INTRODUCTION
The common bean (Phaseolus vulgaris L. 1973) is one of the most important leguminous food crops in Mexico, where it is considered the center of origin, and worldwide, due to its significant content of carbohydrates, proteins, lipids, vitamins, and minerals (Chavez-Servia et al., 2016; Mecha et al., 2018). Similarly to other crops, the common bean is affected by the presence of plant pathogens that limit its production, such as the ubiquitous soil-borne fungus Rhizoctonia solani Kühn 1858 (Teleomorph: Thanatephorus cucumeris). R. solani is a plant pathogen with a broad host range (Valentín Torres et al., 2016) that causes the rot of hypocotyl and roots in the common bean, causing a devastating disease known as damping-off. In addition, an anastomosis variant of this pathogen (AG4 HI) can cause the foliage web blight disease in tropical areas (Valentín Torres et al., 2016; Martins et al., 2018). Current practices for pathogen control rely on chemical treatments, although this strategy is not always desirable for their negative effects in the environment and human health (Abdel-Fattah et al., 2011). It is necessary to improve the sustainability of crop production systems, including the common bean. Thus, biological control represents an alternative method to reduce the use of agrochemicals in crop protection (Mayo- Prieto et al., 2019).
Beneficial microorganisms can activate defense responses in plants, through the induction of gene expression and the production of secondary metabolites (Köhl et al., 2019). Phenolic compounds are secondary metabolites induced in plants by different factors, and include molecules with antimicrobial activity, or involved in signaling pathways, which play important roles in plant-microorganism interactions (Mandal et al., 2010). It has been reported that symbiosis can change the content of phenolic compounds in plants, e.g., a formulation of a mixture of Egyptian mycorrhizal fungi increased the total phenol content in the common bean, and significantly reduced both disease severity and disease incidence in R. solani (Abdel-Fattah et al., 2011). Also, it has been reported that total soluble phenol and flavonoid content was boosted in common bean roots after arbuscular mycorrhizal fungi (AMF) colonization, suggesting that the plant immune system was strengthened, and this was manifested as a reduction in the incidence and severity of infection by the pathogen Fusarium solani (Mart.) Sacc., 1881 (Eke et al., 2016). Inoculation of tomato plants with the AMF Rhizophagus irregularis (Blaszk., Wubet, Renker and Buscot) C. Walker and A. Schüßler 2010 (formerly Glomus intraradices) increased total phenol content and reduced the root-knot nematode infection (Sharma and Sharma, 2017). In addition, individual phenolic acid concentrations (caffeic, chlorogenic and ferulic) have been reported in R. irregularis and Funneliformis mosseae (T.H. Nicolson and Gerd.) C. Walker and A. Schüßler 2010 (formerly G. mosseae) interactions in tomato root plants (López-Ráez et al., 2010). On the other hand, genes involved in the phenylpropanoid biosynthesis, such as PAL1 and HQT, are up-regulated by the mycorrhizal colonization of Tomato mosaic virus infected tomato plants (Aseel et al., 2019). However, no reports on the individual phenolic profile in the common bean co-inoculated with AMF and pathogens have been published. The aim of this work was to analyze the effect of the Mexican biofertilizer Rizofermic-UV, which is formulated as a mix of multi-taxa arbuscular mycorrhizal fungi, on the infection of the common bean by the soil-borne pathogen R. solani, as well as the total and individual phenolic (phenolic acids and flavonoids) compound content profi le during the infection.
MATERIALS AND METHODS
Common bean seeds
Common bean seeds (variety Azufrasin) were provided by the CEVAF (Valle del Fuerte Experimental Field Station from Instituto Nacional de Investigaciones Forestales, Agrícolas y Pecuarias) from the harvest of January 2016. This variety was developed from crosses between three lines (Azufrado Pimono-78/Canario-72)//AZPA-5) in CEVAF, Sinaloa, Mexico.
Biofertilizer
Rizofermic-UV is a biofertilizer formulated as a consortium of 12 arbuscular mycorrhizal fungi [Acaulospora morrowiae Spain and N.C. Schenck 1984, Acaulospora scrobiculata Trappe, Acaulospora spinosa Walker and Trappe 1981, Claroideoglomus etunicatum (W.N. Becker and Gerd.) C. Walker and A. Schüßler 2010, Funneliformis geosporus (T.H. Nicolson and Gerd.) C. Walker and A. Schluessler 2010, F. mosseae, Gigaspora decipiens I.R. Hall and L.K. Abbott 1984, Gigaspora rosea T.H. Nicolson and N.C. Schenck 1979, Glomus aggregatum N.C. Schenck and G.S. Sm 1982, Glomus macrocarpum Tul. and C. Tul. 1845, Rizhophagus intraradices (N.C. Schenck and G.S. Sm.) C. Walker and A. Schüßler 2010, and Scutellospora pellucida (Nicol. and N.C. Schenck) C. Walker and F.E. Sanders 1986], produced in Mexico by the Laboratory of beneficial microorganisms of the Universidad Veracruzana.
Pathogenic fungal inoculum
Rhizoctonia solani strain was kindly donated by Quintin Armando Ayala Armenta, Professor at the Universidad Autónoma de Sinaloa-Faculty of Agronomy. The inoculum was prepared by growing the fungus in bottles containing sterilized rice grain medium at 25 ± 2 °C for 15 days.
Planting, AMF inoculation, and pathogen infection
Common bean seeds were surface-sterilized by immersion in ethanol for two minutes followed by five minutes in 0.5 % sodium hypochlorite, rinsed four times with distilled water and sown in a pot (250 mL) containing a mix of sterile vermiculite and fine-sand (3:1). Half of the pots received 1 g of Rizofermic-UV inoculum per pot (according to the manufacturer's recommendation) at the moment of sowing, and as a soil drench two weeks after sowing (Abdel-Fattah et al., 2011). Plants were fertilized twice a week with irrigation based on the modified Hoagland solution (Miller and Kitt, 1992), which contains one tenth (20 µM) of the regular potassium phosphate strength, for mycorrhizal and non-mycorrhizal sets. The experiments were carried out under greenhouse conditions to analyze the effects of Rizofermic-UV inoculation, on an eight h light (25 °C)/16 h dark (19 °C) photoperiod until harvest. Four weeks after planting, 1 g of infected rice seeds with R. solani was incorporated to the plant substrate to half of the mycorrhizal and non-mycorrhizal sets of plants. The inoculum was set surrounding the stem of the plantlets (Abdel-Fattah et al., 2011). The four treatments: 1) No biofertilizer with no R. solani (Ctrl); 2) Biofertilizer with no R. solani (BF); 3) Biofertilizer with R. solani (BF-Rs); 4) No biofertilizer with R. solani (Rs) were arranged in a completely randomized design.
Harvest, growth parameters and diseases assessment
One week after pathogen inoculation and five weeks after planting, sixteen plants from each treatment were carefully unrooted, and their roots washed using tap water. The disease incidence and severity as well as the growth parameters were registered at the harvest time. The disease incidence was determined by counting the number of plants exhibiting R. solani rot symptoms and expressed as a percentage of the total number of the plants assessed. The severity rate on roots and hypocotyls was evaluated based on a 1 to 4 disease scale: 0 = no damage; 1 = minor discoloration of the hypocotyl; 2 = discoloration plus small necrotic lesions (<1 mm in diameter) on the stem, hypocotyl, or roots; 3 = discoloration with large necrotic lesions (≥1 mm in diameter) on the stem, hypocotyl, or roots; and 4 = death of the seedling (Carling et al., 1999). Disease severity was further calculated using the formula DS (%) = Σ(ab)x100/AK, where α = number of diseased plants having the same degree of infection; b = degree of infection; A = total number of examined plants and K = highest degree of infection (Eke et al., 2016).
Root colonization
For the estimation of AMF root colonization, during harvest time, one portion of the root system from each plant was taken and washed with distilled water to remove substrate particles; immersed in 10 % (w/v) KOH; and heated for several 30 s intervals, until clarified. Subsequently, roots were rinsed twice with distilled water, and hydrogen peroxide (H2O2) was added. Then, roots were incubated at room temperature for 3-5 min. The hydrogen peroxide was removed, and the roots were rinsed three times with tap water, then stained with trypan blue (0.05 %) (Phillips and Hayman, 1970), and heated in the microwave for 30 s. The percentage of colonization was determined for each plant by the gridline intersection method, as previously described (Giovannetti and Mosse, 1980). Non-mycorrhizal control plants were also analyzed for colonization. No mycorrhiza-related structures or external hyphae were detected in non-inoculated roots at any point.
Total phenolic content estimation
Phenolic extracts were prepared from 0.20 g of macerated fresh roots from P. vulgaris, from a pool of four plants, four pools for each treatment; sixteen plants in total were considered for each treatment. Samples were macerated in methanol (80 %) and incubated for 3 h at room temperature. The methanol was thoroughly evaporated at 40 °C using a rotary evaporator, and the aqueous phase was adjusted to 1 mL. Total phenolic content of the phenolic extracts was determined by the Folin-Ciocalteu assay, adapted to a 96-well microplate format (Nurmi et al., 1996). Briefly, 140 µL of distilled water, 10 µL of extract (or 80 % ethanol as blanks, and gallic acid or (+)-catechin as standard), 10 µL of freshly prepared Folin-Ciocalteu reagent, and 40 µL of 7.5 % Na2CO3 were mixed in a microplate well. The absorbance was monitored at 760 nm, and the results were expressed in mg of gallic acid equivalents (GAE) and (+)-catechin equivalents (CE) per g of bean root tissue.
Phenolic profile analysis by HPLC
The phenolic profile was determined from the 80 % methanol extracts (filtered with 0.2 µm PTFE) using a HPLC system Dionex UltimMate DAD-3000 (Thermo Fisher Scientific, New York, NY), equipped with a titanium quaternary pump, an autosampler, a photodiode array detector (DAD-3000), and Chromeleon 7.0 Software; following what was
Table 1 Effect of biofertilizer alone and in dual inoculation with Rhizoctonia solani in common bean growth parameters.
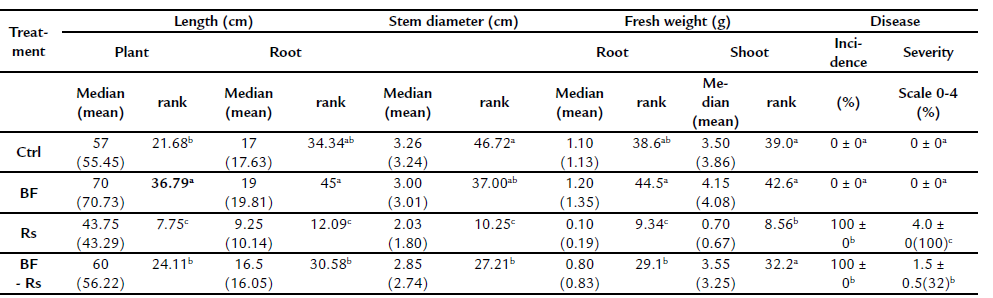
Ctrl= Control root, BF= biofertilized root, BF-Rs= biofertilized root combined with the pathogen Rhizoctonia solani, Rs= Rhizoctonia solani infected root. Different letters indicate significant statistical differences between treatments in each series, (p= 0.05), n = 16.
reported by Sandoval-Castro et al. (2017). The separation was performed with an Aclaim® 120 A C18 column (250 x 4.6 mm i.d., 5 µm particle size) (Thermo Fisher, New York, NY). The volume of injection was 10 µL and the mobile phase consisted of two solvent systems: A=water acidified with acetic acid (pH 2.8), and B= acetonitrile at room temperature. The gradient elution was: 95 % A to 5% B from 0 to 2.5 min; 10 to 12 % B from 2.5 to 6 min; 12 to 23 % B from 6 to 18 min; 23 to 35 % of B from 18 to 24 min; 35 to 95 % B from 24 to 30 min; and returning to the initial conditions (95 % A and 5 % B) at 40 min. The flow rate was 0.3 mL/min, and the fixed wavelengths were 260, 280, 300, 342, and 350 nm. The phenolic compounds were identified and quantified using phenolic standards (Sigma-Aldrich; St. Louis, MO, USA).
Statistical analysis
Two independent experiments were conducted for all analyses, using sixteen plants from each treatment. All data were subjected to normality tests; colonization rates were compared using Student's t-test (for two independent variables); the growth parameters were analyzed with the Kruskal-Wallis and Mann Whitney U tests; and ANOVA and a multiple ranges test (Tukey, p ≤ 0.05) were performed for phenolic assays using the Minitab v18 statistic program.
RESULTS
Colonization by the biofertilizer counteracts damage by R. solani infection
Percentages of mycorrhizal colonization in BF and BF-Rs treatments were 17.5 ±5.6 and 14.12 ±4.5 respectively, and no significant differences were found between them according to the Student's t-test. Non-inoculated treatments had no visible AMF colonization. Typical root and hypocotyl rot symptoms were observed in the pathogen treatment (Rs), and interestingly, the biofertilizer (BF) treatment counteracted the pathogen deleterious effects, by maintaining growth at the uninfected control level (Ctrl) (Table 1). Despite the similarity in disease incidence in the Rs treatment compared to the AMF co-inoculation with the pathogen (BF-Rs), the severity of the disease was reduced up to 68 % in this last treatment (Table 1).
Total phenolic content increased with mycorrhiza- pathogen interactions
Total phenolic content was measured, using both gallic acid, and catechin, as standards. Both sets of data are shown in Fig. 1. In the assay using gallic acid as a standard, no significant differences were found between Ctrl and BF treatments. However, in the assay using catechin as a standard, total phenolic content was statistically higher in BF than Ctrl. These data may indicate a slight, but important, difference in these compounds between Ctrl and BF treatments. On the other hand, a significant increase was found in the pathogen treatment (Rs) compared to the control. The highest increase in total phenolic content was observed in the co-inoculation treatment (BF-Rs), which was at least five-fold of gallic acid equivalents and three-fold of catechin equivalents (Fig. 1).
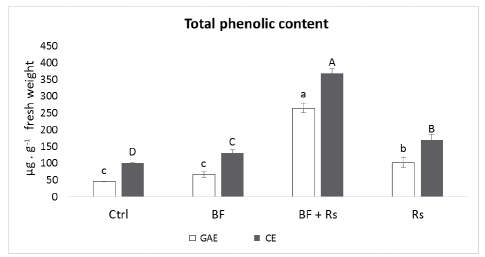
Fig. 1 Total phenolic compounds extracted from fresh root of common bean var. Azufrasin obtained from mycorrhiza-colonized and non-colonized plants grown under greenhouse conditions. Values are expressed as µg equivalents of gallic acid (GAE) or µg equivalents of catechin (CE) per g of root. Ctrl = control root, BF= biofertilized root, BF - Rs = biofertilized root combined with the pathogen Rhizoctonia solani, Rs = Rhizoctonia solani infected root. Different letters indicate significant statistical differences between treatments in each series, (p = 0.05); n = 16.
Mycorrhiza and pathogen treatments change the phenolic compound profile
A set of phenolic acids (Fig. 2a-2f) and flavonoids (Fig. 3a-3d) were evaluated in root extracts of plants subjected to the different treatments. Except for rutin, which did not show any difference compared to the control, the content of all the other phenolic compounds analyzed increased both in BF and Rs treatments, compared to the control. Individual analyses for each compound showed a high catechin concentration in the mycorrhiza treatment (BF) (Fig. 2a); while for caffeic acid, o-cumaric acid, kaempherol and quercetin, the highest accumulation was observed in the pathogen infection treatment (Rs) (Figs. 2a, 2c and 3b, 3c). Finally, vanillic acid was the only compound that showed a significant increase in its content in the co-inoculation treatment (BF-Rs) (Fig. 3f).
DISCUSSION
The use of natural resources, such as soil microorganisms, for their application in sustainable agricultural practices is currently gaining importance, even more when those microorganisms can improve plant nutrient uptake and help to cope with pathogen invasion. AMF are capable of increasing plant nutrient uptake and enhance the plant's defense against pathogens. Mycorrhiza-induced protection against pathogens has been widely reported in different plant species and pathogens, including the protection of the common bean against the shoot pathogen Sclerotinia sclerotiorum (Lib.) de Bary 1884 (Mora-Romero et al., 2015), and the rot root diseases caused by R. solani (Abdel-Fattah et al., 2011) and F. solani (Al-Askar and Rashad, 2010;
Eke et al., 2016). However, the defense response may vary according to the genotype of the plant species (Mora-Romero et al., 2015), pathogen (Hao et al., 2019), and AMF interaction (Pozo and Azcon-Aguilar, 2007; Eke et al., 2016). Abdel-Fattah et al. (2011) reported the enhancement of common bean seedling growth treated with an Egyptian mix of mycorrhizal fungi (containing equal proportions of G. mosseae, G. intraradices, G. clarum, and Gigaspora gigantea) against the pathogen R. solani, compared to uninfected plants. In this work, we tested a consortium of a Mexican AMF mix named Rizofermic-UV, which includes 12 species (A. morrowiae, A. scrobiculata, A. spinosa, C. etunicatum, F. geosporus, F. mosseae, G. decipiens, G. rosea, G. aggregatum, G. macrocarpum, R. intraradices, and S. pellucida) in common bean plants.
When plants were co-inoculated with the AMF consortium and the pathogen R. solani, despite the low rate of root colonization, a reduction of disease severity, up to 68 % was consistently observed (Table 1). Mora-Romero et al. (2015) also reported low-rate colonization (around 20 %) in mycorrhiza enhanced defense against the shoot pathogen S. sclerotiorum in common bean, suggesting that not full establishment of AMF is required in common bean to the induction of defense.
Regarding total phenolic content, the highest concentration was observed in plants infected with R. solani (Rs) compared to the control (Fig. 1). This is consistent with the previously reported fact that phenolic compounds have antimicrobial properties (Hammerschmidt, 2005; Mandal et al., 2010). On the other hand, with the BF treatment, total phenolic content showed a significantly higher concentration compared to the control. Beneficial microorganisms such as arbuscular mycorrhizal fungi, are known to induce a defense priming mechanism, which is a physiological state that prepares plants to react in a stronger and faster manner to a subsequent pathogen attack (Pozo and Azcon-Aguilar, 2007). The priming responses are believed to be discrete in magnitude, but consistent (Conrath, 2011). Thus, the slight increase in phenolic compounds in BF plants might be reflecting the onset of a defense priming state. Interestingly, the co-inoculation of the AMF consortium and the pathogen (BF-Rs treatment) showed a very strong accumulation of total phenolic compounds in roots compared to the control (Fig. 1). This suggests that the putative priming response triggered by mycorrhizal colonization and the response against R. solani interacted in a synergistic way, inducing a significant accumulation of total phenolic compounds (Fig. 1), which paralleled a decrease in disease severity (Table 1). Phenolic compounds could be participating as direct defense molecules, or as players in signaling pathways (Hammerschmidt, 2005; Mandal et al., 2010), which are influenced both by pathogens, as well as beneficial soil microorganisms like AMF (Al-Askar and Rashad, 2010; Abdel-Fattah et al., 2011; Eke et al., 2016; Sharma and Sharma, 2017). Changes in total phenolic compounds and flavonoids in the common bean interacting with different AMF and pathogens have been reported previously, e.g., enhanced biosynthesis and accumulation of total phenolic compounds were observed in AMF mix and R. solani co-inoculation (Abdel-Fattah et al., 2011), as well as in F. solani infection (Al-Askar and Rashad, 2010; Eke et al., 2016) and in the R. irregularis co-inoculated with the root-knot (Sharma and Sharma, 2017). Our results, considering the discrete phenolic compound accumulation induced by mycorrhiza inoculation (BF), and the boost in the tripartite interaction (BF-Rs), are consistent with these reports (Fig. 1).
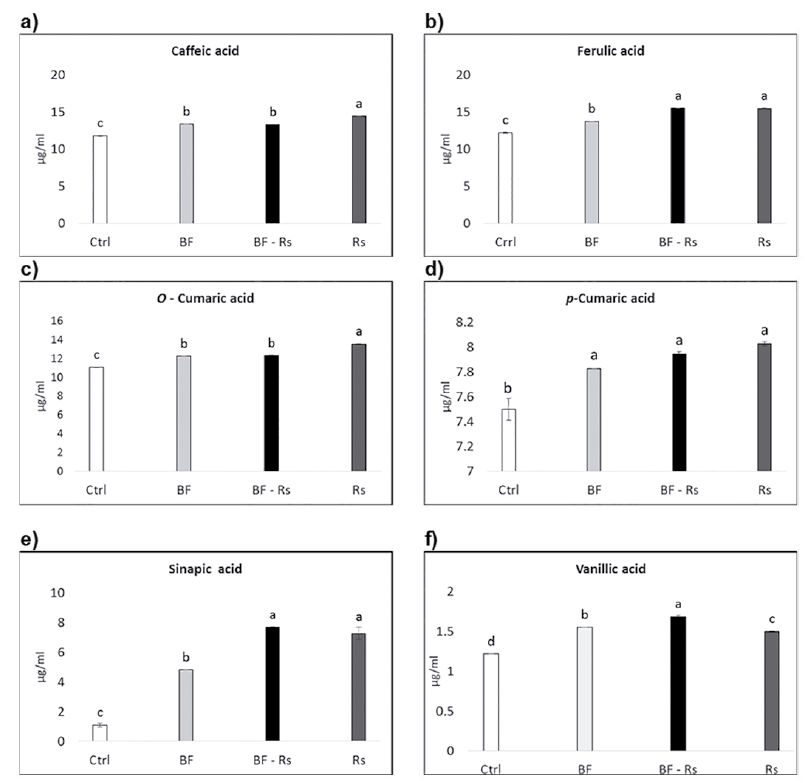
Fig. 2 Phenolic acid profile obtained by HPLC from ethanolic extracts of common bean var. Azufrasin roots. Ctrl = control root, BF= biofertilized root, BF - Rs = biofertilized root combined with the pathogen Rhizoctonia solani, Rs = Rhizoctonia solani infected root. Different letters indicate significant statistical differences between treatments, (p = 0.05); n = 16.
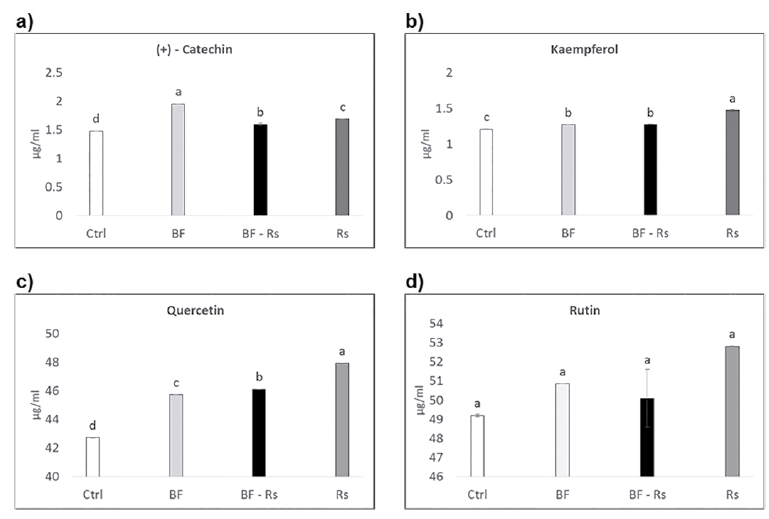
Fig. 3 Flavonoid profile obtained by HPLC, from ethanolic extracts of Azufrasin common bean roots. Ctrl = control root, BF= biofertilized root, BF + Rs = biofertilized root combined with the pathogen Rhizoctonia solani, Rs = Rhizoctonia solani infected root. Different letters indicate significant statistical differences between treatments, (p = 0.05); n = 16.
Several reports have been published on individual phenolic accumulation in mycorrhizal plants (Toussaint et al., 2007; Schliemann et al., 2008; López-Ráez et al., 2010). However, to the best of our knowledge, this is the first report on individual phenolic compounds in the tripartite interaction, plant-mycorrhizal fungi-pathogen. In this study we report the profile of a small group of phenolic acids (Fig. 2a-f) and flavonoids (Fig. 3a-d), most of which increased their concentration in common bean roots by AMF colonization. The flavonoid catechin showed a significant increase in roots inoculated with the biofertilizer, even more than in the pathogen treatment (Fig. 3a). Previously, catechin accumulation has been reported in very specific root areas in ectomycorrhizal colonized roots (Weiss et al., 1997). Whether this pattern of accumulation also occurs in the arbuscular mycorrhizal interaction remains to be investigated. However, the consistent high accumulation of catechin suggests a possible functional role for this metabolite in fungal symbiosis (Weiss et al., 1997). On the other hand, (+)-catechin accumulation has also been reported as a response to a fungal pathogen attack (Chakraborty and Saha, 1994). It is interesting, though, that the accumulation of (+)-catechin decreased in the dual inoculation treatment compared to the AMF inoculation alone. This suggests that the role of this compound is different in beneficial and pathogenic interactions.
Regarding individual phenolic metabolite content, such as the levels of caffeic acid, our results contrast with previous studies, e.g., our results show that caffeic acid content increased in the common bean by AMF inoculation (Fig. 2a), whereas previous reports show a decrease in AMF-colonized roots (Toussaint et al., 2007; López-Ráez et al., 2010).
In the context of the interaction between the plants and the pathogen R. solani, we observed a slightly significant accumulation of the phenolic compounds caffeic and o-cumaric acid, and of the flavonoids kaempherol and quercetin, with pathogen infection compared to AMF inoculation alone (Fig. 2a, 2c and 3b, 3c).
Caffeic acid and o-cumaric acid, both classified as hydroxycinnamic acids, are present in the cell walls. Caffeic acid has been related to biocide activity and plant pathogen resistance against Alternaria alternata (Fr.) Keissl. 1912 (Ruelas et al., 2006). O-cumaric acid is a biosynthetic precursor of salicylic acid, a signaling molecule that plays a role in host defense, and it has been reported to induce the expression of defense-related genes in plants (Montesano et al., 2005; Yang et al., 2008). The flavonoids kaempherol and quercetin act as deterrents against the nematodes Radopholus similis similis (Cobb) Thorne 1949 and Meloidogyne incognita (Kofoid and White) Chitwood 1949, and have shown anti-pathogenic activity (Mierziak et al., 2014). This suggests that these molecules, caffeic acid, o-cumaric acid, kaempherol and quercetin, are activated mostly by the pathogen-plant interaction.
The induction of ferulic acid in mycorrhizal roots has been previously reported (López-Ráez et al., 2010; Grandmaison et al., 1993). Consistently, in this study we observed a significant increase in ferulic acid with biofertilizer treatment alone compared to controls (Fig. 2b). Ferulic acid may be induced by fungal elicitors and jasmonates, suggesting that high levels of this compound may be involved in the onset of the priming state and contribute to the enhanced resistance of mycorrhizal roots to soil-borne pathogens (López-Ráez et al., 2010). High levels of ferulic acid during the pathogen infection were also observed, more than in the AMF treatment alone (Fig. 2b).
Sinapic acid is a common precursor of soluble secondary metabolites and has been detected in mycorrhiza-colonized onions roots (Grandmaison et al., 1993), and involved in seed germination and plant development, affecting ABA catabolism (Bi et al., 2017). Sinapic acid esters play a role in the defense response against pathogens (Konig et al., 2014). In the present work, a dramatic variation of sinapic acid was observed in roots after AMF inoculation, but the accumulation was even greater in the treatment with the pathogen and in the dual inoculation (Fig. 2e). This might be an indication that this compound has a major role in plant-microbe interactions.
Significant increase of vanillic acid content was observed in dual inoculations (Fig. 2f), even more than in AMF or pathogen treatment alone. Its precursor, vanillin, has been detected in ectomycorrhiza (Weiss et al., 1997). Also, the concentration of vanillic acid was shown to increase as part of the defense system during a pathogenic attack in tomato fruits (Ruelas et al., 2006). Thus, the increase of vanillic acid levels may contribute to the enhanced resistance of mycorrhizal roots to soil-borne pathogens, although this remains to be functionally confirmed.
The role of phenolic compounds in mycorrhiza-induced resistance is controversial, although accumulation of these compounds has been reported in AMF colonized roots, as well as in plant-pathogen interactions. Here, we also observed changes in total and individual profiles of these compounds, both in AMF, as well as in pathogen inoculation. The contribution of other phenolic compounds not analyzed in this work cannot be ruled out, and additional work is required to elucidate their role in these plant-microbe interactions.
CONCLUSION
Biofertilization based on a mix of arbuscular mycorrhizal fungi does not increase the root and shoot biomass but contributes to the reduction of the severity of the R. solani infection in the common bean var. Azufrasin plants. The enhanced defense against the pathogen observed in mycorrhizal treatment was accompanied by variations in total and individual phenolic compounds in the plants. This study provides evidence for the existence of a mycorrhiza-induced resistance mechanism in plants and suggests a possible participation of phenolic compounds.