | 0.05 | - | 0.05 | - | 0.05 | - | 0.05 | - |
Halquinol 60% | 0.005 | 0.005 | 0.005 | 0.005 | 0.005 | 0.005 | 0.005 | 0.005 |
Enzyme X (5,000 FTU 100 g/T) | - | - | 0.1 | 0.1 | - | - | - | - |
Enzyme Y (10,000 FTU 50 g/T) | - | - | - | - | 0.05 | 0.05 | - | - |
Chemical composition (calculated on natural matter basis) | ||||||||
Met. Energy (kcal/kg) | 3,050.0 | 3,151.0 | 3,050.0 | 3,151.0 | 3,050.0 | 3,151.0 | 3,017.0 | 3,118.0 |
Crude Protein% | 21.5 | 19.5 | 21.5 | 19.5 | 21.5 | 19.5 | 21.3 | 19.3 |
Calcium% | 0.96 | 0.89 | 0.96 | 0.89 | 0.96 | 0.89 | 0.86 | 0.79 |
Available phosphorus% | 0.45 | 0.40 | 0.45 | 0.40 | 0.45 | 0.40 | 0.35 | 0.30 |
Lysine (digestible)% | 1.17 | 1.06 | 1.17 | 1.06 | 1.17 | 1.06 | 1.16 | 1.05 |
Methionine (digestible)% | 0.59 | 0.53 | 0.58 | 0.53 | 0.58 | 0.53 | 0.58 | 0.53 |
Met+Cystine (digestible)% | 0.85 | 0.77 | 0.85 | 0.77 | 0.85 | 0.77 | 0.84 | 0.77 |
MBM - meat bone and meal; 1Mineral vitamin supplement (initial phase), each ton contains: 9,000 IU of vit. A; 2,500 IU of vit. D3; 14.0 mg vit. E; 2.0 mg vit. K3; 2.5 mg vit. B1; 6.2 mg vit. B2; 4.0 mg vit. B6; 14.0 mcg vit. B12; 40.0 mg of niacin; 15.0 mg of pantothenic acid; 1.0 mg of folic acid; 10.0 mg of copper; 50.0 mg of iron; 80.0 mg of manganese; 60.0 mg of zinc; 1.2 mg of iodine; 0.2 mg of selenium; 2Mineral vitamin supplement (grower phase), each ton contains: 7,000 IU of vit. A; 2,000 IU of vit. D3; 11.0 mg vit. E; 1.6 mg vit. K3; 1.6 mg vit. B1; 4.5 mg vit. B2; 2.2 mg vit. B6; 10.0 mcg vit. B12; 32.0 mg of niacin; 12.0 mg of pantothenic acid; 0.8 mg of folic acid; 10.0 mg of copper; 50.0 mg of iron; 80.0 mg of manganese; 60.0 mg of zinc; 1.2 mg of iodine; 0.2 mg of selenium.
FTU- Its activity in FTU is defined as the amount of enzyme that releases 1 μmol of inorganic phosphorus/min from 0.0051 mol/L of sodium phytate at pH 5.5 and 37 ºC.
*Coxistac PX 12% e MNGrow.
Table 3 Composition and calculated nutritional values of the diets according to the treatments and the growth phases of Experiment II.
Ingredients (%) | Positive control | Enzyme X | Enzyme Y | Negative control | ||||
---|---|---|---|---|---|---|---|---|
1 to 21 | 22 to 35 | 1 to 21 | 22 to 35 | 1 to 21 | 22 to 35 | 1 to 21 | 22 to 35 | |
Corn 7.26% CP | 58.70 | 62.60 | 59.70 | 63.70 | 597.0 | 63.70 | 59.70 | 63.70 |
Soybean meal 47% CP | 32.80 | 28.60 | 33.80 | 29.60 | 33.80 | 29.60 | 33.80 | 29.60 |
MBM 41.86% CP | 3.50 | 2.80 | 1.70 | 1.00 | 1.70 | 1.00 | 1.70 | 1.00 |
Soybean oil | 2.90 | 3.80 | 2.30 | 3.10 | 2.30 | 3.10 | 2.30 | 3.10 |
Limestone 37% Ca | 0.84 | 0.10 | 0.12 | 0.14 | 012 | 0.14 | 0.12 | 0.14 |
Common salt | 0.42 | 0.40 | 0.44 | 0.42 | 0.44 | 0.42 | 0.44 | 0.42 |
DL-Methionine 99% | 0.28 | 0.24 | 0.27 | 0.23 | 0.27 | 0.23 | 0.27 | 0.23 |
L-Lysine 98% | 0.16 | 0.17 | 0.15 | 0.16 | 0.15 | 0.16 | 0.15 | 0.16 |
Mineral vitamin supplement1, 2 | 0.20 | 0.20 | 0.20 | 0.20 | 0.20 | 0.20 | 0.20 | 0.20 |
L-Threonine | 0.08 | 0.07 | 0.06 | 0.05 | 0.06 | 0.05 | 0.06 | 0.05 |
Choline chloride 70% | 0.05 | 0.04 | 0.05 | 0.04 | 0.05 | 0.04 | 0.05 | 0.04 |
Salinomycin* | - | 0.05 | - | 0.05 | - | 0.05 | - | 0.05 |
Nicarbazine+ | ||||||||
Maduracimin* | 0.05 | - | 0.05 | - | 0.05 | - | 0.05 | - |
Halquinol 60% | 0.005 | 0.005 | 0.005 | 0.005 | 0.005 | 0.005 | 0.005 | 0.005 |
Enzyme X (5,000 FTU 100 g/T) | - | - | 0.1 | 0.1 | - | - | - | - |
Enzyme Y (10,000 FTU 50 g/T) | - | - | - | - | 0.05 | 0.05 | - | - |
Chemical composition (calculated on natural matter basis) | ||||||||
Met. Energy Kcal/kg | 3,049.0 | 3,146.0 | 3,049.0 | 3,146.0 | 3,049.0 | 3,146.0 | 3,016.0 | 3,113.0 |
Crude Protein% | 21.5 | 19.5 | 21.5 | 19.5 | 21.5 | 19.5 | 21.2 | 19.2 |
Calcium% | 0.96 | 0.91 | 0.96 | 0.91 | 0.96 | 0.91 | 0.86 | 0.81 |
Available phosphorus% | 0.35 | 0.30 | 0.35 | 0.30 | 0.35 | 0.30 | 0.25 | 0.20 |
Lysine (digestible)% | 1.17 | 1.06 | 1.17 | 1.06 | 1.17 | 1.06 | 1.16 | 1.05 |
Methionine (digestible)% | 0.59 | 0.53 | 0.58 | 0.52 | 0.58 | 0.52 | 0.58 | 0.52 |
Met + Cystine% | 0.85 | 0.77 | 0.85 | 0.77 | 0.84 | 0.77 | 0.85 | 0.77 |
MBM - meat bone and meal; CP - Crude Protein; 1Mineral vitamin supplement (initial phase), each ton contains: 9,000 IU vit. A; 2,500 IU vit. D3; 14.0 mg vit. E; 2.0 mg vit. K3; 2.5 mg vit. B1; 6.2 mg vit. B2; 4.0 mg vit. B6; 14.0 mcg vit. B12; 40.0 mg niacin; 15.0 mg pantothenic acid; 1.0 mg folic acid; 10.0 mg copper; 50.0 mg iron; 80.0 mg manganese; 60.0 mg zinc; 1.2 mg iodine; 0.2 mg selenium; 2Mineral vitamin supplement (grower phase), each ton contains: 7,000 IU vit. A; 2,000 IU vit. D3; 11.0 mg vit. E; 1.6 mg vit. K3; 1.6 mg vit. B1; 4.5 mg vit. B2; 2.2 mg vit. B6; 10.0 mcg vit. B12; 32.0 mg niacin; 12.0 mg pantothenic acid; 0.8 mg folic acid; 10.0 mg copper; 50.0 mg iron; 80.0 mg manganese; 60.0 mg zinc; 1.2 mg iodine; 0.2 mg selenium.
FTU- Its activity in FTU is defined as the amount of enzyme that releases 1 μmol of inorganic phosphorus/min from 0.0051 mol/L of sodium phytate at pH 5.5 and 37 ºC.
* Coxistac PX 12% e MNGrow.
Performance variables (body weight gain (BWG), feed intake (FI), feed conversion ratio (FCR) and productive viability) were evaluated at seven and 35 days of age by recording the weight of the birds, feed supply and surplus on a weekly basis, and the number and weight of birds slaughtered daily.
At 35 days of age, two birds from each replicate -for a total of 12 birds per treatment- were euthanized by cervical dislocation to collect the left and right tibia. Lipid extraction was carried out by immersion in petroleum ether to determine ash percentage in the bones. Ash content was then obtained by calcinating bones in a muffle furnace at 600 ºC for six hours and then weighing the sample. Bone ash was used to make a standard solution to determine the percentage of phosphorus according to AOAC, 2005.
The Seedor index was determined by measuring the greatest length of the tibia and dividing the value by its weight, which was obtained using a semi-analytical digital scale (Seedor, 1991). For histopathological analyses, bone tissue samples were previously fixed in 10% buffered formalin for 48 hours and then maintained in 24% formic acid for 15 days for descaling. To prepare slides, the tissues were dehydrated in an increasing series of ethanol, diaphanized in xylol and included in paraffin to obtain 4-µm serial sections. The sections were stained using the Hematoxylin-Eosin (HE) technique and analyzed under light microscopy with a Binocular L1000-b-ac optical microscope (Bioval, Curitiba, PR, Brazil).
Bone mineral density (g/cm3) was measured in the tibia with a DPX-ALPHA densitometer (Alpha, São Paulo, SP, Brazil). This analysis produced values of bone mineral content (BMC) and bone area, from which bone mineral density (BMD) was obtained by dividing BMC by bone area, since density is the result of the mass/volume ratio. The same bones measured with the densitometer were subjected to a mechanical testing using a universal EMIC test machine (DL 3000 model) (EMIC Equipments and Test Systems Ltda, São José dos Pinhais, PR, Brazil) with load applied at a speed of 5 mm/min and load cell of 2000N in a three-point bending test. The central region of the bone (diaphysis) was selected for application of the load.
The results of the biomechanical analysis were recorded by Instron Series IX software as a graphic of the load vs deformation curve. The analysis of these curves provided bone maximum strength (BMS), bone resilience (BR) and bone rigidity (BRi) for each treatment.
Results
In Experiment I, feed intake and feed conversion during day one to seven of age were not influenced by the treatment (p>0.05) (Table 4). However, the body weight gain of broilers fed the negative control diet was lower than that of those fed the positive control diet or those supplemented with phytase X (p=0.005), and similar to that of birds fed a diet containing phytase Y (p>0.05).
In the period from one to 35 days of age, feed intake and viability were similar among all treatments (p>0.05) (Table 4). However, lower weight gain was observed in broilers fed the diet supplemented with phytase Y or receiving the negative control diet compared to those receiving the positive control (p<0.05). Feed conversion was not influenced by the inclusion of phytases in the diet (p>0.05); however, chickens fed the positive control diet had better feed conversion than those fed the negative control diet (p<0.05) (Table 4).
Table 4 Effect of phytase supplementation in the diet with normal phosphorus levels on growth performance of broiler chicks.
1 to 7 d of age | |||||
---|---|---|---|---|---|
Treatment | Initial body weigth (g) | Feed intake (g) | Body weigth gain (g) | Final body weigth (g) | Feed conversion ratio |
Positive control | 42.93 | 186.40 | 163.53a | 206.46a | 1.142 |
Phytase X | 43.56 | 189.57 | 167.86a | 211.42a | 1.131 |
Phytase Y | 42.76 | 186.11 | 162.31a, b | 205.07a, b | 1.153 |
Negative control | 43.25 | 186.16 | 156.80b | 200.05b | 1.191 |
SEM | 0.163 | 1.31 | 1.46 | 1.40 | 0.005 |
P-value | 0.330 | 0.453 | 0.005 | 0.005 | 0.128 |
1 to 35 d of age | |||||
Feed intake (g) | Body weigth gain (g) | Final body weight (g) | Feed conversion ratio | Viability (%) | |
Positive control | 3,782.23 | 2,557.86a | 2,600.79ª | 1.482a | 95.8 |
Phytase X | 3,756.01 | 2,494.00a, b | 2,537.56ª,b | 1.511a, b | 94.2 |
Phytase Y | 3,730.26 | 2,470.27b | 2,513.03b | 1.512a, b | 94.6 |
Negative control | 3,730.64 | 2,471.73b | 2,514,98b | 1.514b | 94.0 |
SEM | 15.20 | 13.20 | 12.40 | 0.004 | 0.52 |
P-value | 0.334 | 0.005 | 0.005 | 0.008 | 0.683 |
Values (average ± SEM) with no common superscript letters (a, b) within columns are different by Tukey’s test (p<0.05). SEM (Standard Error of the Mean).
Bone quality analysis of broiler chicks at day 35 of Experiment I revealed that the treatments had no effect (p>0.05) on bone mineral content, bone area, bone mineral density, Seedor index, maximum strength endured before bone breakage, bone ash and percentage of phosphorus in tibia (Table 5). For bone resilience, however, bones from positive control birds had higher values (p<0.05) than bones from negative control birds, the latter not differing from the bones of the other treatments. For bone rigidity, the bones from the phytase X treatment had better rigidity than bones from birds of the other treatments (p<0.05).
Table 5 Effect of phytase supplementation in the diet with normal phosphorus levels on bone quality of broilers at 35 days.
Treatment | BMC (g) | Area (cm3) | BMD (g/cm3) | SI | BMS (N) | BR (mJ) | BRi (Mpa) | BA (%) | P (%) |
---|---|---|---|---|---|---|---|---|---|
Positive control | 2.41 | 11.56 | 0.21 | 2.39 | 377.68 | 892.80a | 105.03b | 43.98 | 7.12 |
Phytase X | 2.56 | 11.66 | 0.22 | 2.51 | 380.80 | 800.60a,b | 165.32a | 44.97 | 7.44 |
Phytase Y | 2.67 | 12.14 | 0.22 | 2.17 | 365.93 | 868.17a,b | 109.31b | 45.15 | 8.01 |
Negative control | 2.28 | 11.82 | 0.19 | 2.42 | 335.34 | 701.40b | 111.39b | 42.87 | 7.19 |
SEM | 0.08 | 0.16 | 0.005 | 0.09 | 16.80 | 40.40 | 7.16 | 0.56 | 0.20 |
P-value | 0.744 | 0.846 | 0.066 | 0.331 | 0.168 | 0.029 | 0.003 | 0.071 | 0.234 |
Values (average ± SEM) with no common superscript letters (a, b) within columns are different by Tukey’s test (p<0.05). Bone mineral content (BMC), bone area (Area), bone mineral density (BMD), Seedor index (SI), maximum strength endured before bone breaking (BMS), bone resilience (BR), bone rigidity (BRi), bone ashes (BA) and percentage of phosphorus in the tibia (P). SEM (Standard Error of the Mean).
In Experiment II, during the period from one to seven days of age broilers fed the positive control diet or diet supplemented with phytase X had higher feed intake than broilers fed the diet containing phytase Y or fed the negative control diet (p<0.001) (Table 6).
Weight gain in the first week was influenced by treatment (p<0.001), with the greatest weight gain observed in chickens receiving the positive control feed or feed supplemented with phytase X, followed by chickens that received feed containing phytase Y, and finally those fed the negative control diet. Feed conversion was not influenced by treatment (p<0.05) (Table 6).
In the period from one to 35 days of age, feed intake, weight gain and viability were influenced by treatment (p<0.05) (Table 6). Broilers receiving feed supplemented with phytase X had feed intake similar to chickens fed the positive control diet (p>0.05), and both had higher feed intake compared to the group receiving feed with phytase Y or feed negative control (p<0.05). The negative control had lower viability than the positive control and phytase X treatment (p<0.05).
Table 6 Effect of phytase supplementation in the diet with reduced phosphorus levels on growth performance of broilers chicks.
1 to 7 d of age | |||||
---|---|---|---|---|---|
Treatment | Initial body weigth (g) | Feed intake (g) | Body weigth gain (g) | Final body weigth (g) | Feed conversion ratio |
Positive control | 42.7 | 177.3a | 154.9a | 197.6a | 1.145 |
Phytase X | 42.1 | 181.0a | 155.1a | 197.2a | 1.174 |
Phytase Y | 42.9 | 166.9b | 145.7b | 188.6b | 1.147 |
Negative control | 42.7 | 158.2b | 134.0c | 176.7c | 1.181 |
SEM | 0.15 | 2.05 | 2.22 | 2.02 | 0.009 |
P-value | 0.987 | <0.001 | <0.001 | <0.001 | 0.066 |
1 to 35 d of age | |||||
Treatment | Feed intake (g) | Body weigth gain (g) | Final body weigth (g) | Feed conversion ratio | Viability (%) |
Positive control | 3,608.0a | 2,429.8a | 2,472.5ª | 1.486 | 91.4a |
Phytase X | 3,593.1a | 2,399.9a | 2,442.0a | 1.498 | 89.9a, b |
Phytase Y | 3,347.3b | 2,239.1b | 2,282.0b | 1.494 | 83.5b, c |
Negative control | 2,889.0c | 1,915.3c | 1,958.0c | 1.510 | 76.7c |
SEM | 62.82 | 44.05 | 42.01 | 0.005 | 1.53 |
P-value | <0.001 | <0.001 | <0.001 | 0.938 | <0.001 |
Values (average ± SEM) with no common superscript letters (a, b) within columns are different by Tukey’s test (p<0.05). SEM (Standard Error of the Mean).
Bone quality analysis of broilers at day 35 of Experiment II (Table 7) revealed that the treatments had no effect on bone density, Seedor index, maximum strength endured before bone breakage and BR (p>0.05). However, mineral content and total bone area differed between the phytase X treatment and the negative control (p<0.05), with the former having greater mineral content and total bone area. Percentage of bone ash and phosphorus were lower in the negative control group compared to the other treatments (p<0.05).
Table 7 Effect of phytase supplementation in the diet with reduced phosphorus levels on bone quality of broilers at 35 days.
Treatment | BMC (g) | Area (cm3) | BMD (g/cm3) | SI | BMS (N) | BR (mJ) | BRi (Mpa) | BA (%) | P (%) |
---|---|---|---|---|---|---|---|---|---|
Positive control | 1.5a, b | 11.6a, b | 0.13 | 2.13 | 348.5 | 894.6 | 99.5b | 42.4a | 7.22a |
Phytase X | 2.2a | 12.5a | 0.17 | 2.30 | 422.3 | 1018.6 | 145.8a | 45.6a | 7.42a |
Phytase Y | 1.6a, b | 12.2a, b | 0.13 | 2.38 | 344.1 | 912.6 | 122.7a, b | 43.2a | 7.51a |
Negative control | 1.2b | 10.57b | 0,11 | 2.34 | 292.7 | 685.6 | 151.5a | 36.8b | 5.48b |
SEM | 0.114 | 0.255 | 0.008 | 0.08 | 16.90 | 44.30 | 6.46 | 0.872 | 0.56 |
P-value | 0.038 | 0.020 | 0.063 | 0.089 | 0.072 | 0.321 | 0.016 | <0.01 | <0.01 |
Values (average ± SEM) with no common superscript letters (a, b) within columns are different by Tukey’s test (p<0.05). Bone mineral content (BMC), bone area (Area), bone mineral density (BMD), Seedor index (SI), maximum strength endured before bone breaking (BMS), bone resilience (BR), bone rigidity (BRi), bone ashes (BA) and percentage of phosphorus in the tibia (P). SEM (Standard Error of the Mean).
Results of the histopathological analysis of the bones in both experiments showed no effect of treatment on bone formation; bones presented regular growth plate and vascularization in the region of the metaphysis (Figure 1).
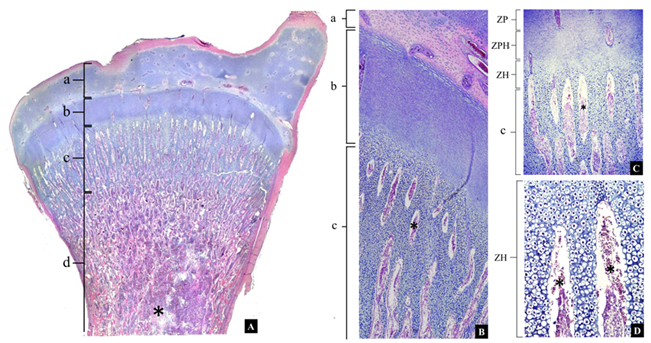
Figure 1 Broiler chicken, normal proximal tibiotarsus. Photos (microscopy with Binocular L1000-b-ac optical microscope -Bioval, Curitiba, PR, Brazil-) A, B and C refer to NCII treatment at the bird’s 35th day of age. In A, it can be observed epiphysis (a), growth plate or physis (b) and metaphysis (c). In B and C, the zones of the growth plate (b) are observed: proliferation zone (ZP), prehypertrophic zone (ZPH) and hypertrophic zone (ZH). The metaphysis vessels (*) can be seen in all images, occasionally invading the hypertrophic zone of the growth plate.
Discussion
In Experiment I, performance results for the two phases (starter and grower) showed that the positive control group was superior to the negative control group for weight gain, even though they had the same feed intake. This finding can be explained by the physiological functions of phosphorus, since it is an essential mineral for growth and structural and metabolic development of broilers. According to Adedokun and Adeola (2013), about 80.0% of ingested P is associated with calcium in bone formation, so it was expected that P would be involved in several stages of broiler life. The negative control group had reduced growth in the starter phase; although P requirement is lower in this period and increases throughout the growth and final stages (Vargas Jr et al., 2003). Demand for P increases with chick growth, while weight gain and feed conversion during days 1-35 were worse for the negative in relation to the positive control. Several studies have shown that phytase addition to broiler diets improves P digestibility and reduces its excretion (Ahmad et al., 2000, Scholey et al., 2018, Leyva-Jimenez et al., 2018) whether or not birds receive reduced dietary levels of P.
In Experiment I, with normal phosphorus, final body weight and feed conversion of phytases X and Y treatments were similar to the negative control group. These can be explained by a variation in the efficiency of phytase activity in the digestive tract of broilers. According to Dersjant-Li et al., (2015), factors such as pH, endogenous protease resistance, dietary phytate levels, feed processing, Ca and Na content, age and intestinal health of birds can affect phytase activity.
In Experiment II, Phytase X results for weight gain in the initial phase (1-7 d) were similar to the positive control and superior to Phytase Y treatment. Thus, although both enzymes can be extracted from the same microorganism, used in the same concentration they can produce different results under reduced levels of dietary P. Leyva-Jimenez et al. (2018) also found differences between enzyme results. The different efficiency between phytases does not necessarily mean one is superior to the other, but may be related to dosage, since both were included at 500 FTU/kg; at a higher dosage it is possible that the enzymes have similar result (Walters et al. 2019; Dersjant-Li et al. 2021; Sens et al. 2021). Accordingly, inclusion levels of these enzymes should be studied, evaluating their efficiency. Still, according to Araújo et al. (2008), mineral levels in diets for broilers are often higher than the minimum required, which represents a waste of ingredients and, consequently, elevated cost in broiler production.
According to the feed conversion ratio at 35 days, phytases for broiler chickens can improve the use of phosphorus and guarantee better performance. Scholey et al. (2018) reinforced the need for broiler chickens to use P for their bone growth. Regarding bone quality in Experiment I, the positive control performed better than the negative control and was similar to the two enzyme treatments. For example, BR -which measures the resistance of bone to tension and then return to its natural state- was similar between the positive control and the Phytase X treatment, and superior to the negative control.
In Experiment II, broilers revealed sensitivity to reduced phosphorus levels. The negative control and Phytase Y treatment showed worse performance and bone quality (0.25 mg P) compared to the positive control and Phytase X treatment. Feed consumption was lower for the negative control, which is in agreement with Persia and Saylor (2006), who found that increased AP in the diet increases feed consumption. Regarding performance, Leandro et al. (2006) stated that chick development in the first week is determinant for weight at 42 days of age. Thus, the low growth of chicks in the initial phase may have been responsible for the low performance observed at 35 days, since the birds did not grow and showed less uniformity. According to Nääs et al. (2009), low growth of broiler chickens can reflect behavioral changes, such as not feeding and/or drinking water due to lack of access to feeders and drinkers, which would reduce performance and increase the number of injured carcasses, thereby reducing viability. Milica et al. (2012) observed that reducing dietary AP from 0.43% to as low as 0.10%, both with and without the use of phytase during the final period of growth resulted in increased mortality. They also observed greater efficacy of phytase in diets with reduced phosphorus. It is important to note that when phosphorus levels were reduced in Experiment II from 0.45 to 0.35% AP, a loss in performance was observed, characterized by reduced BWG and FI (P<0.05) regardless of the use of phytase, which shows that the levels of AP used in Experiment I are more adequate for broilers.
Thus, during the initial phase in Experiment II the viability of birds in the negative control was compromised because they did not have good bone and body development due to the low phosphorus levels of the diet and died during the breeding period because they could not reach the drinkers. Studying the inclusion of different phytases in broilers, Wu et al. (2015) found no differences for poultry viability during the period of 1-21 days; however, P levels were not reduced. Moreover, our performance results also differed from the findings of Brunelli et al. (2012), who did not find differences between groups supplemented or not with phytase; however, they did not include the value of the enzyme in the nutritional matrix and used the enzyme without reducing AP. On the other hand, Laurentiz et al. (2009) also observed a decrease in weight gain during the final period of rearing when P levels were reduced without the use of phytases. Thus, it can be concluded that a deficiency of AP for broiler chickens compromises growth performance, and the use of phytases can help increase AP.
These results are in accordance with Cardoso Júnior et al. (2010), who found that it was possible to reduce AP by 0.15% and Ca by 0.30% in relation to control feed without impairing performance of broilers during the growth phase when the feed was supplemented with phytase. Santos et al. (2011) also found that Ca and AP levels can be reduced to 0.52 and 0.26%, respectively, during the growth phase, and to 0.56 and 0.28%, respectively, during the final phase, if such a reduction is combined with phytase supplementation at the level of 500 FTU/kg of feed. Souza et al. (2015) also concluded that the use of phytase improves bird performance for diets with reduced levels of Ca and AP. They observed that Ca reduction in diets supplemented with phytase should be proportional to the reduction of P in order to maintain the proper Ca:P ratio since inadequate levels of Ca may impair the absorption of other minerals, such as P, Zn and Mg.
According to Pereira et al. (2012), bone ash content is the best parameter for estimating the amount of AP provided by phytase in diets based on corn and soybean meal. Thus, the amount of AP in the negative control diet was insufficient, with about 2.4% less P retention in tibias in relation to the positive control and the chickens of the phytase treatments. Thus, the use of phytases compensated for the low dietary level of P and increased P retention in tibias, as observed in P content and total ash. According to Scholey et al. (2018), the use of low phosphorus during the starter phase causes low mineral deposition, which promotes negative consequences for bone quality and bird performance. In the present study, dietary P reduction was sufficiently compensated for in the later stages of rearing, which can be attributed to the use of phytase, compensating for the AP deficiency and improving bone health and performance. Lelis et al. (2010), reported that phytase supplementation (250 and 500 FTU/kg feed) increased P retention in tibia when the nutritional matrix of the enzyme was considered.
Bone health is very important during rearing, capture, transportation to slaughter, and slaughter of broilers (Zhang et al., 2017). The fact that the rate of muscle growth outpaces that of bone growth increases the probability of leg problems for chickens (Almeida Paz et al., 2009). Despite compromising some bone quality variables of broilers, mainly due to low level of AP in the diet, the worst performance is not always associated with poorer bone quality.
Histopathological bone results from both experiments showed that treatment had no effect on bone formation, because they had bones with a regular growth plate and vascularization in the metaphysis region (Figure 1). According to Zhang et al. (2017), one of the main bone problems for broiler chickens is the lack of cartilaginous mineralization in the metaphysis of long bones. These problems are due to low vascularization in the metaphysis, which consequently inhibits calcification of the cartilage. Such low mineralization could result in increased frequency of carcass condemnations of broilers.
In view of the results presented herein, broiler chickens, even with normal bone growth, may present apparent problems with bone health. According to Wilson (2010), bruises and fractures among broiler chickens usually occur during capture, transport, discharge and hanging of birds, and can be attributed to bone fragility associated with management errors. Partial condemnations for bruises are the most common condemnations in slaughterhouses, and are due to pre-slaughter management problems (Almeida et al., 2018). Thus, the results of the present study support future studies to evaluate the effect of increased P availability and bone health on traumatic lesions of broiler chickens. Such research is particularly relevant for the final pre-slaughter management phase and the slaughtering process, since bone quality problems may result in increased carcass condemnations.
Therefore, the AP level in broiler feed must be sufficient for optimal performance and bone development. Broiler bones must be strong so that the carcass goes through the slaughter process without bones breaking. The poultry industry is concerned with problems related to bone integrity and resistance, especially because poorly developed and fractured carcasses lead to high discard rates at slaughterhouses.
In conclusion, reduced AP without the use of phytase reduces body weight gain and feed intake of broilers in addition to altering bone parameters, such as phosphorus concentration in the tibia. The efficiency of the commercial phytases studied differed, with Phytase X being more efficient.