Introduction
Water for agriculture is critical for future global food security, because agriculture constitutes the largest user of fresh water, with irrigation withdrawals representing approximately 70% of total water use (Knox et al., 2012). However, only half of this, is estimated to reach the intended crop, the rest being lost somewhere between the point of extraction and the crop (Turral et al., 2010). The significant quantity of water used by agriculture for production raises the question as to whether agriculture could become more efficient in its water use (Hatfield, 2015), on the fact that most fresh water is stored as glaciers or deep groundwater (Oki & Kanae, 2006). Furthermore, global warming threatens to exacerbate the situation with a predicted reduction in water resources. In the Yucatan Peninsula, an increase in the drought severity index is expected (Orellana et al., 2009), which could have a direct effect on crops. Habanero pepper (Capsicum chinense Jacq.) is the most important crop in the Yucatan Peninsula. As the hottest pepper, it represents one of the major spices traded on international markets due to its typical burning sensation, known as pungency, caused by the presence of capsaicinoids (Garruña-Hernández et al., 2013). However, few studies of water use are available on this species. Quintal-Ortiz et al. (2012), applied five irrigation levels in pots with drainage and concluded that an irrigation level (IL) of 60% of available water capacity (AWC) of substrate increased both plant growth and fruit size. It is not clear in that study, was all the water used in evapotranspiration? What would happen when using pots without drainage? Movement of water throughout soil profile is known to occur when the amount of water exceeds the soil storage capacity and water moves downward under gravitational force. Respectively, in the search for a better water use efficiency, the aim of this research was to identify the best irrigation level for Habanero pepper plants based on AWC of substrate.
Material and methods
Plant material
The study was conducted at the Instituto Tecnológico de Conkal, Yucatan, Mexico. Habanero pepper (Capsicum chinense Jacq.) seeds from five genotypes (4 landrace: H225, H241, H244, H226; and 1 commercial: Jaguar) were germinated in 200-well polystyrene trays in a peat moss substrate. After 45 days, the seedlings were transplanted to 6 Kg pots (without drainage) containing a soil-poultry manure-henequen bagasse mix (2:1:1 v/v) and placed in a greenhouse for the experimental phase. The environmental conditions of the greenhouse during all crop cycles were registered with a portable micro meteorological station (HOBO; Onset Computer Corporation Massachusetts, USA) (Figure 1).
Irrigation treatments
After transplanting, all irrigated plants at field capacity, were subject to homogenize the substrate, two days later the start of irrigation treatments. The field capacity (Өfc ) and permanent wilting point (Өwp ), were estimated with gravimetric method based on conventional oven-dry weight of substrate. To calculate the irrigation level, the equation of Wallender & Grimes (1991), was modified using Өfc -Өwp instead of AWC and pot depth instead of soil depth (Equation 1).
Where: IL = irrigation level (cm), Өfc = field capacity (%), Өwp = permanent wilting point (%), PD = pot depth (cm), BD = bulk density (g cm-3), WD = water density (g cm-3). To convert IL to water volume (WV) in cm3, IL, was multiplied per pot area (cm2). The water volume calculation to be applied per treatment (Equation 2).
Where: FIL = final irrigation level in volume (cm3) and IA = irrigation amount (in this research: 20, 40 or 60% of AWC).
Growth, phenology and water potential
When plants were in fruiting stage (150 days after sowing), the stem diameter and plant height, were measured with a digital vernier and measuring tape respectively. In order to obtain the leaf area and dry weight (matter), were evaluated accordingly to Garruña-Hernández et al. (2014).
The inflorescence stage was considered when more than half (50% + 1) of the plants in each treatment exhibited flowers (Garruña-Hernández et al., 2013). Soil water potential (Ψs) was estimated at 8:00 and 14:00 hours. 100 g of substrate in plastic bags, stored in a cooler with ice, carried to the laboratory, and then water potential measured with a WP4 dewpoint potentiometer (Decagon Devices, Washington, USA).
Chlorophyll fluorescence parameters
The maximum photochemical quantum yield of photosystem II (Fv/Fm) was measured with a port able pulse amplitude modulation fluorometer (PAM Walz, Effeltrich, Germany) at pre-dawn (5:00 h), where Fv is variable fluorescence (Fm - F0), F0 is initial fluorescence, and Fm maximum fluorescence. Fluorescence measurements performed on the third or fourth leaf from the shoot apex. Measurements performed at 145 days after sowing (100 days af ter starting the water deficit treatment). The saturation pulse intensity was 6000 µmol photons m-2 s-1 and the pulse length was 0.8 s. Light intensity during the measurement was 204 µmol photons m-2 s-1. Light response curves of the relative electron transport rate (ETRPSII) and the effective photochemical quantum yield of PSII (ΦPSII) measurement were obtained through the application of a series of nine saturation pulses under increasing actinic irradiance (PPFD) from 0 to 1500 µmol photons m-2 s-1, obtaining ETRPSII by equation 3.
Where PAR = Photosynthetic active radiation. ETR-Factor = Ratio of photons absorbed by photosynthetic pigments to incident photons (a value of 0.84 which matches the average absorptance in the visible range of many green leaves). PPS2/PPPS = Photons absorbed by PSII relative to photons absorbed by photosynthetic pigments (A value of 0.5 is reasonable if one assumes the presence of only linear electron transport and, hence, equal electron transport rates in PSI and PSII. The ΦPSII obtained by equation 4.
Where: Fm´ = Maximum fluorescence levels during a treatment is induced by saturating light pulse application, which closes all PSII reactions centers, and F´ = Is the level of the fluorescence curve during treatments and shortly before a saturating pulse is applied.
Experimental design
A randomized complete block design with bi-factorial arrangement was performed employing three irrigation levels (20, 40 and 60%) and five genotypes (H225, H241, H244, H226, Jaguar). Four replicates were established (15 plants each). Data were examined by two-way ANOVA and means were compared using Tukey's test at P ≤ 0.05 (Statistic Six Sigma, Release 7, StatSoft).
Results and discussion
The irrigation level (IL) affected early plant growth, the IL of 60% could not be evaluated because pots became flooded and plants did not survive. A previous study on IL in C. chinense (Quintal-Ortiz et al., 2012) concluded that 60% of AWC increased both plant growth and fruit size, but pots used for this study had drainage.
Irrigation treatments affected significantly plant growth (plant height, stem diameter, leaf area and dry matter). However, plant genotype did not have a significantly effect on plant growth parameters. Accordingly to González-Dugo et al. (2007), the pepper growth is closely linked to the water amount applied. Our results showed plant height of the jaguar genotype irrigated at 40%, was statistically similar to H241 (92±3.7 and 79±4.8 cm respectively) genotypes, but different to other treatments. Decreasing the IL from 40 to 20% produced a negative effect on plant height in both Jaguar and H241 genotypers (Figure 2A). The same occurred for stem diameter in all genotypes (Figure 2B). Similar responses were observed by Achten et al. (2010), who applied dry treatments to Jatropha curcas and showed there was a decrease in both stem length and diameter. In addition, dry matter was higher in the roots. However, in our study, there was a higher root dry weight in plants with high irrigation levels (Table 1). All genotypes had similar leaf area at an IL of 20%, but at an IL of 40%, leaf area of genotypes H225, H241 and H244 had at least 48% greater leaf area than Jaguar plants (Table 1). Similarly, there were no significantly differences in total dry matter among genotypes from an IL of 20%, but with an IL of 40% the total dry weight of H241 and Jaguar plants was 30 and 28% greater than plants of H226. Clearly, an increase in dry matter was produced by raising the IL (Table 1), such as occurs in tomatoes, where total dry matter accumulation is significantly depressed by soil water deficit (Patanè et al., 2011).
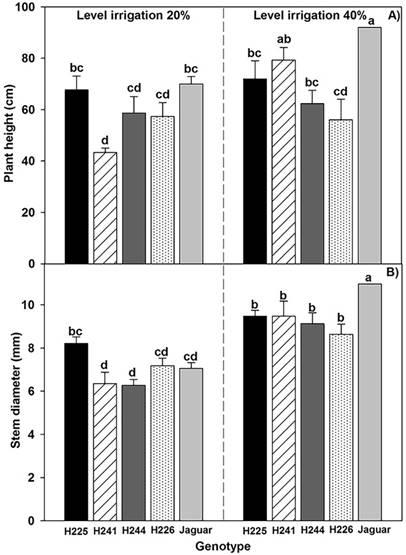
Figure 2 A) Plant height. B) Stem diameter of five habanero pepper genotypes at two irrigation levels (20% and 40%). Data are means ± SE. Different letters represent statistically significant differences (Tukey, =0.05).
In all genotypes, the inflorescence stage was earlier in plants with an IL of 20%, compared to an IL of 40% (Figure 3). This was a stress consequence due to water deficit. The degree of crop sensitivity to water deficit during the different developmental stages of a plant species is a significant characteristic to consider (Jaimez et al., 2000). Morales et al. (2013), found that raspberry under drought stress showed earlier inflorescence, while Ávila et al. (2012), observed that flowering was increased by water stress in Citrus. However, Jaimez et al. (2000), observed that low water availability delays the occurrence of maximum flowering in C. chinense. Our results showed the earlier inflorescence time was in response to water stress, H241, H244 and H226 genotypes, showed only two days of delay in flowering development under both irrigation treatments (Figure 3). Inflorescence time could be influenced by genotype.
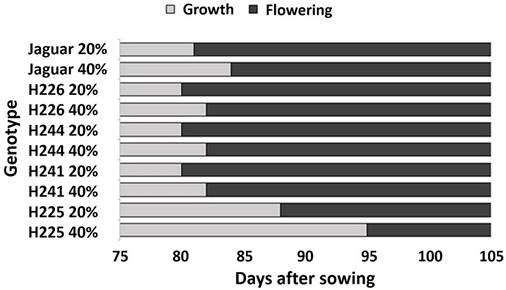
Figure 3 Flowering phenophase of five genotypes of Habanero pepper at two irrigation levels (20% and 40%). Data are means ± SE. Different letters represent statistically significant differences (Tukey, =0.05)
The soil water potential (Ψs) decreased from morning to afternoon, but it was lower in IL of 20% both at 8:00 (Figure 4A) and 14:00 h (Figure 4B). Therefore, water stress conditions were evident in the most restrictive treatment (IL of 20%).
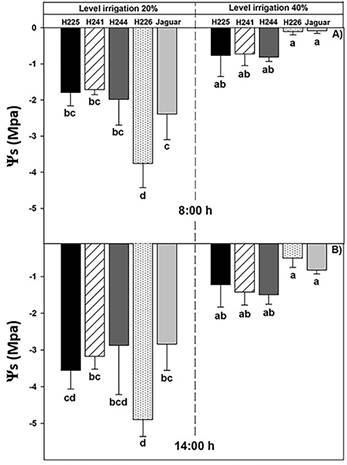
Figure 4 Soil water potential (Ψs) of five genotypes of habanero pepper at two irrigation levels (20% and 40%) at A) 8:00 and B) 14:00 hours. Data are means ± SE. Different letters represent statistically significant differences (Tukey, =0.05)
Regarding the chlorophyll (Chl) fluorescence parameters, the values of Fv/Fm reflect the potential quantum efficiency of PSII and are performed as a sensitive indicator of plant photosynthetic performance (Maxwell & Johnson, 2000). With the exception of genotype H225, Fv/Fm in leaves was higher in plants in an IL of 40% than in an IL of 20%. Plant leaves of H241 (0.81), H244 (0.83) and H226 (0.83) had higher Fv/Fm values. An Fv/Fm value of around 0.83 is an optimal measurement for most plant species (Maxwell & Johnson, 2000). Likewise, H244, H226 and H241 plants at an IL of 40% exhibited better performance both in terms of electron transport rate (ETR) and effective photochemical quantum yield of photosystem II (ΦPSII). Genotypes H225 and Jaguar therefore showed greater physiological susceptibility to the lack of water. Penella et al. (2014), did not find any differences among control and water stress treatments in the Fv/Fm of 18 pepper genotypes. However, Ogaya & Peñuelas (2003), showed drought treatment produced a decrease in Fv/Fm values of Quercus ilex and Parentucellia latifolia plants.
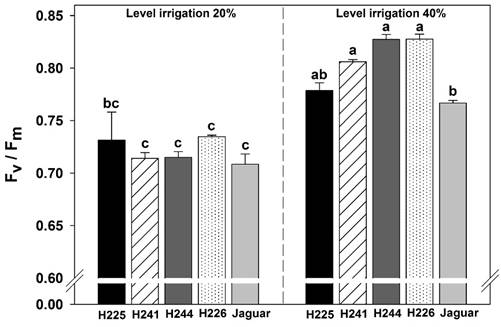
Figure 5 Maximum photochemical quantum yield of photosystem II (Fv/Fm) in five genotypes of Habanero pepper at two irrigation levels (20% and 40%). Data are means ± SE. Different letters represent statistically significant differences (Tukey, =0.05)
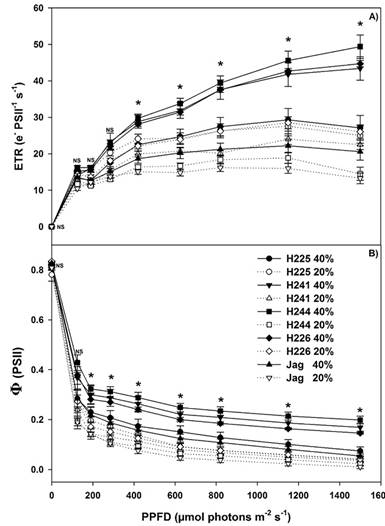
Figure 6 Response curves of A) Electron transport rate (ETR) and B) Effective photochemical quantum yield of photosystem II (ΦPSII) to photosynthetic photon flux density in five genotypes of Habanero pepper at two irrigation levels (20% and 40%). Data are means. NS: not significant. *: significant (ANOVA, P ≤ 0.05).
Moreover, drought treatment also produced a slight decrease in ETR values for both species, and the highest PSII Yield was in the season when no drought stress occurred (Ogaya & Peñuela, 2003). The effects of abiotic and biotic stresses on plant photosynthetic performance lead to fitness and yield decreases. The maximum quantum efficiency of photosystem II (Fv/Fm) is a parameter of Chl fluorescence classically used to track changes in photosynthetic performance (Bresson et al., 2015). If Chl fluorescence parameters can provide useful tests of photosynthetic performance in vivo and indicate the extent to which performance is limited by photochemical and non-photochemical processes (Baker, 2008), H241, H244 and H226 plants at an IL of 40% can be considered to show excellent photosynthetic performance, avoiding damage by photo inhibition.
Conclusion
The IL of 20% decreased the soil water potential (Ψs) sufficiently to cause water stress in plants and H241 and Jaguar plants showed good performance on growth parameters at an IL of 40%. However, inflorescence time was earlier in H241, H244 and H226 plants. In addition, Chl fluorescence measurements confirmed that genotypes H241, H244 and H226 had higher physiological performance than Jaguar; i.e. they are less probably due to photo inhibition damage. Meanwhile, the IL of 60% caused flooding and plant death without drainage. These results suggest the enforcement of a suitable irrigation level based in available water capacity of substrate (40% in this research) coupled with a vigorous genotype can encourage healthy plants and high water use efficiency.