Services on Demand
Journal
Article
Indicators
-
Cited by SciELO
-
Access statistics
Related links
-
Cited by Google
-
Similars in SciELO
-
Similars in Google
Share
Ingeniería e Investigación
Print version ISSN 0120-5609
Ing. Investig. vol.33 no.2 Bogotá May/Aug. 2013
O. M. Martínez A.1, J. H. Ramirez F.2 and M. L. Toledo R.3
1 Oscar Mauricio Martínez Ávila. Ingeniero Químico, Universidad Nacional de Colombia, Colombia. MSc. Ingeniería Química, Universidad Nacional de Colombia, Colombia. Affiliation: Universidad Nacional de Colombia - Sede Bogotá, Colombia. E-mail: ommartineza@unal.edu.co
2 José Herney Ramírez Franco. Ingeniero Químico, Universidad Nacional de Colombia, Colombia. MSc. Chemical Engineering, Universidad de Sao Paulo, Brasil. PhD Chemical Engineering, Universidad do Porto, Portugal. Affiliation: Universidad Nacional de Colombia, Colombia. E-mail: jhramirezfra@unal.edu.co
3 Magda Lorena Toledo Reyes. lngeniera Química, Universidad Nacional de Colombia, Colombia. Affiliation: Universidad Nacional de Colombia, Colombia. E-mail: mltoledor@unal.edu.co
How to cite: Martínez, O. M., Ramírez, J. H., Toledo, M. L., Total organic carbon removal from a chemical lab's wastewater using Fenton's reagent., Ingeniería e Investigación. Vol. 33, No. 2. August 2013, pp. 30 - 35.
ABSTRACT
Treating industrial wastewater represents a serious problem nowadays; it requires a strong understanding of the particular systems and (in most of cases) ad hoc solutions. This work describes the use of Fenton's reagent (reaction between H2O2 and Fe(II)) for removing total organic carbon (TOC) from a particular chemical laboratory's lab-scale batch reactor wastewater. Some operating variables (hydrogen peroxide and ferrous ion concentration, temperature and pH) were evaluated regarding final TOC removal. An economic optimisation was made by means of a second order polynomial model representing these variables' behaviour regarding TOC removal (0.94 R2). The highest experimentally reached TOC removal was 88.8% at 50 mg/L [Fe(II)]0, 50 mM [H2O2]0 , pH=2.8 at 80oC, while 53.9% was obtained in optimised conditions, i.e. 36 mg/L [Fe(II)]0 , 45.5 mM [H2O2]0 , pH=2.6 at 20oC. It was found that the Fenton process could achieve 41% removal, even in adverse conditions (pH close to 6). It was noted from the analysis that both H2O2 concentration and temperature had a powerful effect on organic matter degradation efficiency, as well as on total treatment cost.
Keywords: Fenton's reagent, advanced oxidation, laboratory wastewater, TOC removal.
RESUMEN
El tratamiento de efluentes residuales industriales representa un serio problema en la actualidad, ya que, por lo general, requiere de soluciones particulares (ad hoc). Este trabajo describe el uso del reactivo Fenton [reacción entre H2O2 y Fe(II)] en la remoción de materia orgánica medida como carbono orgánico total (COT), sobre un efluente proveniente de un laboratorio de análisis químico. Para dicho fin se evaluó el papel que desempeñan algunas de las condiciones de operación del proceso [concentraciones de H2O2 y Fe(II], temperatura y pH] sobre la remoción final de COT del efluente. Al mismo tiempo se desarrolló la optimización económica del tratamiento, ajustando los datos experimentales a un modelo multivariable polinomial de segundo orden que representa el comportamiento del sistema con un R2 de 0.94. Experimentalmente, la mejor remoción encontrada fue de 88,8%, empleando [Fe(II)]0 = 50 mg/L; [H2O2]0 = 50 mM; pH = 2,8 y T = 80 oC, mientras que en el óptimo económico ([Fe(II)]0 = 36 mg/L; [H2O2]0 = 45,5 mM; pH= 2,6 y T = 20 oC) se alcanzó un 53,9%. Por otra parte, se evidenció que incluso en condiciones adversas para el reactivo Fenton (pH sobre 6) se consiguió una remoción de COT del orden de 41%. Se encontró que la concentración de H2O2 y la temperatura de reacción tienen los efectos más significativos tanto en la eficiencia del proceso como en el costo de operación, lo que las convierte en variables a priorizar en la ejecución del tratamiento.
Palabras clave: reactivo Fenton, procesos avanzados de oxidación, agua residual laboratorio, remoción de COT.
Received: May 10th 2012 Accepted: May 28th 2013
Introduction
Water contamination continues having an impact on the environment; water-born effluent comes from a large variety of chemical industries, containing organic components such as phenol, alcohol, benzene, ether and chlorinated aromatic derivatives at differing concentration, all of them being toxic and dangerous when they are released into the environment. Society's growing demand for water decontamination has been reflected in stricter regulations, driving the development of new depuration technologies during the last few years.
An explicit example in this field would be chemical waste produced by academic and commercial laboratories; such waste is a by-product of research, teaching and testing. It can be considered as producing the most polluted wastewater and, at the same time, could afford the highest challenges for treatment and disposal due to its inherent characteristics (Benatti et al, 2006). Its constituents may include waste from chemical, biological and/or microbiological experiments involving a wide range of synthetic and natural samples. Heavy metals, high levels of organic matter, low pH conditions and a variety of non-quantified harmful substances are common in such wastewater.
The main techniques used for decontaminating laboratory wastewater today involve physical processes. However, these processes may be costly, inefficient and tend to produce an excessive volume of secondary waste (Metcalf & Eddy, 2003). Ozone and hypochlorite oxidation represent effective methods but they are not desirable because of high operating costs, equipment cost and the concomitant pollution arising from the residual chlorine (Malik & Saha, 2003). Such waste is usually disposed of via sewers carrying municipal waste without having any type of treatment.
A particularly relevant alternative for dealing with difficult effluent involves using advanced oxidation processes (AOPs) where organic pollutants are transformed into harmless compounds, CO2 and water by oxidation. Fenton's reagent represents an example of an AOP; it has proved to be a promising and attractive treatment method for effectively destroying a wide variety of dangerous organic pollutants (Lin & Lo, 1997; Dutta et al., 2001; Lee & Shoda, 2008; Bianco et al., 2011). The process is straightforward and inexpensive, taking place at low temperatures and atmospheric pressure (Bigda, 1995). It consists of a reaction between ferrous iron and H2O2 and is based on generating hydroxyl radicals (•OH) which are highly reactive (standard potential: 2.8 eV); these hydroxyl radicals are formed by catalytic decomposition of H2O2 in an acid environment (equation 1) (Walling, 1975):

The by-product, ferric ions, can react with hydrogen peroxide or superoxide radical (O2-) to regenerate the ferrous iron as shown below:

All three previous reactions constitute the iron cycle among states of ferrous and ferric oxidation, whereas H2O2 becomes entirely consumed thereby producing hydroxyl radical (Neyens & Baeyens, 2003). These ·OH radicals can non-selectively oxidise a variety of organic compounds having high reaction rates. This property can be used for the complete mineralization of a pollutant or its degradation into less harmful substances. The main advantage of Fenton's reagent is that its components are easy to handle, are environmentally-friendly and cheaper than other oxidants (Kavitha & Palanivelu, 2004). However, experimental conditions should be optimised to ensure suitable performance because many variables can affect pollutant removal effectiveness, and even more so due to the presence of a wide variety of contaminants.
Fenton´s reagent has been employed over real wastewater decontamination for treating unusual types of pollutants, obtaining different results in each case. For instance (Swaminathan et al, 2003) and (Rodrigues et al, 2009) have used Fenton process for decolorizing water, finding color removal close to 99%. At the same time, (Bautista et al, 2007) treated cosmetic wastewater optimizing Fenton´s reagent achieving 60% of total organic carbon (TOC) removal. Some other authors have used Fenton process for decontaminating complex wastewaters like (Martínez et al, 2003), (Badawy & Ali, 2006) and (Méndez et al, 2010) with decontamination percentages of 56% up to 90% in terms of chemical oxygen demand (COD). (Lucas & Peres, 2009) also employed Fenton´s oxidation for improving the quality of olive mill wastes reaching 70% of COD removal. With respect to laboratory wastewaters, (Benatti et al, 2006) assessed Fenton reagent in a decontamination process, optimizing the oxidation process with a 92% of COD removal, but they required pretreatment for improving the effectiveness of overall treatment.
In spite of the numerous applications for Fenton's reagent, little research has been done into its effect regarding laboratory wastewater. In fact, understanding is superficial regarding the multiple interactions amongst heavy metals, solids and organic material involved in oxidation. Furthermore, economical assessment of the treatment seems to be a negligible component in most of the previous studies. This work thus involved treatment performance being evaluated through experiments and economic optimisation based on a system's actual requirements and particular constraints.
Materials and Methods
Wastewater samples
Wastewater was obtained from the Regional Autonomous Corporation of Cundinamarca's Environmental Lab's treatment plant in Bogotá, Colombia. Samples were taken using composite sampling over a 6-hour period and then stored at 4oC in the dark immediately after this procedure. The mixed, composite sample (used in oxidation experiments) was first characterised in terms of some parameters according to local policies as well as final effluent after treatment. Table 1 shows these samples' characterisation.
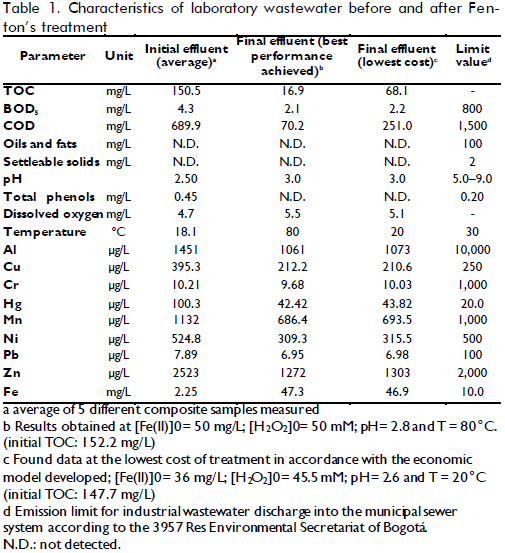
Experimental procedure
Chemical oxidation of the wastewater samples took place in a 0.5 L batch jacketed glass reactor (0.25L sample size). A thermostatic bath was used for temperature control and the reactor was continuously stirred at 200 rpm with magnetic bar. Oxidation was carried out as follows: pH was adjusted to the appropriate value (2 to 6) by adding sulphuric acid (H2SO4, 1N) and sodium hydroxide (NaOH, 1N), followed by setting the temperature through the thermostatic bath. Solid iron sulphate (FeSO4.7H2O from Merck) was then dissolved and hydrogen peroxide solution (30%, w/w from Merck) was added. Time zero coincided with adding the total volume of H2O2 and experiments were run up to 90 min. Most experiments were repeated at least twice and the average relative error regarding final TOC removal in all repeated runs was 9.5%.
Experimental design and statistics
A second order polynomial was considered in the present work as the model for describing oxidation data; it has been used in previous work (Ramirez et al., 2005; Benatti et al., 2006) for optimising process variables for maximising pollutant removal. The mathematical model was used in the economic evaluation, taking four measured variables into account: H2O2 and Fe(II) concentration, temperature and pH. These natural Xi variables were converted into dimensionless codified xi values for statistical calculation, using equation (4) to allow comparing different factors to different units and reduce error regarding polynomial fit:

The experimental results for response yi (TOC removal measured as a conversion, XTOC) were then fitted to a second order polynomial model (5) that took linear and quadratic effects of n variables into account as well as interactions amongst them:

where xi referred to codified variables derived from XTOC values, β0, βi, βii and βij represented the parameters in the second order model (β0 intercept term, βi linear term, βii quadratic effects, βij interactions among variables). General algebraic modelling system (GAMS) software was used for calculating the corresponding coefficients, using global optimisation for least-squares fitting.
Analytical methods
TOC was measured by selective catalytic oxidation followed by IR spectrometry for CO2 quantification using a SKALAR Formacs HT/Analyzer which has an automatic sample injector. TOC values ??were the average of at least two measurements. Samples were taken from the reactor at 10 minutes intervals for each analysis; the reaction was stopped by adding excess solid sodium sulphite (Na2SO3) which instantaneously consumed the remaining H2O2. COD, BOD5 (Biochemical oxygen demand), settleable solids, oils and fats, pH, temperature and dissolved oxygen were performed in accordance with (Standard methods, 2005). COD was measured with a Spectroquant TR 320 reactor and metals concentrations (Al, Cu, Cr, Hg, Mn, Ni, Pb, Zn) were characterised by inductively coupled plasma mass spectroscopy (ICP-MS) according to EPA guidelines (1994) using a Varian 820-MS. Iron concentration (Fe) was determined by atomic absorption spectroscopy using a UNICAM 989.
Results and Discussion
TOC removal was chosen as a target parameter for establishing appropriate conditions for the aforementioned operational variables because, although wastewater has seasonal characteristics according to laboratory work, TOC content tends to show less variation over large periods of sample collection. TOC has the advantage of advising directly on the degree of mineralization, namely, complete oxidation to CO2 and H2O, which undergoes the organic matter present in the wastewater. Table 2 shows the oxidation experiments carried out with Fenton's reagent in wastewater samples. It should be noted that ranges were chosen based on the authors' previous results (Ramirez et al., 2005) and findings in the pertinent literature (Swaminathan et al., 2003; Malik & Saha, 2003; Lucas & Peres, 2009).
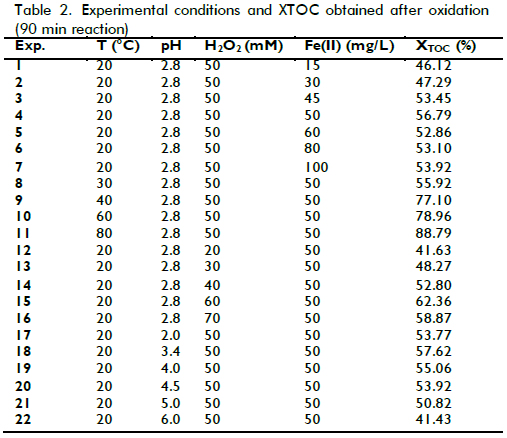
TOC conversion by Fenton reaction after 90 minutes' oxidation was 41.4% to 88.8% depending on the operating conditions. This TOC data was directly related to organic matter content in samples; XTOC therefore represents these compounds degree of mineralization to CO2 and water. Despite the calculated conversion, smaller intermediate, less contaminating molecules still affected TOC measurement.
Effect of initial pH
The in?uence of initial pH on the mineralization of laboratory wastewater was first studied, Figure 1 shows that when the initial pH increased from 2 to 2.8, the XTOC value rapidly increased, decreasing drastically when pH was raised from 2.8 to 3.4 and when values were above 2.8. This pattern had been previously observed by the authors (Ramirez et al., 2005) and agreed with the literature as it is generally accepted that acid pH levels close to 3 are the optimum for Fenton oxidation (Walling, 1975).
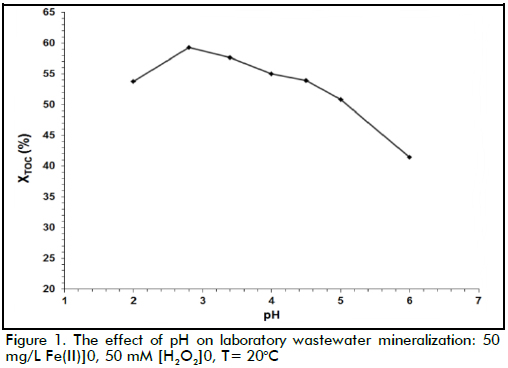
The process becomes less effective at pH<3. Indeed, Fe(II) regeneration in such conditions (via reaction between Fe(III) and H2O2) becomes inhibited due to a reduction in formation of Fe(II)-peroxo-complexes (as intermediates) (Pignatello, 1992). Performance signi?cantly decreased at pH above 3.4, mainly because the dissolved fraction of iron species also decreased (Pera-Titus et al., 2004). Fe(III) precipitates at high pH values thereby decreasing the concentration of dissolved Fe(III). Consequently, Fe(II) species' concentration also decreased because Fe(III) hydroxides are much less reactive towards H2O2 than dissolved Fe(III) species. Despite this pattern, TOC removal was around 41% at pH 6.0, meaning that Fenton's reagent worked properly in this kind of wastewater, even in adverse conditions.
The effect of temperature
The effect of temperature on laboratory wastewater mineralization can be observed in Figure 2, where XTOC was obtained at five different temperatures. Figure 2 clearly shows that the reaction rate increased when temperature increased, this being expected due to the exponential dependency of the kinetic constants on reaction temperature (Arrhenius' law). It should be noted that TOC removal was close to 77% at 40oC and around 79% at 60oC. Although lower than that obtained at higher temperatures, TOC removal values achieved at 40oC might be considered satisfactory, taking into account that lower temperatures might have reduced process costs.
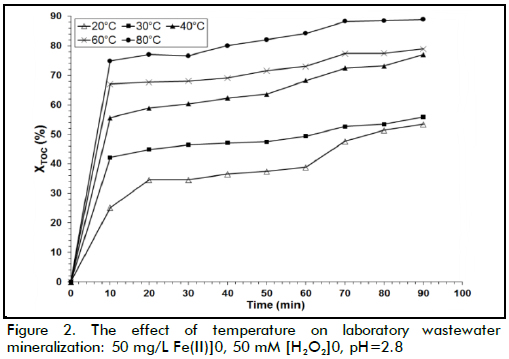
The effect of Fe(II) concentration
The above procedure was also applied when analysing iron concentration effect on XTOC (Figure 3). The data showed that the degradation rate was acutely sensitive to iron concentration because it acted as a catalyst in the Fenton reaction. An increase in Fe(II) load implied that more •OH radicals were available for oxidation. When the iron concentration changed from 15 to 50 mg/L, the reaction rate increased significantly but, when higher iron loads were used (i.e. 80 mg/L), the reaction rate decreased. However, final TOC conversion was remarkably similar in the three cases, being around 50% TOC removal. The best XTOC value was achieved at Fe(II) 50 mg/L. When higher Fe(II) concentrations were used, the efficiency of the reaction decreased; excess catalyst may, however, have led to a loss of •OH species by the following scavenging reaction (Walling, 1975):

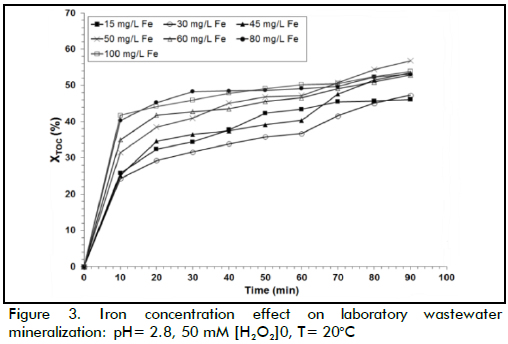
TOC removal took place in two stages with Fenton's reagent regarding time (Figure 2 and 3); organic matter was rapidly degraded during the first stage (Fenton stage) where iron was mainly Fe(II). The second stage (Fenton-like stage), where iron was mostly Fe(III), produced oxidation at a slower rate. Figure 3 shows how oxidation occurred faster during the first 30 minutes of reaction and then degradation slowed down. This pattern was mainly due to ferrous ions reacting quickly with hydrogen peroxide (k1≈ 70 M-1 s-1) (Neyens & Baeyens, 2003) to produce large quantities of hydroxyl radicals, as can be seen in equation (1), consequently reacting rapidly with organic matter. Fe(II) regeneration was clearly slower than spreading reaction rate (equation 1) based on the reaction mechanism described in equations 2 and 3, due to its low constant rate (k2≈ 0.05-0.27 M-1s-1) (Ramirez et al., 2009). The oxidation rate during the second stage was thus slower than the first one.
The effect of H2O2 dose
The effect of initial hydrogen peroxide concentration on TOC conversion can be observed in Figure 4; the results show that the degradation rate increased for increasing hydrogen peroxide loads when this load changed from 20 to 60 mM; however, the reaction rate decreased when 70 mM H2O2 concentration was used. The fact that extremely high H2O2 concentration values led to a decrease in TOC conversion in some conditions was possibly due to the competition between these species for hydroxyl radicals. Indeed, •OH radicals are quite non-selective, reacting with the organic matter present but also with other species. In this work, a maximum wastewater degradation performance value was achieved with 60 mM hydrogen peroxide concentration due to the following reaction having a high kinetic constant that allowed degradation of H2O2 into water (k7≈ (1.2*107-3.3*107) M-1 s-1) (Ramirez et al., 2009):

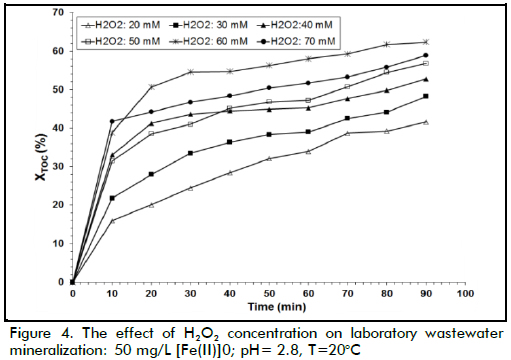
Economic optimization
Using Fenton's reagent as a wastewater treatment involved many conditions in accordance with technical requirements for decontamination and regarding treatment cost. An economic assessment of the Fenton process should take into account initial investment, operating costs and the expenses arising from inadequate regulatory completion. The Fenton process could be defined as a process involving a large demand for reagents which cannot be recovered due to their consumption and dispersion in reactive medium. This led to a need for determining operating conditions which would allow suitable TOC removal and an effective treatment cost. Such requirement is critical because the process could become expensive if reagent consumption rises and because cost depends on the scale of implementation.
Cost in terms of operational variables and some penalties related to lack of regulatory compliance was used to describe using Fenton's reagent as treatment for the chemical lab studied to establish accurate operating conditions. Cost F(x) was established as follows:
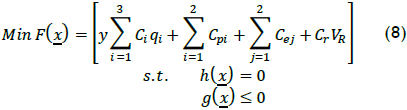
where y referred to matching reaction processed per day, Ci the individual cost per unit for each operational variable i, qi the quantity used for each operational variable i per batch, Cpi the cost of regulatory incompliance or saving due to reuse of water per volume of treated water, Cei service costs, Cr reactor cost per volume and VR reactor volume. Restriction h(x) described the system (i.e. mass and energy balance, XTOC, sizing, etc.), and g(x) established boundaries for the problem (i.e. Tr-max, XTOC-min, VRmax, etc.).
Taking into account that the cost function involved the four measured variables, and these determined TOC removal pattern as well as part of the operational costs of the treatment, it was necessary to make explicit how such variables affected this function. One way of doing this was by means of the second order polynomial model described above. The model described by equation (5) was used for fitting the data shown in Table 2 after codification in accordance with equation (4), where X1, X2, X3 and X4 referred to [Fe(II)], [H2O2]0, temperature and pH, respectively. Table 3 shows the determined coefficients after fitting by least-squares using GAMS software. Figure 5 shows how the model properly predicted XTOC in the measured interval, achieving 0.94 R2 which means the model was able to explain close to 94% of data variability.
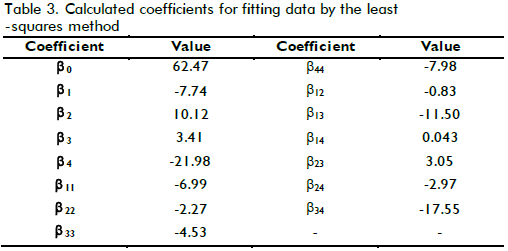
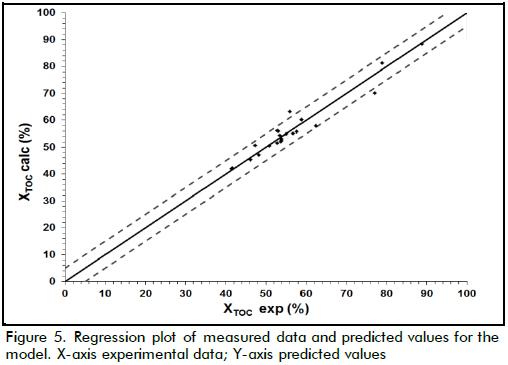
As can be seen from Table 3, XTOC had a strong dependence on pH, and hydrogen peroxide concentration due to the higher values of the linear terms in the model. Nevertheless, there was another strong effect produced by the interaction between T-pH and T-Fe concentration (interaction parameter βij ). That meant each variable played an important role during oxidation, but temperature maintained a remarkable influence on TOC removal. In fact, above 20% XTOC increase was achieved when temperature increased from 20oC to 60oC.
The problem optimisation described by equation (8) is mixed integer nonlinear programming (MINLP) which defined operational costs for the Fenton reaction in study. Using the parameters listed in Table 4, and the BARON solver (GAMS), problem (8) was solved to establish an operational condition where TOC removal was significant and the operational cost of treatment led to economic feasibility in accordance with laboratory budget.
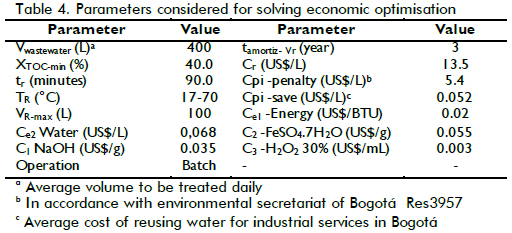
After solving the optimisation problem, the condition that produced the lowest cost was 36ppm [Fe(II)]0, 45.5mM [H2O2]0 TR = 20.4oC and pH=2.6, giving an expected XTOC= 50.6 %. The cost was US$ 20.19 representing total daily cost for treating 400L wastewater in 7 reaction batches, meaning US$ 0.050 per litre of treated wastewater. When the lowest cost condition was compared to the cost for the best performance, at 50ppm [Fe+2]0, 50mM [H2O2]0, TR = 80oC and pH= 2.8 with XTOC=88.8%, total treatment cost was US$ 24.90 daily, 23% higher than optimised conditions. An experiment was carried out in optimised conditions to verify the mathematical model's predictions. Table 1 lists the characterisation of the water treated in such operating conditions, giving XTOC=53.9% which was close enough to empirical model prediction. A higher level of detail could probably be obtained if TOC measurements were complemented by characterising each pollutant present in wastewater. The integration of such information could provide a kinetic model and thereby a more precise design for the reactor and the complete system.
Conclusions
The results confirmed the potential of Fenton's reagent for treating real wastewater and they also suggested that this method is a viable option for treating laboratories' wastewaters due to the TOC reduction achieved. The best performance was obtained using pH 2.8, 50 mg/L Fe(II) concentration and 50mM initial H2O2 concentration which led to 88.8% TOC removal. Even in the worst operational conditions, Fenton's reagent gave close to 41% TOC removal which meant the evaluated process could be applied to a wide range of operating conditions, including pH around 6. The economic optimisation of Fenton's reaction was interesting as assessment took additional parameters into account to determine operating conditions able to comply with technical, economic and regulatory requirements. Economic optimisation specified that expected TOC removal was 50.6 % at 36ppm [Fe(II)]0, 45.5mM [H2O2]0, TR=20.4oC and pH=2.6, giving a daily cost of US$ 20.19. The results could be applied to a similar process having similar characteristics, as the mathematical model was developed in accordance with specific restrictions and over a specific range for the variables.
Acknowledgements
The authors gratefully acknowledge the CAR's Environmental Lab in Bogotá for providing the wastewater samples from the treatment plant, as well as for making its laboratory available for developing this project. Technical assistance by the staff of the Universidad Nacional de Colombia's Chemical Engineering Laboratory in Bogotá is highly appreciated.
References
Badawy, M. I., Ali, M. E. M., Fenton's peroxidation and coagulation processes for the treatment of combined industrial and cosmetic wastewater., Journal of hazardous material, Vol. B136, 2006, pp. 961-966. [ Links ]
Bautista, P., Mohedano, A. F., Gilarranz, M. A., Casas, J. A., Rodriguez, J. J., Application of Fenton oxidation to cosmetic wastewaters treatment., Journal of hazardous material, Vol. 143, 2007, pp. 128-134. [ Links ]
Benatti, C. T., Granhen, C. R., Guedes, T. A., Optimization of Fenton´s oxidation of chemical laboratory wastewaters using response surface methodology., Journal of environment management, Vol. 80, 2006, pp. 66-74. [ Links ]
Bianco, B., De Michelis, I., Veglio, F., Fenton treatment of complex industrial wastewater: Optimization conditions by surface response method., Journal of hazardous material, Vol. 186, 2011, pp. 1733-1738. [ Links ]
Bigda, R. J., Consider Fenton´s chemistry for wastewater treatment. Chemical engineering progress, environmental protection., 1995, pp. 62-66. [ Links ]
Dutta, K., Mukhopadhyay, S., Bhattacharjee, S., Chaudhuri, B., Chemical oxidation of methylene blue using a Fenton-like reaction., Journal of hazardous material B, Vol. 84, 2001, pp. 57-71. [ Links ]
EPA, Method 200.8 Determination of trace elements in waters and wastes by inductively coupled plasma - mass spectrometry., Cincinnati, Ohio, U. S. Environmental Protection Agency, 1994. [ Links ]
Kavitha, V., Palanivelu, K., The role of ferrous ion in Fenton and photo-Fenton processes for the degradation of phenol., Chemosphere, Vol. 55, 2004, pp. 1235-1243. [ Links ]
Lee, H., Shoda, M., Removal of COD and color from livestock wastewater by the Fenton method., Journal of hazardous material, Vol. 153, 2008, pp. 1314-1319. [ Links ]
Lin, S. H., Lo, C. C., Fenton process for treatment of desizing wastewater., Water research, Vol. 31, No. 8, 1997, pp. 2050-2056. [ Links ]
Lucas, M. S., Peres, J. A., Removal of COD from olive mill wastewater by Fenton's reagent: Kinetic study., Journal of hazardous material, Vol. 168, 2009, pp. 1253-1259. [ Links ]
Malik, P. K., Saha, S. K., Oxidation of direct dyes with hydrogen peroxide using ferrous ion as catalyst., Separation and purification technology, Vol. 31, 2003, pp. 241-250. [ Links ]
Martínez, N. S. S., Fernández, J. F., Segura, X. F., Sánchez, A., Pre-oxidation of an extremely polluted industrial wastewater by the Fenton's reagent., Journal of hazardous material, Vol. B101, 2003, pp. 315-322. [ Links ]
Méndez, R. I., García, R. B., Castillo, E. R., Sauri, M. R., Tratamiento de lixiviados por oxidación Fenton., Revista Ingeniería e Investigación, Vol. 30, No. 1, 2010, pp. 80-85. [ Links ]
Metcalf, Eddy, Inc., Wastewater engineering: treatment and reuse., 4th ed., Boston, Mc Graw Hill, 2003, pp. 1819. [ Links ]
Neyens, E., Baeyens, J., A review of classic Fenton´s peroxidation as an advanced oxidation technique., Journal of hazardous material, Vol. B98, 2003, pp. 33-50. [ Links ]
Pignatello, J. J., Dark and photoassisted iron (3+)-catalyzed degradation of chlorophenoxy herbicides by hydrogen peroxide., Environmental Science & Technology, Vol. 26, 1992, pp. 944-951. [ Links ]
Pera-Titus, M., Garcia-Molina, V., Baños, M. A., Gimenez, J., Esplugas, S., Degradation of chlorophenols by means of advanced oxidation processes: a general review., Appl. Catal. B: Environ., Vol. 47, 2004, pp. 219-256. [ Links ]
Ramirez, J. H., Costa, C. A., Madeira, L. M., Experimental Design to optimize the degradation of the synthetic dye Orange II using Fenton´s Reagent., Catalysis Today, Vol. 107-108, 2005, pp. 68-76. [ Links ]
Ramirez, J. H., Duarte, F. M., Martins, F. G., Madeira, L. M., Modelling of the synthetic dye Orange II degradation using Fenton's reagent: From batch to continuous reactor operation., Chemical engineering journal, Vol. 148, 2009, pp. 394-404. [ Links ]
Rodrigues, C., Madeira, L. M., Boaventura, R., Optimization of the azo dye Procion Red H-EXL degradation by Fenton's reagent using experimental design., Journal of hazardous material, Vol. 164, 2009, pp. 987-994. [ Links ]
Standard methods. Standard Methods for the Examination of Water and Wastewater., 21th ed., Washington D. C., American Public Health Association/American Water Works Association/Water Environment Federation, 2005. [ Links ]
Swaminathan, K., Sandhya, S., Sophia, A. C., Pachhade, K., Subrahmanyam, Y. V., Decolorization and degradation of H-acid and others dyes using ferrous-hydrogen peroxide system., Chemosphere, Vol. 50, 2003, pp. 619-625. [ Links ]
Walling, C., Fenton´s reagent revisited., Accounts of chemical research, Vol. 8, 1975, pp. 125-131. [ Links ]