Introduction
Rhizobacteria can be widely found in agriculture as they are symbiotically associated with legumes. Thus, they receive protection and nutrition from symbiont plant and, in turn, provide nitrogen (N), allowing in many cases the absence of mineral fertilization for this substantial nutrient.
In the 1990s and 2000s, a series of cutting-edge experiments outlined a new paradigm for the interaction between rhizobia and plants. Yanni, Rizk, Abd, El-Fattah, Squartini, Corich, Giacomini, ... Dazzo, F. B. (2001), are one of the first researchers to study rhizobia inoculation on cultivated non-legume plants.
Rhizobia not only act as nitrogen fixers when combined with legumes, but they also stimulate plant growth in non-legume plants. As well, they are capable of synthesizing phyto-stimulating substances, such as auxins (Osório, Gano, Binz, Lima, Martinez, Ramirez, Caballero, de Sá & Giongo, 2014; Ghosh, Kumar & Kanti, 2015), cytokinins (Senthilkumara, Madhaiyan, Sundaram & Kannaiyan, 2009), gibberellin (Miransari & Smith, 2009), ACC deaminase (Nascimento, Brígido, Alho, Glick & Oliveira, 2012), among others. The production of all these phyto-stimulating substances is intrinsically related to an increasing of non-legume crop yield and should be then explored.
As in the acceptance of the atmospheric nitrogen fixation by scientific community, evolving from the 20s to 60s and 70s, to subsequently benefit thousands of farmers throughout the world, it is a consensus that rhizobia acting as growth promoters of non-legume crops through production of phyto-stimulating substances, which are still under evaluation. We strongly believe that only through successive corroborative studies we can reach a common understanding that secondary mechanisms for plant growth promotion can also be introduced in crop succession systems, with considerable benefits.
Therefore, two valuable principles are required: an isolation of plant-bacteria and compatibility studies. In early stages of research, isolation is obtained under axenic conditions (in vitro or under greenhouse conditions) to ensure that the effect was mainly caused by plant-related bacteria. Compatibility studies are needed because rhizobia isolates are beneficial to some species or specific cultivars, but inert to others. Thus, before field studies, an extensive prospecting research both in laboratory and greenhouse are required.
One of the major phyto-stimulating substances produced by rhizobia is auxins, among which Indole Acetic Acid (IAA) is the most studied and the most produced by bacteria. IAA primarily focuses on the formation of side roots and root hairs which increases the uptake of nutrients by plant and is related to shoot dry formation (Lwin, Myint, Tar & Moe, 2012). In addition, IAA is related to colonization strategies of plants by bacteria (Spaepen, Vanderleyden & Remans, 2007), however, the effects of IAA on plant root tissue are concentration dependent and can be species specific (Gutierrez, Matsui, Lincoln & Lovell, 2009).
Different pathways for IAA biosynthesis have been identified in bacteria, being indole-3-acetamide (IAM) and indole-3-pyruvate (IpyA) tryptophan-dependent (Lambrecht, Okon, Broek & Vanderleyden, 2000). The pathway of indole-3-pyruvate (IPyA) was identified in rhizobia (Giraud, Moulin, Vallenet... Sadowsky, 2007) as well as in intermediate components of tryptamide (TRP) (Kaneko et al., 2000) and indole-3-acetonitrile (IAN) (Kaneko et al., 2002).
Millet (P. glaucum), is an exotic plant of African origin that produces high quality forage in a short time, being excellent for feeding cattle. It is widely recommended in Rio Grande do Sul and Santa Catarina states of Brazil for pastures composition and silages, according to their suitability, being highly resistant to drought (Fontanelli, 2012). Sudan grass (S. sudanense) is a forage species originating in Sudan and southern Egypt, adapted to dry and hot climates.
According to Machado, de Sá, Hahn, Oldra, Mangrich, João, Osório, Dias, Dobler & da Silva (2016), Sudan grass and millet are responsive to inoculation with rhizobia. In a study of inoculation with rhizobia in Sudan grass and millet, authors verified an increasing in total dry mass production with rhizobia inoculation.
Given these concerns, this study aimed to evaluate the effect of inoculation with plant growth promoting rhizobia in the yield of millet (P. glaucum) and Sudan grass (S. sudanense) and verify if the enrichment of culture medium with tryptophan interferes with the rhizobium-plant interaction.
Material and methods
Two rhizobia isolates largely studied for the promotion of grass growth, VP16 and UFRGS Lc348, were used. They were obtained from root nodules of white clover (Trifolium repens L.) and birds foot trefoil (Lotus corniculatus L.) plants, respectively. These organisms were obtained from the UFRGS Collection of Rhizobia at the Soil Microbiology Laboratory, Department of Soils, Federal University of Rio Grande do Sul, Brazil.
Rhizobia were assessed for their effect on the initial plant growth and development of adult inoculation of plant growth promoting rhizobia in Sudan grass (Sorghum * sudanense (Piper) Stapf cv. Sudanense) and millet (Pennisetum glaucum (L.) R.Br. cv. BRS1501) plants of Sudan grass and millet. Experiments were carried out in the Soil Microbiology Laboratory and in the greenhouse facilities of the Department of Soils of Agronomy School of the Federal University of Rio Grande do Sul-UFRGS, Brazil.
Quantification of IAA production
Rhizobia UFRGS Lc348 and VP16 were characterized based on IAA production in yeast mannitol (YM) liquid medium enriched with tryptophan and in medium without tryptophan using the method described by Asghar, Zahir, Arshad & Khaliq (2002). Cultures were centrifuged at 10000 rpm for 5 min at 4°C. Subsequently, 1.5 ml of supernatant was collected, and 1 mL of Salkowski solution was added (2mL of FeCl3 + 98mL of HClO4 35%). Samples were stored for 30 min in a dark container for oxidation reaction and color formation (as HClO4 is an oxidizing agent for IAA, and with FeCl3, it produces a pink color whose intensity is proportional to the amount of IAA in the sample).
Production of IAA by rhizobia was quantified in triplicate samples using a spectrophotometer at wavelength of 530 nm. IAA concentration in the samples was calculated by comparison of readings with a standard zero curve as follows: 0.2, 1, 2, 3, 6, 11, 20, 45, 100, 200 and 300 Mg of synthetic IAA mL-1.
Germination and plant growth
Two experiments were conducted in laboratory by inoculating Sudan grass (S. sudanense L. cv. Sudanense) and millet (P. glaucum cv. BRS1501) seeds. Each experiment consisted of six treatments with four replicates in a completely randomized design. Each replicate presented 40 seeds on a petri dish, totaling 48 dishes and 1920 seeds. Treatments were as follows: a) treatments inoculated with VP16 and UFRGS Lc348 rhizobia in YM culture; b) treatments inoculated with the same isolates in YM culture enriched with 50 mg of tryptophan L-1 (VP16 + Trp and UFRGS Lc348 + Trp); c) two control treatments cultivated in YM medium with and without tryptophan (YM Control + Trp and YM Control).
Seeds were previously sterilized by successive immersions in ethyl alcohol (70%) for 30s, followed by sodium hypochlorite (2.5%) for 30s, and five successive washes with sterile distilled water by autoclaving at 120°C for 15 min.
In order to produce the inoculum, bacteria were multiplied in tubes with YM (pH 6.8) and incubated at 28°C for 15 days. Subsequently, each rhizobia culture was inoculated in 80 mL of YM liquid medium, with and without tryptophan, and incubated at 28°C and 120 rpm for 7 days. At the end of this period, the inoculum presented 108 colony-forming units (cfu). mL-1. Each petri dish was sterilized by autoclaving at 120°C for 15 min.
Subsequently, with the aid of a previously flamed tweezer, seeds were sterilized one by one, and carefully laid out on paper towel sheets within dishes. In each dish, 2 mL of sterile distilled water and 0.5 mL of the inoculum for each treatment were added. For two control treatments, 2 mL of water and 0.5 mL of sterile medium (YM Control + Trp and YM Control) or sterile distilled water were added. On the second day, all dishes were moistened again with 2 mL of sterile distilled water. The same procedure was carried out after a period of 5 days.
One day after the experiment started, a first assessment for quantifying and measuring germinated seedlings (in mm) was made. This procedure was carried out every day for seven days. In the course of experiments, dishes with seeds were kept in a dark room at 28°C. At the end of the period, germination rate and germination speed index (GSI) were calculated, which represents the sum of the number of germinated seeds each day divided by the number of cultivation days. Seedlings were dried at 80°C to constant weight in order to determine their dry mass. Data were subjected to analysis of variance and mean test (Scott Knott, 5%), using the statistic software SISVAR 5.6.
Tests in adult plants
Experiments were conducted in a greenhouse with both evaluated plants. Each experiment consisted of eight treatments with four replicates using a completely randomized design. Two of these treatments were inoculated with rhizobia after YM culture (VP16 and UFRGS Lc348), both inoculated with the same YM isolates enriched with 50 mg of tryptophan L-1(VP16 + Trp. and UFRGS Lc348 + Trp.). As well, two treatments of combined inoculations (UFRGS Lc348 + VP16 and UFRGS Lc348 + VP16 + Trp) and two control treatments without inoculation were conducted.
Plastic pots of 2 L previously flamed with ethyl alcohol (99%) filled with an inert mixture of vermiculite and sand (2:1) previously sterilized by autoclaving for 90 minutes at 120°C and 1 atm were used in order to conduct the experiments. Seeds were previously sterilized and pre-germinated in a greenhouse at 28°C for 48 hours, with 8 germinated seeds in each pot. Therefore, thinning was performed 15 days after plant emergence, leaving two plants for each pot.
The inoculum was produced following the same methodology used in the experiments with inoculation in laboratory. After seeding, 3 mL of rhizobia broth containing 108 colony forming units CFU.mL-1 was inoculated in each pot. In treatments with combined inoculation, 1.5 mL of the broth from each isolates was used; and in control treatments, 3 mL of YM medium without inoculation was added.
Nitrogen was weekly added to inoculated treatments and control treatments without inoculation. Control / N treatment and inoculated treatments received weekly doses of NH4NO3 solution (7.14 g.L-1), corresponding to nitrogen at a dose of 75 kg.ha-1 in the end of experiment, yet control N treatments received the equivalent to 150 kg.ha-1.
In the first two weeks of the experiments, 250 mL.pot-1 of N-free nutrient solution was weekly used. After this initial stage, the same quantity of nutrient solution was applied in each pot within an interval ranged from 3 to 5 days.
Seeding was cultivated for 70 days. Plant height was measured, and number of leaves, tillers and panicles determined. Roots and the shoots of plants were separated and then stored in paper bags and placed to dry at 65°C to reach constant weight and later determine the plant dry mass. Data were subjected to analysis of variance and mean test (Scott Knott, 5%), using the software SISVAR 5.6.®
Results
Isolate VP16 produced 4.0 Mg of IAA.mL-1 in YM liquid medium and 10.9 Mg of IAA mL-1 in YM liquid medium enriched with tryptophan. Compared to control treatment, both VP16 and VP16 + Trp, when inoculated on millet seeds, induced epicotyl and hypocotyl higher elongation (Table 1).
Table 1 Plant growth and development parameters of millet seedlings inoculated with rhizobia {in vitro conditions)
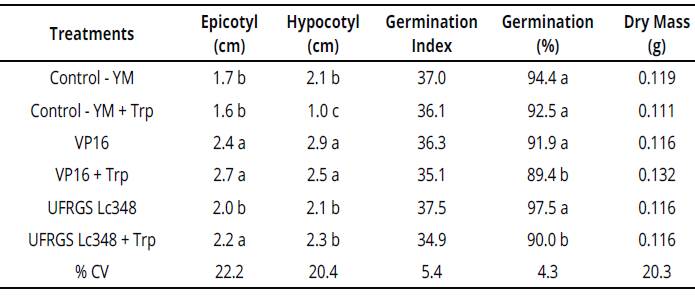
Averages of four replicates, each replicate consisted of 40 seeds. Averages followed by same letter in the column do not differ by the Scott Knott test at 5% error probability.
Averages without letters in the column do not differ. YM: yeast manitol, Trp.: tryptophan, % CV= coefficient of variation percentage.
These in vitro stimuli render an adaptive advantage of cultivar BRS1501 and may induce a more effective search for water and nutrients.
Isolate UFRGS Lc348 produced 1.1 Mg of IAA. mL-1 in YM liquid medium and 60.7 Mg of IAA.mL-1 in YM liquid medium enriched with tryptophan. Compared to both control and UFRGS Lc348 treatments, UFRGS Lc348 + Trp presented a greater epicotyl length in millet seedlings (Table 1), possibly due to high production of IAA by isolate UFRGS Lc348 in a tryptophan-enriched medium.
In vitro experiment, inoculations of the VP16 isolate in millet induced a higher epicotyl and hypocotyl length, but no direct gains were observed in plant dry mass or anticipation of germination. Thus, although they do not have allowed direct gains in dry mass, these stimuli induced by VP16 in millet can mean advantages in a dispute for nutrients in the rhizosphere, or through photosynthetic radiation, which indirectly favors the plant growth and initial development of millet.
Despite inducing greater in vitro millet seedlings length, largest production of indole substances by rhizobia in enriched substrate (VP16 + Trp and UFRGS Lc348 + Trp treatments), had achieved a decreasing in the number of germinated seeds at the end of the process (Table 1). On the other hand, the effects observed in in vitro millet seedlings were not observed in experiment with adult plants under greenhouse conditions (Table 2).
Table 2 Plant growth and development parameters of millet at 60 days after inoculation (greenhouse conditions)
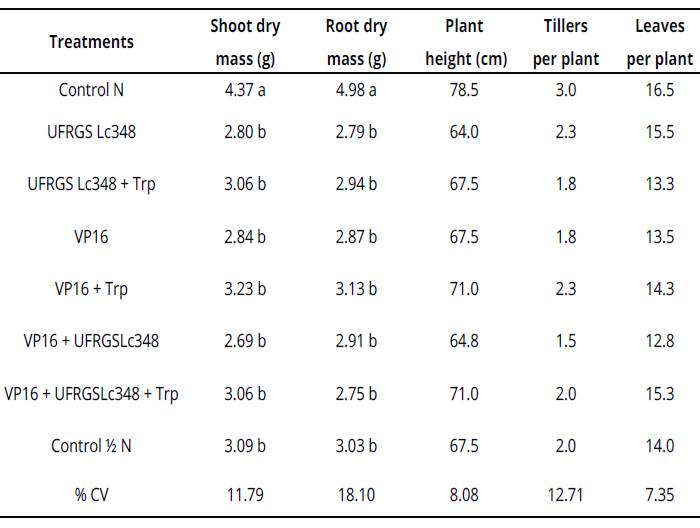
Averages of four replicates, with two plants per replicate. Averages followed by same letter in the column do not differ by the Scott Knott test at 5% error probability. Averages without letters in the column do not differ. For statistical analysis, variables number of tillers per plant and number of leaves per plant were transformed according to the formula: ((X + 1)0,5). %CV= coefficient of variation percentage, Control N: treatment not inoculated with nitrogen dose equivalent to 150 kg.ha-1, Control Κ N: treatment not inoculated with nitrogen dose equivalent to 75 kg.ha-1. Values without letters in the column did not differ.
Indole substances from the culture medium enriched with tryptophan were diluted in pots of 2 L, which is possibly associated to the inefficiency of millet inoculations under greenhouse conditions.
In experiments with in vitro Sudan grass inoculation, UFRGS Lc348 + Trp stimulated the seedling, inducing a greater length of the hypocotyl. The length of inoculated Sudan grass hypocotyl with UFRGS Lc348 was 60% higher than YM control treatment, indicating a beneficial effect to seedlings (Table 3).
Table 3 Plant growth and development parameters of Sudan grass seedlings inoculated with rhizobia (in vitro conditions).
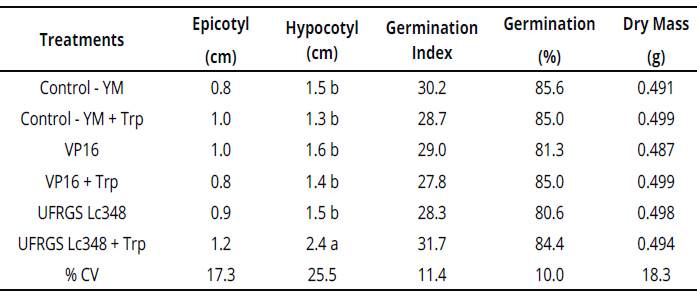
Averages of four replicates, each replicate consisted of 40 seeds.
Averages followed by the same letter η the column do not differ by the Scott Knott test at 5% error probability.
Averages without letters in the column do not differ. YM: yeast manitol.Trp.: tryptophan, %CV= coefficient ofvariation percentage.
However, treatments inoculated with isolate UFRGS Lc348 in a greenhouse were the worst, including control treatment / N. Indole substances produced by VP16, much lower than the one with the isolated UFRGS Lc348 enriched with tryptophan, did not affect the in vitro growth of Sudan grass. However, VP16 + Trp increased the growth of the shoot dry mass of adult plants in 13.3 %, compared to control treatment with the same dose of nitrogen (Table 4).
Table 4 Plant growth and development parameters of Sudan grass at 60 days after inoculation (greenhouse conditions).
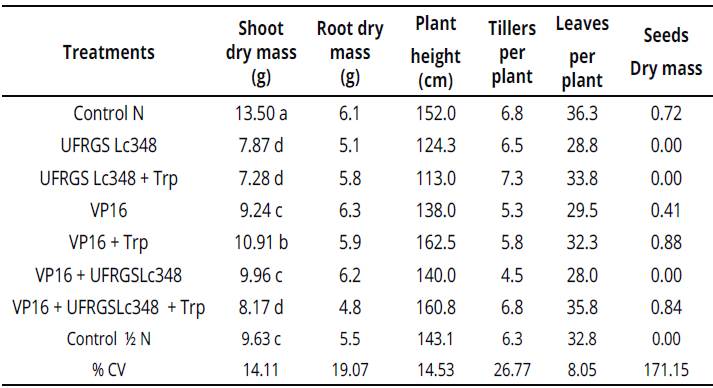
Averages of four repetitions, with two plants per repetition. Averages followed by the same letter in the column do not differ by the Scott Knottiest at 5% error probability.
Averages without letters in the column do not differ. For statistical analysis, the variables number of tillers per plant and number of leaves per plant were transformed according to the formula: ((X + 1)0,5). %CV: percent coeficiente ofvariation, Control N:treatment not inoculated with nitrogen dose equivalent to 150 kg.ha-1, Control Κ N: treatment not inoculated with nitrogen dose equivalent to 75 kg.ha-1. Values without letters in the column did not differ.
Enriching the medium with tryptophan was essential to the success of interacting VP16 with Sudan grass, as a simple inoculation with VP16 did not surpass the control treatment 1 N. The interaction between Sudan grass and rhizobia VP16 should be tested in field experiments, and, if the effects of inoculation still persists, will be possible to reduce nitrogen fertilizers without damaging Sudan grass yields.
Another important finding was the production of Sudan grass seeds 70 days after seeding. When inoculating isolate VP16 (0.41g of seeds.plant-1), VP16 + Trp (0.88g of seeds.plant-1) and VP16 + UFRGS Lc348 + Trp (0.84g of seeds.plant-1). In Control treatment / N, no seeds production during the experiment could be observed.
Discussion
A deleterious effect of inoculation may be associated with a large production of the hormone, which has herbicidal action when in high concentrations (Malik & Sindhu, 2011; Senthilkumara, Madhaiyan, Sundaram & Kannaiyan, 2009). On the other hand, Schlindwein, Vargas, Lisboa, Cruz, Eichelberger, Gabiatti, Prates & Stumpf (2008), observed similar results in lettuce seedlings, in which the rhizobacteria inoculation able to synthesize a great amount of indole substances damaged the crop yield.
These results open a range of new hypotheses to further studies, for example, the exudation of tryptophan by Sudan grass roots. There are some plants able to produce tryptophan, which under field conditions, could be used by rhizobia in IAA synthesis. Kravchenko, Azarova, MakarovaI & Tikhonovich (2004), reached a similar result, when they quantified tryptophan exudation of tomato and radish aseptic roots. A tomato plant had released up to 5.3 ng of tryptophan daily, while radish released up to 390 ng of tryptophan daily. In the same study, authors observed that radish root mass increased around 36% to inoculate Pseudomonas growth promotion rhizobia, while inoculating the same bacteria in tomato, which was inefficient.
Yanni et al. (2001), and Osorio et al. (2014), reported grass growth promotion by using phyto-stimulating substances for rhizobia inoculation. If field conditions present the same results, effects of these inoculations may take into account stop using nitrogenous fertilizers without damaging crop yields. These mechanisms are additional tools to biological nitrogen fixation in legumes and should be considered for better rhizobia integration in intercropping or crop rotation systems.
Despite having a high variance associated to seed production parameter, rhizobium VP16 induced certain precocity of Sudan grass, which can be interesting in seed or Sudan grass silage production systems.
The next step in compatibility tests between pastures and plant growth promotion rhizobia should be pasture stimulus field of Sudan grass through inoculating VP16. Field conditions possibly provide tryptophan in an amount sufficient to synthetize indole substances due a complex system enriched with root exudates and decomposing tissues. Thus, there can be an increasing in Sudan grass plantation after the inoculation with VP16. Without mineral fertilization, is certainly interesting for the sustainability of pasture management systems. As it increases green mass production without increasing the costs with mineral fertilization, which reduces the risk of loss and environmental pollution by using nitrogen fertilizers.
Conclusion
Inoculation of millet BRS1501 with isolate VP16 cultivated in medium with and without tryptophan induces higher seedling growth, while isolate UFRGS Lc348 induces greater length for the hypocotyl of millet seedlings cv. BRS1501. Despite these stimuli in the initial phase, no effect of inoculation on millet adult plants could be observed.
In Sudan grass, the inoculation of tryptophan-enriched VP16 increases the shoot dry mass of adult plants.