Introduction
The excessive use of phytosanitary products (PPs) in agriculture has led to the development of insecticide resistance, the generation of chemical residues, and the elimination of beneficial insects, as well as to environmental pollution and human toxicity. However, the demand for healthy and contamination- free food has promoted the development of alternative control measures for pest and disease control (Lacey et al., 2015; Nguyen et al., 2015; Potrich et al., 2018).
Among these alternative control measures, entomopathogenic fungi (EF) are considered important to control and reduce pest populations in the post-harvest of cereal Fcrops. However, they cannot totally replace synthetic chemical insecticides. Thus, several studies have evaluated the combination of EF and chemical insecticides to be used in integrated pest management (IPM) programs (Lacey et al., 2015; Dal Bello et al., 2018; Fregonesi et al., 2016). This alternative would allow the reduction of insecticide application due to the presence of substances contained in PPs, which act as insect stressors that stimulate fungal infection and thus increase the efficiency of pest control. It may also minimize the dangers for human and animal health and environmental contamination because it would imply applying low doses or subdoses of insecticides ( Moino and Alves, 1998).
However, PPs can also act deleteriously on microorganisms. In particular, the toxicity of these products on EF may vary with the fungal species and strains, the chemical nature of the active ingredient, the action mode, and the product formulation and doses, as well as with the environmental conditions (Pessoa et al., 2020). These factors can inhibit fungal vegetative growth and conidial survival, prevent the occurrence of genetic mutations, and alter the virulence of the EF (Alves et al., 2008; Oliveira et al., 2018). Thus, before recommending a specific PP to be used in combination with an EF, the action of these products on the microorganisms requires the knowledge and evaluation of the compatibility between them (Lacey et al., 2015; Fregonesi et al., 2016).
Based on this, the aim of the present work was to study the effects of two PPs commonly used in Argentina and Brazil combined with three EF (Beauveria bassiana, Metarhizium anisopliae and Cordyceps (Isaria) fumosorosea), for the control of insect pests in the post-harvest of wheat, under laboratory and semi-field conditions.
Materials and methods
The study was carried out at the Reference Laboratory Unit (RLU) for Biological Control of the Advanced Center for Research in Plant Protection and Animal Health of the Instituto Biológico, located in Campinas, Sao Paulo, Brazil.
Entomopathogenic fungi (EF)
EF used in this study were obtained from the collection of entomopathogenic fungi “Oldemar Cardim de Abreu” from the RLU for Biological Control: Beauveria bassiana isolate IBCB 66, Metarhizium anisopliae isolate IBCB 425, and Cordyceps (Isaria) fumosorosea isolate IBCB 130 (Table 1). The isolates were cultured on potato dextrose agar (PDA) and incubated at 25 ± 2 °C and a photoperiod of 12:12 h (light: darkness) for 14 days until obtaining a matrix plate.
Phytosanitary products (PPs)
The PPs used in this study are commonly recommended for the control of insect pests in post-harvest in both Argentina and Brazil (Table 2). The following five PP treatments were evaluated: Pirimiphos methyl 6 mL L-1 (PM6), Pirimiphos methyl 10 mL L-1 (PM10), Pirimiphos methyl 16 mL L-1 (PM16), Pirimiphos methyl 8 mL L-1 + Lambdacialotrine 2 mL L-1 (PM8 + L2), and Lambdacialotrine 7 mL L-1 (L7).
Bioassay 1: Assessment under in vitro laboratory conditions
Broth test: Germination bioassay (conidia + PPs)
In the first in vitro experiment, each PP was dissolved in 0.01 % (v/v) Tween 80 (sodium polysorbate) in sterile distilled water and then combined with conidia of B. bassiana, M. anisopliae or C. fumosorosea, mixed separately. The control had conidia without PPs. Then, this mixture (conidia + PPs) was vortexed for 2 min to homogenize the suspension. One hour later, in absolute rest at room temperature, a 0.1 mL aliquot from each mixture was spread with a Drigalsky loop onto the PDA contained in five sterile Petri dishes (90 mm in diameter). The experiment was repeated twice (n = 10 replicates). The dishes were incubated at 25 ± 2 °C and a photoperiod of 12:12 h (light: dark). After 18 h of incubation, the germination percentage was calculated (adapted from Rossi-Zalaf et al., 2008).
Compatibility calculation of the biological index (BI): Vegetative growth (colony diameter), sporulation and germination percentage bioassays
The compatibility between the different PP treatments and the EF chosen was determined in vitro. To this end, PPs were incorporated into the autoclaved PDA before it solidified, at approximately 40 - 45 °C. The mixture was poured into five disposable sterilized Petri dishes. After solidification, the B. bassiana, M. anisopliae and C. fumosorosea isolates were inoculated with a platinum loop at three equidistant points per dish on the surface of the medium. The control had PDA without PPs. The experiment was repeated twice. The dishes were incubated at 25 ± 2 °C and a photoperiod of 12: h (light:dark). After 14 days of incubation, vegetative growth was evaluated by measuring the diameter in two perpendicular senses in each colony to obtain the mean diameter.
The fungal colonies described in the previous bioassay were cut with a scalpel and transferred individually to a sterile glass tube containing 10 mL of 0.01 % (v/v) Tween 80 (sodium polysorbate) in sterile distilled water. The conidia of each colony were dislodged from the PDA by vortexing for 2 min. Successive dilutions were performed until the desirable suspension was obtained to quantify sporulation using a hemocytometer (Neubauer chamber), and germination percentages were calculated using the same methodology described above (Adapted from Rossi-Zalaf et al., 2008).
The compatibility between the evaluated PPs and EF was calculated based on the BI, according to Rossi-Zalaf et al. (2008), as follows: BI = [47(VG) + 43(ESP) + 10(GER)]/100, where BI = biological index, based on the percentage of vegetative growth (colony diameter) (VG), the percentage of sporulation (ESP), and the germination percentage (GER) of fungal colonies in relation to the respective control. The limits established were: Toxic: 0 - 41; moderately toxic: 42 - 66; and compatible: > 66.
Colony forming units (CFUs)
Conidia were inoculated on the surface of 15 g of wheat grains onto Petri dishes by spraying them with 1 mL of B. bassiana, M. anisopliae or C. fumosorosea at a concentration of 2 x 108 conidia mL-1 using a Potter tower (Burkard Manufacturing Ltd., Mod. 1, England). After 1 h, all the PP treatments were sprayed in the same way. The control was sprayed with each fungal suspension and 0.01% (v/v) Tween 80 (sodium polysorbate) in sterile distilled water. After application, Petri dishes were incubated at 25 ± 2 °C and a photoperiod of 12:12 h (light: dark) (the same conditions as described above), and then evaluated for 24, 48 and 72 h (times of persistence of EF on grains). Then, 1 g of wheat grains was collected at random from each Petri dish, diluted with 10 mL 0.01 % (v/v) Tween 80 (sodium polysorbate) in sterile distilled water and vortexed for 2 min for homogenization. Then, 0.1 mL of each dilution was inoculated with a Drigalsky loop on the surface of Petri dishes containing PDA supplemented with 0.5 mg L-1 of pentabiotic. Five replicates were used, and the experiment was repeated twice. The plates were incubated for 4 days at the same conditions described in the previous assay. After this period, colonies were counted and the number of CFUs was quantified.
Statistical analysis
For each isolate, germination percentage, colony diameter, sporulation, and number of CFUs were analyzed. Differences between PP treatments were verified assessing whether the data met the assumptions of normality and homogeneity of variance with the Shapiro-Wilk and Levene’s tests, respectively. In the case of reaching the assumptions, an ANOVA F-test was used, whereas in the case of not reaching the assumptions, the Welch’s t-test (with normality and heterogeneity of variance), the Kruskal- Wallis test (with non-normality and homogeneity of variance), or the Fisher-Pitman permutation test (with non-normality and heterogeneity of variance) were used. The post-hoc analyses used for each situation were the Tukey’s HSD test, the t-test for difference of pairs, the Wilcoxon test and the test of difference of pairs with permutations. The global tests were considered significant when the p value < 0.05. In the case of multiple comparisons, a significance of 5 % was considered for the Tukey’s HSD test, while for the remaining tests to achieve a false discovery rate of 5 %, the p values were adjusted using the Benjamini- Hochberg method. All analyses were carried out with the R software version 4.0.3.
Results
Bioassay 1: Assessment under in vitro laboratory conditions
Broth test: Germination bioassay (conidia + PPs)
In each of the isolates studied, the mean germination percentages were reduced by the effect of the PPs, with differences between the treatments and the control (Table 3). Particularly, the PPs with the active ingredient pirimiphos methyl led to a gradual reduction in the germination percentage as the dose increased. PM16 caused the greatest reductions in the germination percentage of B. bassiana and C. fumosorosea, followed by PM10. Differently, in M. anisopliae, PM10 showed no differences in relation to PM16. PM8+L2 caused the lowest reductions in the three isolates (Table 3).
Table 3 Germination percentage (mean ± SE) of B. bassiana (IBCB 66), M. anisopliae (IBCB 425), and C. fumosorosea (IBCB 130) with different PP treatments
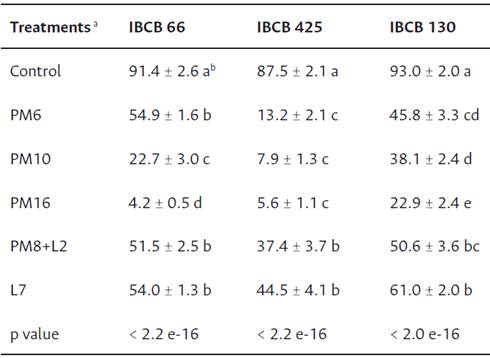
Note. a Control = control treatment; PM6 = Pirimiphos methyl 6 mL L-1; PM10 = Pirimiphos methyl 10 mL L-1; PM16 = Pirimiphos methyl 16 mL L-1; PM8+L2 = Pirimiphos methyl 8 mL L-1 + Lambdacialotrine 2 mL L-1; L7 = Lambdacialotrine 7 mL L-1. b Means followed by different letters in the column for each isolate tested are significantly different (p value < 0.05).
Compatibility
All isolates showed differences in their colony diameter, sporulation and germination percentage between the treatments and the control (Table 4). In all the fungal isolates, PPs caused a decrease in colony diameter (Figure 1). The greatest decrease was observed with the application of PM16 and PM8+L2, followed by L7 with great variability, and then by PM10 and PM6, both of which led to the lowest decrease. In B. bassiana and M. anisopliae, no differences between PM10 and PM6 were found.
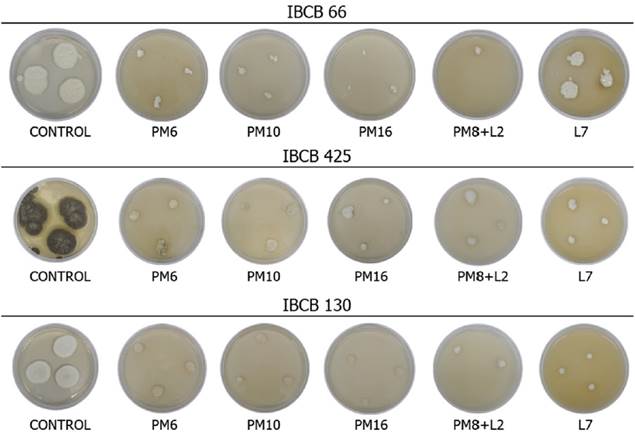
Figure 1 Vegetative growth (colony diameter) of B. bassiana (IBCB 66), M. anisopiiae (IBCB 425), and C. fumosorosea (IBCB 130) with different PP treatments. Note. Control = control treatment; PM6 = Pirimiphos methyl 6 mL L-1; PM10 = Pirimiphos methyl 10 mL L-1; PM16 = Pirimiphos methyl 16 mL L-1; PM8+L2 = Pirimiphos methyl 8 mL L-1 + Lambdacialotrine 2 mL L-1; L7 = Lambdacialotrine 7 mL L-1.
Table 4 Biological Index (BI): Colony diameter (mean ± SE), sporulation (mean ± SE) and germination percentage (mean ± SE) of B. bassiana (IBCB 66), M. anisopiiae (IBCB 425), and C. fumosorosea (IBCB 130) with different PP treatments
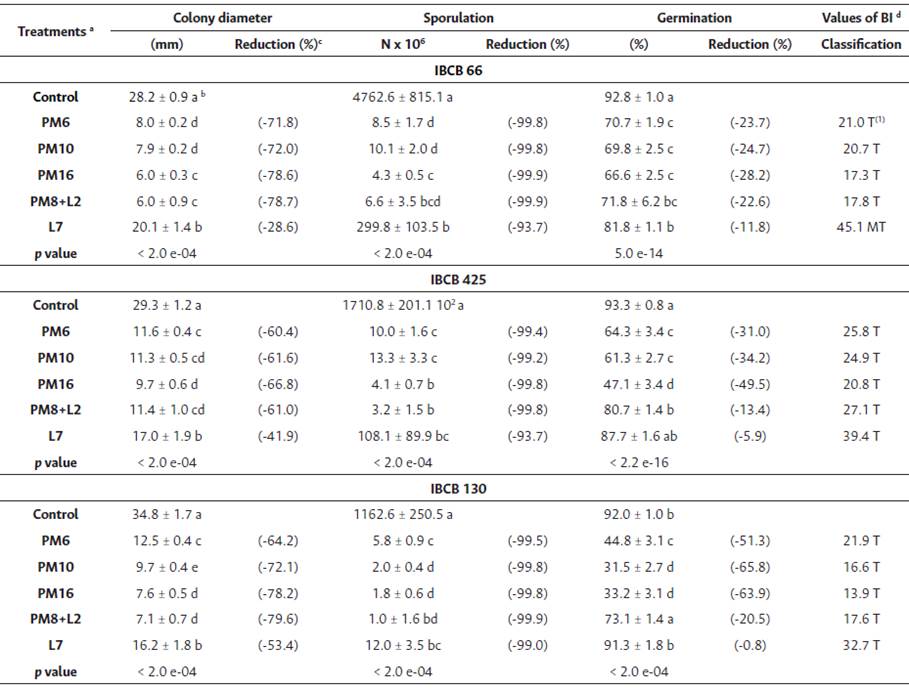
Note. a See Table 3. b See Table 3. c Equation = Reduction (%): [Treatments mean/control mean) x 100]-100. d Values of BI (Rossi- Zalaf et ai., 2008). Toxic = 0 - 41 (T); moderately toxic = 42 -66 (MT); and compatible = > 66 (C).
PPs also caused a decrease in sporulation of all the isolates. In B. bassiana, the lowest mean sporulation was observed with the application of PM8+L2, followed by PM16. PM6 and PM10 caused lower mean decreases than the previous treatments, but greater than L7. In contrast, in M. anisopliae, the lowest mean sporulation was observed with the application of L7. This treatment had great variability, so that it was like PM8+L2 and PM16, as well as with PM6 and PM10. Finally, in C. fumosorosea, the lowest mean sporulation was observed with the application of PM16, PM10 and PM8+L2 (without significant differences between them), followed by PM6 and L7.
PP also caused a decrease in the germination percentage compared to the control in all the isolates, except for L7 in M. anisopliae and C. fumosorosea. In B. bassiana, the lowest mean germination was observed with the application of PM16, PM10 (without significant differences between them) and PM6. L7 caused lower mean decreases than the previous treatments and did not show differences with PM8+L2. The latter treatment had great variability, so that it was similar to PM16, PM10 and PM. In contrast, in M. anisopliae, the lowest mean germination was observed with the application of PM16, followed by PM10 and PM6. PM8+L2 caused lower mean decreases than the previous treatments. Finally, in C. fumosorosea, the lowest mean germination was observed with the application of PM16 and PM10 (without significant differences between them), followed by PM6. PM8+L2 caused lower mean decreases than the previous treatments.
The BI showed that the PP treatments were not compatible with any of the three isolates, ranging from 13.91 to 39.42 (i.e. toxic), except for the L7 treatment in B. bassiana, which was moderately toxic (45.08) (Table 4).
Bioassay 2: Assessment under semi- field conditions
Colony-forming units (CFUs)
The PPs evaluated did not affect the number of CFUs of B. bassiana or C. fumosorosea with respect to the control, independently of the persistence time of EF on the grains. In B. bassiana, the variation percentage ranged from 2 to 50 % between the different times evaluated, whereas in C. fumosorosea, it ranged from 0 to 25 %. In M. anisopliae, the number of CFUs varied, independently of the persistence time of EF on the grains. The greatest decrease in the number of CFUs was observed with the application of L7, followed by PM16, PM10, PM6 and PM8+L2. The control showed a mean compatible with all PPs (Table 5).
Table 5 N umber of colony forming units (mean ± SE) of B. bassiana (IBCB 66), M. anisopiiae (IBCB 425), and C. fumosorosea (IBCB 130) with different PP treatments evaluated for 24, 48 and 72 h (times of persistence on grains)
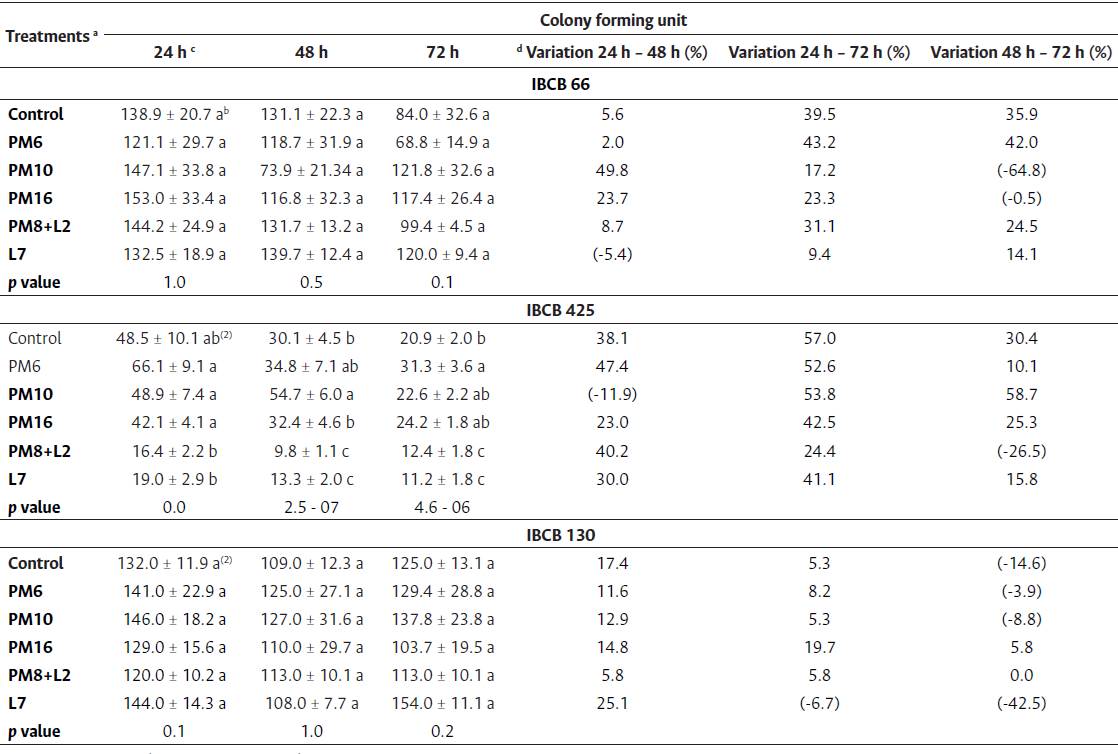
Note. a See Table 3. b See Table 3. c x103. d Equations: Variation 24 h - 48 h % = [48 h mean/24 h mean) x 100]-100; Variation 24 h - 72 h % = [72 h mean/24 h mean) x 100]-100; Variation 48 h - 72 h % = [72 h mean/48 h mean) x 100]-100.
Discussion
The combination of PPs and EF could be a new alternative in IPM programs for improved control and regulation of insect pest populations in the post-harvest of cereal crops (Moino and Alves, 1998; Lacey et al., 2015). In this study, both positive and negative interactions were observed depending on the different active ingredients of the PPs, the entomopathogenic fungal species and the application conditions.
The tests performed under laboratory conditions and in the direct culture medium showed that the PPs evaluated had a toxic effect on EF and affected negatively their vegetative growth, germination percentage and sporulation. Oliveira et al. (2018) and Pessoa et al. (2020) showed that in vitro studies have the advantage of exposing the microorganism as much as possible to the action of PPs, which could explain the results obtained in this study.
When evaluating the combination between PPs and EF, the germination percentage is considered as the most important factor because pathogens infect insects through conidia germination by ingestion or contact. In this study, the PP treatments evaluated caused a reduction in the fungal germination percentage with respect to the control. Thus, the use of the mixtures with EF is not recommended. This agrees with the results observed by Mamprim et al. (2014), who found inhibition of B. bassiana isolates when combined with several insecticide formulations, and thus proposed separate applications of B. bassiana and insecticide to prevent severe interaction.
In the compatibility calculation, the vegetative growth (colony diameter) represents the growth potential of the fungus (Potrich et al., 2018). In the present study, the PPs used reduced this parameter in the three fungi evaluated. In contrast, Pessoa et al. (2020) observed that the vegetative growth of B. bassiana increased when it was applied together with PPs. These authors considered that, when microorganisms are in the presence of toxic compounds that alter the culture medium and impair their development, they use all their reproductive effort, thus resulting in greater vegetative growth.
The sporulation of an isolate is important for the maintenance of the pathogen-host cycle, since the increase of this parameter results in greater dispersal of propagules in the environment (Potrich et al., 2018). In the present work, this parameter was reduced by the PPs evaluated as compared to control conditions. In contrast, Alves et al. (2008) and Fregonesi et al. (2016) found that PPs do not inhibit the sporulation of EF. Moino and Alves (1998) explained that vegetative growth and sporulation may be promoted by a mechanism of physiological resistance of microorganisms, which can metabolize the toxic compounds of the active ingredient, or, by using the molecules resulting from this process, release them in the culture medium as secondary nutrients.
In this study, the treatments with organophosphates, particularly PM16, had toxic effects on almost all the isolates tested, with the exception of the B. bassiana isolate tested with the L7 pyrethroid treatment, whose effect was moderately compatible. Fregonesi et al. (2016) observed differences in the response to different PPs in isolates of B. bassiana. Moreover, as pointed out by Oliveira et al. (2018), the commercial formulations prepared with the same active ingredient by different manufacturers can present different biological effects. Also, the addition of other products in the preparation, for example, emulsifiers and additives, can contribute to having varied biological effects on vegetative growth and sporulation.
The results observed here showed that, when PPs were applied under semi-field conditions in which the exposure was conditioned by several factors, the number of CFUs of the fungi was not affected by the PPs. Large productions of conidia on the substrate are fundamental for pathogen control. This coincides with that reported by Dal Bello et al. (2018), who showed the highest survival of EF. This demonstrates that the incompatibility in vitro does not always indicate that the same occurs under field conditions (Alves et al., 1998).
Conclusion
It is important to study the effects of PPs in combination with EF to reduce the doses and number of applications of PPs. The treatment with the active ingredient lambdacialotrine would be the most effective compared to the treatments with pirimiphos methyl, which led to a gradual reduction in the germination percentage as the dose increased. On the other hand, PPs caused a decrease in colony diameter, sporulation and germination percentage in all the isolates. In consequence, the BI showed that the treatments were not compatible with any of the three isolates given their toxicity. Based on the results obtained, it was concluded that the application of PPs and EF is recommended separately and under semi- field conditions. However, further research under field conditions should be conducted to confirm the compatibility of PPs and EF within an IPM strategy.