INTRODUCTION
Blueberry is a new crop introduced in Mexico, and during the last decade (2010-2020), the production area and consumption of blueberries have increased (SIAP, 2019). A greater understanding of the health benefits of antioxidants, as well as increased public awareness of blueberries as a superfood that contains high levels of naturally occurring antioxidants (Fulcher et al., 2015), fueled this increase. However, meeting current food demands requires production in a sustainable and low-input agricultural system (Mezzetti, 2016). In particular, sustainability strives to preserve the ecological integrity of areas with complex ecosystems that include a wide variety ofbacteria, fungi, protists, and animals (Chaparro et al., 2012; Muller et al., 2016). The rhizosphere supports microbial populations that play a vital role in root health maintenance, nutrient uptake, and environmental stress resistance, thus affecting plant growth (Ahmed and Khan, 2011). Among these microorganisms, plant growth-promoting rhizobacteria (PGPR) improve plant growth through the production of phytohormones, siderophores, antibiotics, enzymes, and fungicidal compounds (Seleim et al., 2011; Saharan and Nehra, 2011; Alizadeh et al., 2012). Bacterial species such as Pseudomonas, Azozpirillus, Azobacter, Klebsiella, Enterobacter, Alcaligenes, Burkholderia, Bacillus, and Serratia enhance plant growth (Wahyudi et al., 2011; Kumar et al., 2012; Rojas et al., 2016).
Fluorescent pseudomonads form a functional group that comprises Pseudomonas species and produce a greenish fluorescent compound known as pyoverdine, a siderophore (Cézard et al., 2015). Some fluorescent pseudomonads directly influence plant growth and seed emergence or improve crop yield; thus, these are often referred to as biofertilizers (Glick et al., 2012).
The production of phytohormones by P. fluorescens is one of the main mechanisms of plant growth promotion. Some of the phytohormones produced are abscisic acid (ABA), cytokinins (CKs), gibberellins, and indole-3-acetic acid (IAA) (Egamberdieva, 2009; Hernandez et al., 2015; Verma et al., 2015a). IAA plays an essential role in developmental and physiological plant processes such as embryogenesis, organogenesis, vascular differentiation, root and shoot development, trophic growth, and fruit development (Etchells et al., 2016). Other mechanisms of plant growth promotion include the reduction of ethylene levels in the plant through ACC deaminase action (Saleem et al., 2007) and the increase uptake of nutrient by the plant by, for example, increasing phosphorus uptake through the solubilization of inorganic phosphates (Jha and Saraf, 2015; Goswami et al., 2016). Phosphorus (P) is the second most important nutrient for plants (Hariprasa and Niranja,2009), and the use of plant growth promoting bacterial, such as P. fluorescens, could be an alternative to improve P uptake, being an ecofriendly option of chemical fertilization (Oteino et al., 2015).
Ortiz et al. (2018) studied the diversity of endophytic bacteria associated with blueberry plants, var. Biloxi and found a higher population density of endophytic bacteria in roots than in stems and leaves; the most abundant genera were Pantoea, Pseudomonas, Burkholderia, and Bacillus. Bacillus and Pantoea produced higher quantities of phytohormones (IAA), biofilms, and siderophores. However, their particular plant growth-promoting effects need to be studied. Symbiotic relationships are very important in blueberry plants because they have very fine and highly branched, fibrous roots without root hairs, highly branched, with the finest roots reaching 20 µm in diameter (Valenzuela-Estrada et al., 2008). The lack of root hairs on blueberry roots is a serious disadvantage for survival, especially in the absorption of P, which is an immobile nutrient. The current study examined the growth of blueberry plants inoculated with four strains of P. fluorescens (UM16, UM240, UM256, and UM270) under greenhouse conditions in combination with two slow-release fertilizers (Basacote Plus and nitrophosphate). The results obtained in this study could be important in sustainable organic blueberry production.
MATERIALS AND METHODS
Biological material
The experimental work was carried out on blueberry seedlings (Vaccinium sp., var Bilox) obtained from in vitro culture. Before use, the seedlings were acclimated for 40 days under greenhouse conditions. Four P. fluorescens strains were also acclimated and were identified as UM16, UM240, UM256, and UM270 (Hernández et al., 2015). These strains were isolated from Medicago sativum at Ciénega in Morelia, Michoacán, México.
The plants were sown in polypropylene trays with 60 cells, each with a volume of 220 mL. The trays were filled with a mixture of peat moss and perlite (50:50). Subsequently, 85 blueberry seedlings between 5 and 6 cm long were planted in the cells, and their root length and plant height were recorded.
Bacterial growth conditions
Bacteria were grown in potato dextrose (PD) liquid medium (BD Bioxon®), the pH was adjusted to 7, and the volume was adjusted to 1000 mL with distilled water. The mixture was sterilized in an autoclave for 16 min at 121 °C. Inoculation of bacteria in the PD medium was carried out in a laminar flow hood under sterile conditions. With a bacteriological loop, bacteria were taken from the Petri dish and placed in 300 mL of medium. Subsequently, the flask containing the inoculated medium was agitated for 24 h at 30 °C and 150 rpm in an orbital shaker.
Fertilization and inoculation of plants
After the plants were inoculated (Hernández et al., 2015; Rojas et al., 2016), they were fertilized with nitrophosphate 12-12-17 (+2 Mg, + 20 S) (12 units of nitrogen, 12 units of phosphorus, and 17 units of potassium plus two units of magnesium and 20 units of sulfur) or with Basacote Plus 3M 16-8-12 (+2) (16 units of nitrogen, eight units of phosphorus, and 12 units of potassium plus two units of magnesium), except for the control. For each plant, 0.2 g of fertilizer was used, since previous tests showed that higher amounts of fertilizers were toxic. Every ten days for 30 days, each plant was reinoculated with 10 mL of bacterial suspension contained each strain (1 x 10-6 CFU mL-1). Data collection was performed 90 days after the last inoculation. Non-inoculated or non-fertilized plants were used as absolute controls.
Experimental design and statistical analysis
The experimental design was completely random, with a factorial arrangement of treatments. In a first experiment plants were inoculated with one of the following P. fluorescens strains: UM16, UM240, UM256, and UM270 strains. After that, another independent experiment was established where the plants were fertilized with nitrophosphate or basacote and inoculated one, two or three times with one of the four strais. Each experiment had control plants that were irrigated with water. The treatments contained five plants, and each plant was an experimental unit. Every experiment was duplicated. The statistical package SAS version 2008 was used for data analysis. Treatments that showed significant differences after an ANOVA were analyzed using the Tukey test (p £ 0.05) or Fisher's minimum significant difference test. Data were collected for the following variables: plant length (cm), plant fresh and dry weight (g), root length (cm), and root fresh and dry weight (g). To evaluate plant and root dry weight the tissues were put in a paper bag and placed in an oven at 50 oC for 36 hours; after that, they were weighed on an analytical balance.
RESULTS
Effect of P. fluorescens in combination with nitrophosphate or basacote fertilization on plant length
Blueberry plants inoculated with P. fluorescens were longer than the non-inoculated control (6.1 cm, average length), whereas plants inoculated with the UM16 strain had an average length of 7.5 cm. Plants inoculated with strains UM256, UM240, and UM270 averaged 7.2, 7.1, and 6.5 cm, respectively (Fig. 1a). Plants inoculated up to three times with the different strains and fertilized with nitrophosphate (N) grew more vigorously than either control plants or inoculation-only plants; the average lengths of the N1, N2, and N3 plants were 9.8, 9.9 and 9.6 cm, respectively. Plants fertilized with Basacote (B) and inoculated once (B1) had an average length of 7.6, while the length for both B2 and B3 was 8.1 cm (Fig. 1b). Inoculation-only plants were, on average, 7.1 cm long.
Effect of P. fluorescens in combination with nitrophosphate or basacote fertilization on fresh weight
Plants inoculated with strain UM16 had an average weight of 1.04 g, while those inoculated with strains UM240, UM270, and UM256 weighed 0.95, 0.89, and 0.76 g, respectively. Control plants had an average fresh weight of 0.4 g (Fig. 1c). The number of inoculations affected plant fresh weight. Plants fertilized with nitrophosphate and inoculated once (N1) accumulated the highest fresh weight (1.5 g plant-1); these plants were more vigorous and had a higher number of shoots. N3 and N2 plants weighed 1.2 and 1.1 g plant-1, respectively, while B1, B2, and B3 plants weighed between 0.71 and 0.73 g. These results were significantly higher than those for plants inoculated with bacteria or control plants, which had fresh weights of 0.25 g and 0.04 g, respectively (Fig. 1d; Fig. 5).
Effect of P. fluorescens in combination with nitrophosphate or basacote fertilization on root length
Plants inoculated with bacteria had longer roots than non-inoculated plants (control). The plants inoculated with strain UM16 had average lengths of 9.92 cm, while those inoculated with UM256, UM240, or UM270 were 9.20, 9.16 and 9.05 cm long, respectively. In comparison, control plants had a length of 6.57 cm (Fig. 2; Fig. 5). On the other hand, the N1, N2, N3, B1, B2, and B3 plants had root lengths between 9.5 and 9.8 cm, unlike the only inoculated plants and the controls (Fig. 2).
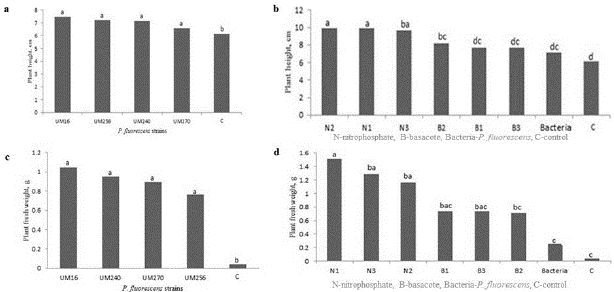
Figure 1 Effect of Pseudomonas fluorescens UM16, UM240, UM256 and UM270 strains on the length (upper) and fresh weight (lower) of blueberry plants var. Biloxi only inoculated (a and c) or fertilized with nitrophosphate (N) or basacote (B) and inoculated one, two or three times (b and d). C shows the control. Values with the same letter are not significantly different (Tukey, P>0.05).
Effect of P. fluorescens in combination with nitrophosphate or basacote fertilization on fresh root weight
Blueberry plants inoculated up to three times with any of the bacterial strains had higher root fresh weights than uninoculated plants; the values were 0.784, 0.754, 0.6979, and 0.860 g for strains UM16, UM240, UM256, and UM270, respectively, while the control weighed only 0.2470 g (Fig. 3). Likewise, root fresh weights of plants inoculated up to three times and treated with nitrophosphate (N1, N2, N3) or Basacote (B1, B2, B3) were higher than that of the inoculated plants and the control plants. The N1, N2, and N3 plants had root fresh weights of 1.1133, 0.9874, and 0.979 g, respectively. These values were higher than those obtained for Basacote-fertilized and inoculated plants: 0.6752 g for B1, 0.6133 g for B2, and 0.4424 g for B3 (Fig.3).
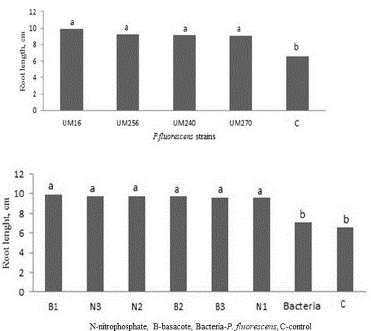
Figure 2 Root length of blueberry plants var Biloxi inoculated with Pseudomonas fluorescens UM240, UM270, UM256 and UM16 strains (upper) or fertilized with nitrophosphate (N) or basacote (B) and inoculated one, two or three times (lower). C shows the control. Values with the same letter are not significantly different (Tukey, P>0.05).
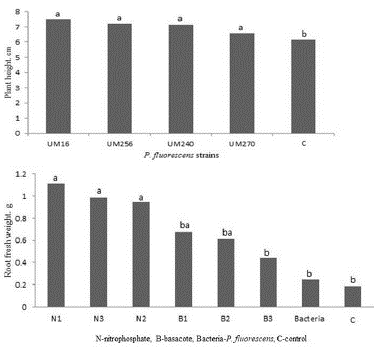
Figure 3 The fresh root weight of blueberry plants var Biloxi inoculated with Pseudomonas fluorescens UM240, UM270, UM256 and UM16 strains (upper) or fertilized with nitrophosphate (N) or basacote (B) and inoculated one, two or three times (lower). C shows the control. Values with the same letter are not significantly different (Tukey, P>0.05).
Effect of P. fluorescens in combination with nitrophosphate or basacote fertilization on shoot and root dry weights of plants
The dry weights of the roots and aerial parts were significantly different among the blueberries inoculated with each of the P. fluorescens strains and the inoculated and fertilized blueberries (Fig. 4). Plants inoculated with strains UM16 and UM240 and fertilized with nitrophosphate had higher aerial dry weights, of 1.05 g and 0.82 g, respectively (Fig. 4, left, W/N), than plants inoculated with strains UM256 and UM270 and fertilized with nitrophosphate or Basacote (Fig. 5, W/N, and W/B). Only inoculated plants had lower dry weights (Fig. 4, left, C). In terms of root dry weight, inoculation had a visible effect because root dry weight of plants inoculated with strain UM240, UM256, or UM270 (Fig. 4, right C) were not significantly different from those of inoculated plants fertilized with nitrophosphate (Figure 4, right, W/N). Plants with the lowest root dry weight were, for the most part, those inoculated and fertilized with Basacote (Fig. 4, right, W/B).
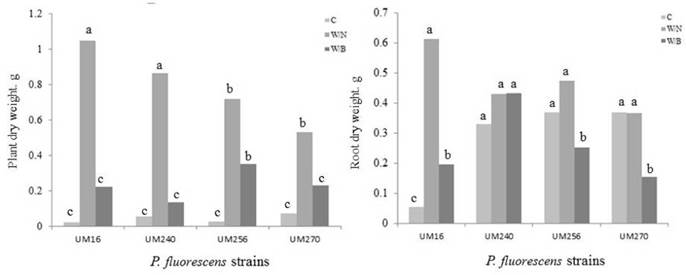
Figure 4 Dry weight of the aerial parts (left) and roots (right) of blueberry plants var. Biloxi inoculated with Pseudomonas fluorescens UM240, UM270, UM256 and UM16 strains or inoculated and fertilized with nitrophosphate (W/N) or basacote (W/B). Values with the same letter are not significantly different (Duncan, P>0.5).
DISCUSSION
P. fluorescens strains UM16, UM240, UM256, and UM270 had a positive effect on the root and aerial growth of blueberry plants grown under greenhouse conditions, and fertilization with high-phosphorus fertilizer facilitated this effect. Our results are similar to those observed by Hernández et al. (2015), who reported a weight increase in shoots and roots in Medicago truncatula plants inoculated with strains UM240, UM256, and UM270. However, this study found that for blueberry, inoculation with any of the P. fluorescens strains caused an increase in both root and shoot lengths when compared to those of the control plants.
Blueberry plants inoculated with P. fluorescens strains had higher root fresh weight, in comparison with the control. Similar results have been reported by Kirankuman et al. (2008) and Kumar-Mishra et al. (2010), who observed that the genus Pseudomonas significantly improved the plant growth of tomato plants by recording higher values for height, fruit yield, and nutrient absorption. Pseudomonas spp. stimulate plant growth by IAA production (Patten and Glick, 2002; Prasad et al., 2015). However, the range of beneficial effects provided by IAA is very narrow, and high concentrations of IAA can be detrimental to plants (Suzuki et al., 2003).
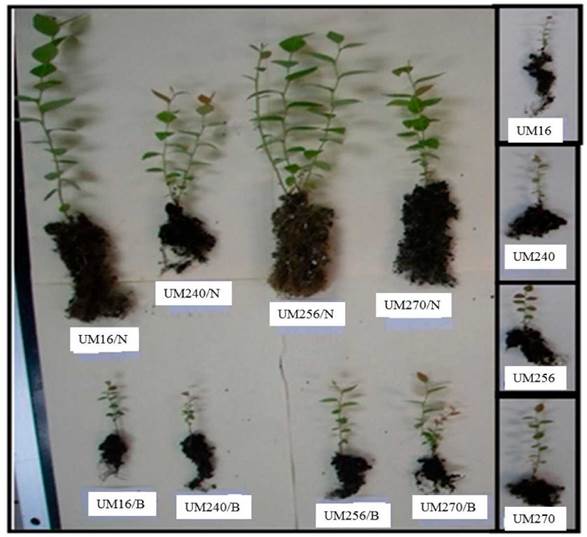
Figure 5 Blueberry var Biloxi plants inoculated with Pseudomonas fluorescens UM216, UM240, UM256, and UM270 strains and fertilized with nitrophosphate (strain/N) or basacote (strain/B) or only inoculated with each bacteria (strain/C).
Some bacterial genera, such as PGPR rhizobacteria, are capable of promoting plant growth through a wide variety of mechanisms (Santoyo et al., 2012). Beneficial effects ofPGPR include facilitation of nutrient uptake from the soil through the synthesis of specific compounds (Hayat et al., 2010). P. fluorescens has significant effects on the development and production of other crops, including beans. In the presence of phosphorus (25 kg ha-1), inoculation with strain 168 led to an increase in grain yield of 42% to 65% compared to that non-inoculated plants. These results showed that strain 168 increases yield (Ghasempour and Aminpanah, 2015). The inoculation of soils with P. fluorescens, in combination with fertilizers, plays an essential role in the stimulation of chickpea growth (Rokhzadi et al., 2008). Such actions considerably increase cane fresh and dry weight (Mehnaz et al., 2009) and promote higher yields in legumes (Johri, 2001).
Other studies have shown that direct inoculation of each Pseudomonas strain (UM16, UM240, UM256, and UM270) on in vitro plants of M. truncatula led to significant increases in root weight as well as high concentrations of chlorophyll (Hernández et al., 2015). Kimar et al. (2015) observed that tomato seeds and plants inoculated with Pseudomonas sp. LPN4 showed significant increases in seed germination (83.3%), root (0.438 g), and shoot (0.118 g) dry weight.
The most significant effect was observed in the root dry weight of blueberry plants, as bacterial inoculation promoted root development similar to that of plants additionally fertilized with nitrophosphate. Pseudomonas can fix nitrogen and solubilize phosphate much better than other rhizobacteria, thereby increasing root weight. Phosphorus is an essential macronutrient for plant growth and development (Jha and Saraf, 2015). Phosphorus is inaccessible to plants in its natural state (Goswami et al., 2016). Thus, plant growth-promoting microbes must convert the insoluble forms of phosphorus (inorganic and organic forms) to available forms to promote plant yield (Jha and Saraf, 2015).
It is important to note that most wild blueberry species have adapted to low-fertility, sandy, acidic soils in North America. The symbiotic relationship between blueberry roots and mycorrhizal fungi and many ecto- and endophytic bacteria that provide a wide range of nutrients to plants promote this adaptability (Bonfant and Anca, 2009; Ortiz et al., 2018).
CONCLUSIONS
P. fluorescens has a significant influence on the development of roots and foliar system of blueberry var. Biloxi. Significant differences due to the type of strain inoculated as well as the type of fertilizer were observed. This effect was enhanced when plants were inoculated and additionally fertilized with nitrophosphate; plants in this treatment showed improved growth compared to those treated with Basacote fertilization. The number of bacterial inoculations had no significant effect on the measured parameters. P. fluorescens is an alternative for soil enrichment that promotes efficient phosphorus fixation and the sustainability of agricultural production systems.