Introduction
The CaTiO3 is a perovskite with a basic cubic structure present in a wide variety of materials, where A is an element of the representative metal group (IIA) and B is a transition metal (IVB) [1]. The properties of this system are governed by the elements A and B, which could be modified by means the synthesis methods through of substitution, doping or modification. Special attention deserves the substitutions made with elements of the rare earth series, achieving promising physicochemical properties due to the chemical stability related with these atoms, creating a series of distortions in the lattice which are reflected in the magnetic properties and electronic conduction levels [2]. On the other hand, although the solid state reaction method has been adequate to obtain this type of systems, starting from corresponding high purity oxides with excellent results at compositional and structural level, some drawbacks are important due to impurity generated by the low kinetic reaction related with the solid state at high temperatures [3]. For this reason, with the aim of reduce these adverse effects, some authors evaluate the possibility of increase the magnetic response of these oxides by means the incorporation of Dy, Ho, and Gd which make these systems were effective in various technological applications, such as magnetic and optoelectronic devices, described and proposed in literature [4]. Although the incorporation of rare earth in systems derived from CaTi1-xMxO3 system, represent a great interest in several nanotechnology areas for the manufacture of advanced equipment and opto-electronic materials in the automotive and biomedical industry, is clear that the electronic configuration 4fn of these atoms, is the main responsible of spin-electron interactions in an electric field, this phenomenon can be controlled by modifications of the cations of CaTiO3, which is widely described in the literature, generating new developments in materials science [1,5]. This work focuses on the synthesis, structural and magnetic characterization of the CaTi1-xMxO3 system, where M = Dy. Ho, Gd with a degree of substitution of x = 0.5 by the solid-state reaction method, with the purpose of evaluate the effect of ionic radii on the stability and properties of the structure.
Methodology
The synthesis of the CaTi0.5M0.5O3 (M = Dy, Ho, Gd) perovskite, starting from corresponding metal oxides of CaO, TiO2, Dy2O3, Ho2O3 and Gd2O3 99.9 % all from Sigma-Aldrich. In all cases, the solids were grounded in an agate mortar for 4 hours, to promote a high degree of homogenization. The solids were identified as follows: CaTi0.5Dy0.5O3 (CTDO), CaTi0.5Ho0.5O3 (CTHO) and CaTi0.5Gd0.5O3 (CTGO), which were pressed in a pellet form using hydrostatic press operated at 2.0 MPa. The pellets were treated at 1273.15 K for 24 hours and sintering at 1423.15 K for 24 hours respectively in air atmosphere. The structural parameters were evaluated by X-ray diffraction in a PANalytical X'Pert diffractometer with Cu K α radiation (λ = 1.54x10-10 m), between 20° to 90°. The analysis of the diffraction patterns were done through the Rietveld routine using the GSAS software. The magnetization measurements based on the applied magnetic field and temperature were carried out on a Versalab vibrating sample magnetometer (VSM), with temperatures between 50 K and 300 K and magnetic fields between -30 kOe and 30 kOe.
Results and discussion
From the X-ray diffraction analysis shown in Figure 1, is clear that the CaTi05M05O3 system (M = Dy, Ho, Gd) is present in an orthorhombic structure with a spatial group Pbnm (62) which constitutes the main crystalline phase. The average grain size domains indicate values around 3.5x10-8 -3.7x10-8 m for the obtained materials, which were calculated using the Debye-Scherrer equation from the highest diffraction signals [6].
The analysis of the lattice parameters and crystalline phases based on the Rietveld refinement method shown in figure 2, show that the modification of CaTiO3 with Dy, Ho and Gd was effective and the presence of secondary phases is insignificant [7,8]. Two impurities were identified in each system, corresponding with rare earth oxides, which are identified with the (♦) and (♠) symbols for the CaO and RE2O3 respectively. In the case of CTDO system the solid shown an I a-3 (206) spatial group while in the CTHO and CTGO systems was clear a classification according with Fm-3m (225) spatial group respectively. These structures were indexed from corresponding crystallographic data and summarized in the Tables 1-3.
Table 1 Lattice parameters and crystalline phase data derived from Rietveld refinement method for the CTDO system.
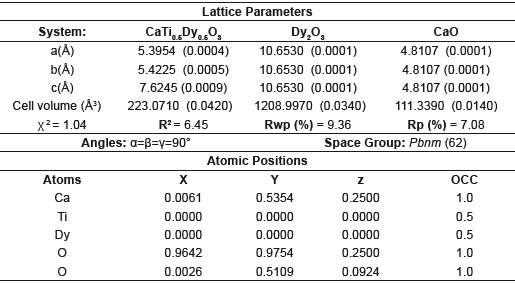
Table 2 Lattice parameters and crystalline phase data derived from Rietveld refinement method for the CHDO system.
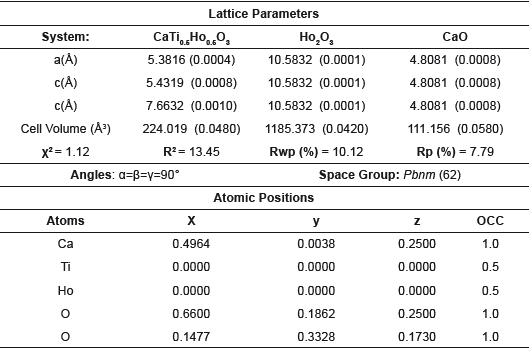
Table 3 Lattice parameters and crystalline phase data derived from Rietveld refinement method for the CGDO system.
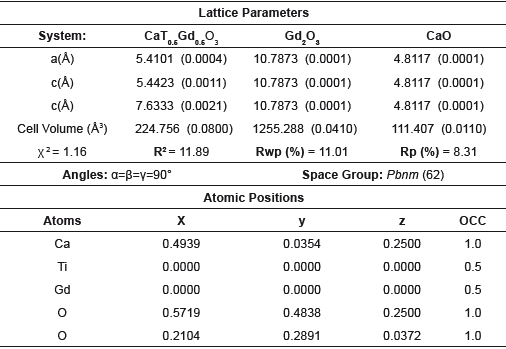
The magnetic characterization as a function of the temperature for all samples, suggest that the different modifications exhibit a paramagnetic behavior (M vs T), due to the presence of unpaired electrons in the 4fn orbital in Dy, Ho and Gd cations, whose electrons suffer an orientation process along magnetic field direction (a) (figures 3-5).Through the hysteresis curve (M vs H), this paramagnetic behavior is corroborated by a linear trend in figure (b).
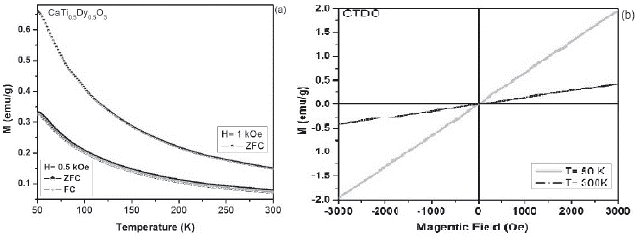
Figure 3 a. ZFC-FC magnetization curves at 1 kOe and 0.5 kOe for the CaTi05Dy05O3 sample. b. M-H curves of CTDO system at 50 K and 300K.
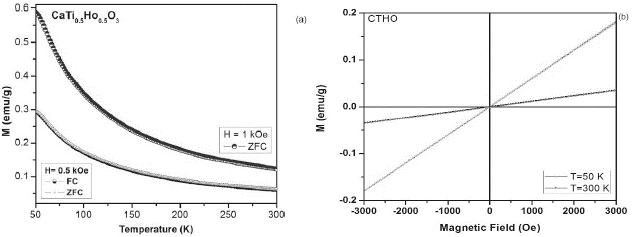
Figure 4 a. ZFC-FC magnetization curves at 1 kOe and 0.5 kOe for the CaTi05Ho05O3 sample. b. M-H curves of CTHO system at 50 K and 300K.
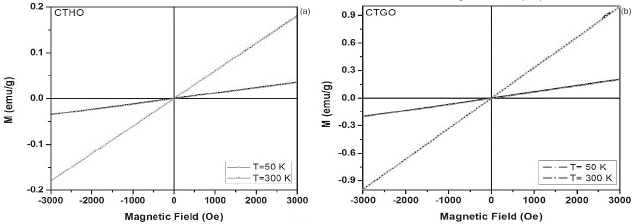
Figure 5 a. Magnetization vs temperature (ZFC-FC) at 1 kOe and 0.5 kOe for the CaTi05Gd05O3 sample. b. M-H curves of CTGO system at 50 K and 300K.
Such results, corroborate a cationic distortion of Ti3+ and RE3+ cations, mainly provoked for the electronic interaction given by the metal occupation, due to the doping level (x = 0.5) used, which induces the modification of the structure and its magnetic character by modification bond distances between cations which results in the appearance of defects or dislocations and oxygen vacancies, when rare earths replace site Ti3+, forming octahedral coordination [2,9,10,11].
In systems developed from manganites such as CaMnO3, modified with Mo, this behavior can be observed in the form of paramagnetic-antiferromagnetic transition in substitutions of x = 0.1 at temperatures around 150K. [12]. Similarly, it is produced by modifying rare earth systems, such as the cerium oxide system modified with Praseodymium Ce0.6Pr0.4O2, whose paramagnetic behavior increases as the temperature is reduced T ≤ 150K [13]. The above results are attributed to the sintering temperature of 1423,15K which gives it some degree of distribution to the material in terms of its structural and magnetic characteristics, which is evidenced in Mn-doped CaTiO3 systems, which at a temperature of 1473,15K Has an antiferromagnetic behavior [5].
Conclusions
The incorporation of rare earths in the CaTi0.5M0.5O3 (M = Dy, Ho, Gd) system, synthesized by the standard solid state method, gives the material an orthorhombic crystal structure with a space group Pbnm (62) and Paramagnetic character given by the measurements of magnetization as a function of temperature and applied magnetic field, due to the ionic radius of the rare earth, observed in a range of 0.97 (Å) to 1.02 (Å) of these elements, which interact from such way with the titanium, giving rise to vacancies of oxygen that allow the distortion of the structural parameters; Heat treatment in this range of materials in terms of their structural and magnetic properties is also an influential factor given by order and ionic interaction at the electronic level.