1. Introduction
The term clay was initially defined as a material made up of grains less than 2 μm in diameter. Clay minerals make up about 16% of the total volume of matter on the earth's surface [1]. As a rock, clay is generally made up of a mixture of clay minerals and other rocky debris of varying compositions.
With the conventional exploitation of clay materials by the industry, new geopolymerization processes have recently been developed to produce building materials in the form of geopolymers by using kaolinite. These products could be used as low-cost building materials for green housing. They are characterized by high strength, high heat resistance, low production cost, low energy consumption, and low CO2 emissions [2].
However, the phenomenon of shrinkage and swelling constitute the most important risks induced by clay soils. These soils have high plasticity indices that can damage the structures they are supporting, especially when a significant variation in their water content occurs. This phenomenon can cause damage in constructions if the foundations and structure are not rigid enough [3].
These damages can be cracking on the facade, distortions of doors and windows, dislocations of pavements and partitions, or ruptures of buried pipes.
The pathologies observed at the site of the Hacène Ben Mouloud university campus in Médéa, in Algeria, are indicative of this phenomenon [3]. Other sites in Algeria are a matter of significant concern because their type of soil can favor the occurrence of swelling and shrinkage phenomena. The nature of the soil is the predominant factor that predisposes a site to the shrinkage-swelling hazard.
Soil expansion is a major challenge for scientific research [4-8], being an intrinsic property caused by the presence of active clay minerals in soils [9,10]. Detecting the presence of such clay minerals is a key factor in differentiating potentially expansive soils. Additionally, identifying and quantifying their abundance is essential to assess soil expansion. In this sense, the conventional mineralogical analysis includes X-ray diffraction analysis, scanning electron microscopy, transmission electron microscopy, differential thermal analysis, thermogravimetric analysis, and various methods of chemical analysis. These methods are essential in research laboratories to explore the basic properties of clay minerals. However, they are expensive and require sophisticated laboratory procedures. The application of remote sensing techniques can constitute an alternative to supplying the information obtained from these methods.
The application of remote sensing sciences in the field of geology is very diverse and wide. One of its most important applications in earth sciences is geological mapping [11]. The use of satellite data in combination with data derived from field geology, geochemistry, geophysics, and laboratory analysis has provided valuable and detailed information useful for mineralogical and geological studies [12]. This technique has been used successfully in numerous studies, such as extracting information for environmental planning in cities, determining potential geothermal energy areas as well as mapping clay soils [13-17].
Images from the series of LANDSAT satellites have been a key element of geological remote sensing since 1973 and can be used to determine land use, geological and mineralogy exploration [18]. However, NASA’s on-orbit Advanced Spaceborne Thermal Emission and Reflection Radiometer (ASTER) multispectral sensor, collects data in more bands with comparatively narrower spectral channels than Landsat data. Furthermore, ASTER’s thermal bands are favorable for mapping silicates and carbonates [19]. These advantages make ASTER an appropriate satellite sensor for spectral mapping techniques among other multispectral sensors [18].
ASTER has three spectral bands in the visible near-infrared (VNIR), six bands in the shortwave infrared (SWIR), and five bands in the thermal infrared (TIR) regions, with 15-, 30-, and 90-m ground resolution, respectively. The VNIR subsystem has one backward-viewing band for stereoscopic observation in the along-track direction. Because the data will have wide spectral coverage and relatively high spatial resolution, it will be possible to discriminate a variety of surface materials [20].
ASTER image analysis needs to perform an atmospheric correction in order to obtain reflectance values [14].
The reflectance spectra of minerals are well known, and many studies have been developed to determine the reflection spectrum of rocks. The reflectance spectra of minerals are measured by spectroscopic instruments of varying resolution and stored in spectral libraries that are stressed in digital formats [11].
Clay is generally made up of smectite, kaolinite and, illite. Smectite is a group of clay minerals that is characterized by three layers, including two layers of silicon and oxygen tetrahedron and one layer of aluminum and hydroxyl octahedron, the most important member of those is montmorillonite. In these layers, water molecules are absorbed between the layers. Montmorillonite approaches a strong absorption spectrum of 1.4, 1.9, and 2.2 µm. The spectra of illite, montmorillonite, and muscovite have almost the same absorption bands. Similar to montmorillonite, illite shows an absorption band of 1.4 and 1.9, as well as 2.2, 2.3, and 2.4 µm [11,21].
Kaolinite is one of the clays that consists of two layers of silica in the form of tetrahedron and aluminum in the form of octahedron. Absorption properties of the whole kaolinite range are 2.2 µm and 1.4 µm. The reflection spectra of illite, kaolinite and montmorillonite are in the short infrared spectral range [11,22].
The mapping of clay soils can thus be carried out by spectral methods, allowing a rapid delineation of clay units and the identification of their mineralogy, in particular with regard to illite, montmorillonite and kaolinite [21]. The objective of this study is to present an efficient and inexpensive satellite method to characterize soil types, in particular clay soils, by using spectra ASTER images as the association of SWIR and VNIR in this work.
2. Mers El Kébir region
The Mers El Kébir city is located in northwest Algeria, bordered by the Mediterranean Sea to the north [Figure 1]. It is a municipality in the Wilaya of Oran, which is 7 km away and separated from Oran city by Mount Murdjado, which directly overlooks the sea [23]. In antiquity, Mers El Kébir was a port called Portus Divinis and an important maritime arsenal of the Almohad dynasty in the 12th century. Mers El Kébir currently hosts an important industrial shipbuilding complex.
Mers El Kébir is located in a depression between Mount Santon to the northwest and Mount Murdjadjo to the southeast. The geology of Mers El Kébir first concerns Mount Murdjadjo, which is composed of native schistosity [24]. Furthermore, Miocene clays in the Mers El Kébir region are sediments of marine origin that were deposited in a fairly thick layer (300 m) during the Tortonian period. The formation consists mainly of illite and montmorillonite, with an appreciable level of calcium carbonate [25].
The presence of a very clayey soil prompted the emergence of the brickyard industry in 1868 with the exploitation of a large deposit of clayey marls on the sides of the Santon hill. Figure 2 shows the geological composition of the Mers El Kebir region.
3. Methodology
Each element of the earth's surface can be identified by an analysis of its recorded spectral signature from visible to thermal infrared [22]. The spectral signature of each element (plants, rocks, soils, minerals) is characterized by absorption bands, which can be compared to the spectral signatures of different elements that discriminate elements like minerals [27].
Spectroscopy analyzes the reflectance of materials which reflects the interactions of light with matter in the form of absorption, scattering, or reflection.
The absorbed energy forms depressions on the absorption curves. The general shape of these curves and the position of the depressions thus form a spectral signature allowing the characterization of materials
The minerals can be discriminated by reflectance measurements in three main atmospheric windows: 1. visible and near infraRed (VNIR) from 0.4 to 1.1µm, 2. Short Wave InfraRed (SWIR) from 1.1 to 2.5 µm and 3. Thermal InfraRed (TIR) from 8 to 14 µm [27].
ASTER library, provided by NASA (National Aeronautics and Space Administration - USA) contains more than 2000 spectra of minerals, rocks, soils, man-made materials, water, and snow (http://speclib.jpl.nasa.gov) and makes part of the spectral libraries, expressed in reflectance, for certain natural and artificial materials that are available to the public. The USGS (United States Geological Survey) library contains about 500 spectra for minerals, mainly in addition to a few plants. These libraries have been used in many works on remote sensing minerals identifications through satellite images [28-31].
The NASA spectral library deconvolved into ASTER bands in the SWIR2 range highlights several spectral characteristics relevant to the classification of clay materials [Figure 3]. The characteristics highlighted are as follows. Illite exhibits an absorption characteristic in Band 6 and a slight flexion in Band 8; montmorillonite exhibits a unique absorption characteristic in band 8; kaolinite has a large absorption capacity in bands 5 and 6 and a slight inflection in band 8. These spectral characteristics are sufficiently discriminating to allow the identification of the mineral composition [28].
To achieve the classification of clay materials in the study area, the NASA spectral library was used. The SAM (Spectral Angle Mapper) mapping method, is widely validated in the scientific literature. The Spectral Angle Mapper (SAM) method [32,33], compares the reference spectra of the spectral library with each spectrum of pixels in the image. The SAM method algorithm determines the similarity between a reference spectrum and an image spectrum by calculating the “spectral angle” between them. An angular threshold value is then chosen, so that only pixels having smaller angles to the reference spectra will be mapped.
Smaller values for the angle indicate the highest similarity between the pixel spectrum and the reference spectra.
4. Results and discussion
A false-color IR composite image (342 RGB) was generated in order to distinguish the different geomorphological units in the study area as shown in Figure 4.
The same ASTER image is used to identify clayey materials in the Mers El Kébir region. ASTER images allow the mapping of clay minerals, via the six spectral bands in its shortwave infrared range, in particular wavelengths between 2.145 and 2.43 μm [28]. This image is classified by the SAM method [Figure 5].
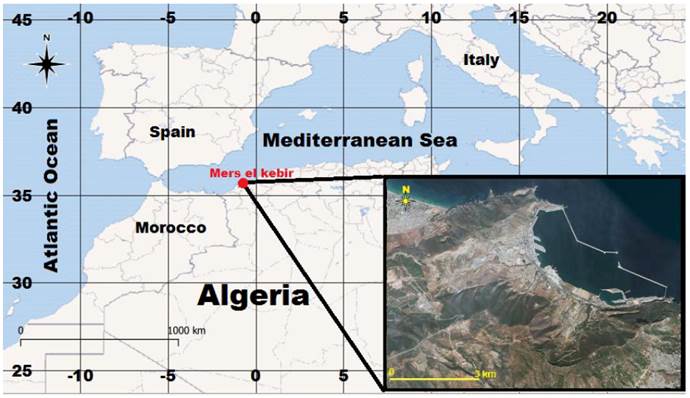
Source: authors
Figure 1 Geographical position of the city of Mers El Kébir in Algeria - WGS 84 Web Mercator.
Mers El Kébir clay is an aluminosilicate with a silica / alumina ratio of around 3.5. The percentages of Fe2O3 and CaO confirm the presence of goethite and calcite. In addition, the K2O content shows the existence of illite in the soil. The proportion of silica reflects the presence of silica in the form of associated minerals [34].
X-ray diffraction (XRD) analysis is used to determine the proportions of the different minerals present in a clay matrix [35].
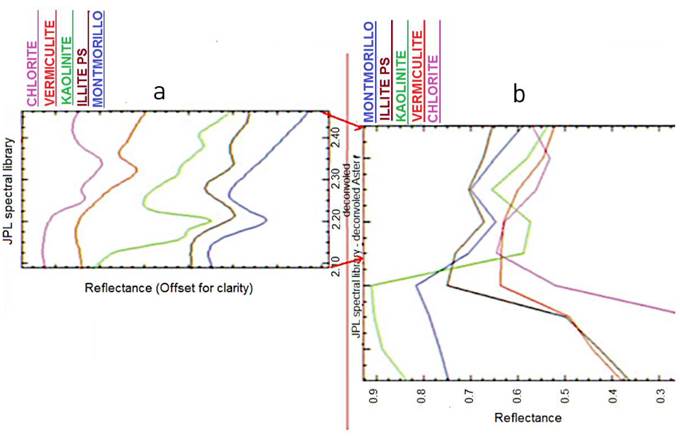
Source: [28]
Figure 3 a) various clay spectra in the SWIR2 range (wavelength on the abscissa); b) the corresponding ASTER-deconvolved spectra (band number shown on the abscissa).
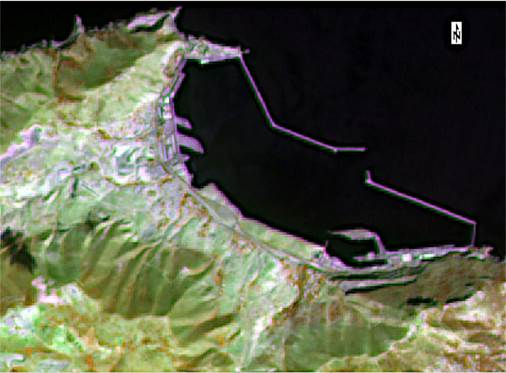
Source: Authors
Figure 4 False-color IR ASTER image dated 12/07/2001 of Mers El Kébir area, showing the main geomorphological features of the study region.
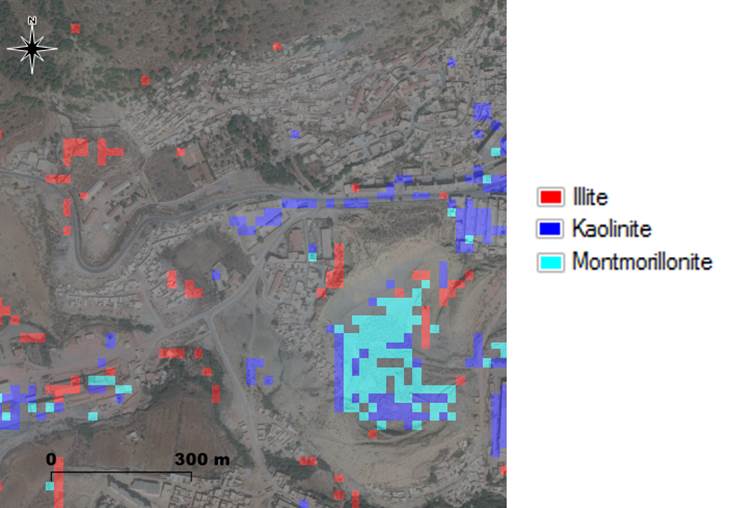
Source: Authors
Figure 5 Classification by the SAM (Spectral Angle Mapper) method of clayey materials from the quarry zone adjacent to the Mers El Kébir brickyard from an ASTER image dated 12/07/2001 adjusted on a Bing image.
This method is based on basal reflections giving d-spacing of the basal layer, which represents the thickness of the silicate layers. Clay mineral peaks can generally be distinguished by the width halfway up the peak.
Figure 6 shows the X-ray diffractogram which indicates that the clay of Mers El Kébir contains montmorillonite, illite, kaolinite, quartz and calcite.
The first peak corresponds to a basal spacing d = 13.8 × 10−4 μm, proving the existence of montmorillonite.
The presence of illite is demonstrated by the second peak corresponding to d = 10 × 10−4 μm. The third reflection at 7.1 × 10−4 μm is attributed to the presence of kaolinite. Reflections at 4.21 × 10−4 and 3.03 × 10−4 μm are attributed to quartz and calcite [34].
Figure 5 shows the presence of Kaolinite, Illite, and Montmorillonite, particularly in the quarry adjoining the Mers El Kébir brickyard. The proportion calculated using the designed GIS represents 20% of all classified materials. This same proportion is 23.8%, according to the analyzes carried out by XRD during the work of Bourokba Mrabent et al., 2017 [34]. This encouraging result shows that the mapping of clay soils can be carried out, at a lower cost, by spectral methods from ASTER images which are still used for the remote sensing of clay materials [36-38]. Thus, the phenomenon of shrinkage-swelling of the soil experienced by many regions [39], can be better controlled by the rapid delimitation of clay units and the identification of their mineralogy, in particular with regard to illite, kaolinite, and montmorillonite.
5. Conclusions
The use of ASTER data for the detection and mapping of heat-shrinkable clays has been demonstrated in the region of Mers El Kébir with an association of both spectral and texture characteristics from the VNIR and SWIR bands. This combination achieves higher accuracy than using spectral images alone [28]. The ASTER images allow an efficient approach to regional clay mineral mapping, via the six spectral bands in its shortwave infrared domain.
The application of this simple operational treatment method should improve the rapid mapping of clay zones. However, this approach can be improved by constructing a spectral library corresponding to samples of clay materials validated by X-ray diffractometric analyzes. Furthermore, the acquisition and processing of hyperspectral satellite images can provide better precision to the cartographies dedicated to the shrinkage-swelling hazard.