1. Introduction
Artisanal gold mining is an ancient practice in Colombia, predating Spanish colonization. Gold, an important element of indigenous culture and economy [1], was cast to form religious icons, jewelry, and offerings [2]. When the Spaniards arrived, mineral extraction increased, which contributed to the foundation of cities in western Colombia, such as Cali, Popayán, Cartago, and Santa Fe de Antioquia [3]. Between 1670-1800, gold production began in Santa Rosa de Osos and Rionegro in the department of Chocó [4]. In 1864, the foreign company Frontino Gold began exploiting hard rock deposits in Remedios and Segovia in the department of Antioquia [1] using dynamite and hydraulic mills. This led to the use of other mining techniques in Antioquia, such as cyanidation, precision surveying, and steam engines [4]. In these areas, gold miners began to use mercury (Hg) for gold extraction, a technique developed more than 450 years ago. Between 1550-1880, an estimated 200,000 tons of Hg were released in South America from gold mining [3].
In present-day Colombia, miners extract gold from alluvial and hard rock deposits [5] mainly located along the central and western mountain ranges [6]. These deposits are primarily exploited by miners from the artisanal and small-scale gold mining (ASGM) sector rather than conventional large-scale mining companies [7]. In Colombia, there are approximately 200,000 artisanal and small-scale gold miners, who annually produce 30 tons of gold, 10-20 tons of which is from Antioquia [8]. With a 5% growth in global production, gold mining has experienced a boom in the last decade [9], due primarily to increased international gold prices and investment by international companies [10]. Several departments in Colombia currently have both substantial foreign investment in mining along with high mining exports [11]. These departments include Antioquia, Bolívar, Caldas, Cauca, Chocó, and Córdoba, which are the main gold-producing departments in the country according to a report published by Colombia’s Mining and Energy Planning Unit [12].
Researchers estimate that there are more than 200,000 ASM sites in Colombia [8]. In 2018, a study associated 15 tons of Hg emitted into water bodies with 6,330 illegal gold mining operations, concluding that the operations impacted a total of about 200,000 hectares of river and jungle ecosystems [13]. Air Hg emissions reached 47 tons [14], and concentrations in mining towns in Antioquia are among the highest recorded in the world, reaching 1,000 µg/m3 [15]. These high levels have attracted global concern. In 2019, approximately 98,028 hectares of land in Colombia were affected by alluvial gold mining [16]. In that year alone, the ASGM sector emitted about 15 tons of Hg into the soil, according to the National Mercury Inventory.
According to the World Health Organization (WHO), exposure to high concentrations of Hg can impact the nervous, immune, and digestive systems, the lungs, and the kidneys [17]. The indiscriminate use of Hg is a critical problem in Colombia [18], with over 192 tons of Hg emitted into the environment annually according to a 2014 report published by CORANTIOQUIA [19]. As a result, Colombia was classified in 2012 by the United Nations Organization for Industrial Development (UNIDO) as the third most polluted country in the world [7], despite Colombia only being the nineteenth-highest gold producer in the world as of 2020 [20].
In 2007, the Colombian government began to develop and implement policies and legislation to control Hg use in ASGM and mitigate its associated impacts on human health and the environment. Following initial negotiations of the Minamata Convention [21], Colombia issued Law 1658 in 2013 to gradually eliminate Hg use [22], establishing the prohibition of Hg in mining activities by July 2018 and in other industrial activities by 2023 with provisions for commercialization [23]. Despite these efforts, illegal mining activities and Hg use continue to affect new areas. The national government views this as a priority issue. As a result, developing site assessment tools to investigate the impacts of Hg use in ASGM is crucial to prioritizing interventions.
In 2009, the international environmental health non-profit organization Pure Earth (formerly Blacksmith Institute) developed the Toxic Site Investigation Program (TSIP) to identify and assess contaminated sites in low- and middle-income countries and help communities and governments investigate and mitigate threats to human health from toxic pollution [24]. To date, the TSIP database contains roughly 5,000 contaminated sites [25], improving decision-making and intervention priorities in approximately 50 countries [26]. The TSIP methodology, a systematic process that is standardized worldwide, is a useful tool to diagnose the effects of Hg in high proportions, generating the possibility of prioritizing interventions in communities and/or ecosystems.
In 2016, Pure Earth began implementing the TSIP in Colombia with the support of the United States Agency for International Development (USAID) and in collaboration with the Colombian Ministry of Environment and Sustainable Development and regional environmental authorities. Information from the site assessments was collected and uploaded to the TSIP database (www.contaminatedsites.org) and made available to relevant stakeholders. During 2020-2021, the number of mercury-contaminated sites assessed through the TSIP expanded due to collaboration from the Ministry of Mining (MINEM) and additional support from the United Nations Development Programme (UNDP) [27].
This paper presents a rapid review of mercury-contaminated sites in various departments of Colombia using information from the TSIP database on demographic parameters and the degree of mercury contamination in soil and water. The sites were assessed for health risks to the surrounding populations in this study through the calculation of the Blacksmith Index. In addition to data from secondary studies, information on mercury-contaminated sites in Colombia available in the TSIP database is derived primarily from assessments conducted from November 2016 to February 2021 by Pure Earth investigators collecting data.
2. Methodology
The TSIP utilizes a rapid assessment protocol known as the Initial Site Screening (ISS), which is completed by professionally trained investigators and is designed to gather information related to environmental impacts and human health risks. Investigators collect qualitative and quantitative data, including an analysis of soil and/or water samples. ISS implementation uses the following steps: 1) review available information, evaluating parameters such as location, local contact, contaminant to be evaluated, availability of equipment, and work team; 2) visit site, performing activities such as interviews, simple and/or compound sampling, mapping and data collection with field equipment such as the X-ray fluorescence (XRF), Jerome, and Hermes (depending on equipment availability); 3) ensure information is collected in an organized and complete manner to comply with the TSIP database required fields.
The ISS is not a comprehensive assessment tool designed to fully characterize health risks, but rather is intended to prioritize sites for further analysis, regulatory inspections, and/or risk mitigation measures [28]. Because the ISS is a rapid assessment designed to be completed typically in one or two days at a low cost, only limited sampling is done with a focus on potential human exposure routes.
The ISS protocol follows a Pollution-Migration-Pathway-People model as the basis for understanding and assessing risks at a particular site. In short, this means that investigators record information on the following: 1) the source, nature, and concentration of a contaminant; 2) the migration route and media between the source and the most likely receptor community; 3) the most likely pathway(s) from the migration media into the human body; and 4) the behavior, location, density, and demographic details of the receptor community. Data from completed ISS are entered into the TSIP database, which is shared with relevant government agencies and is accessible to the public. Access to the TSIP database is managed by Pure Earth.
TSIP site assessment methodology includes a field screening for heavy metals using portable equipment such as an XRF device for measuring Hg (and other heavy metals) in soil. The use of the XRF depends upon equipment availability and field conditions. Depending on availability and the type of study being performed, other portable equipment can be used such as the Hermes or Jerome. For mercury-contaminated sites, in addition to the XRF, specific field instruments such as the Jerome or Lumex mercury analyzers are used to measure Hg vapors. Soil and water samples are also generally collected for laboratory analysis.
Along with environmental sampling, relevant information, including a description of the area and an estimate of the population potentially exposed to mercury, is collected from the site. To estimate the potentially exposed population, researchers include factors such as the number of adults and children living near the potential Hg source and the distance (50, 100, and 500 meters) of living quarters from the source. This information is generally collected by observation, available information, and official reports. If there are schools present, the number of students that attend each school is included in the estimate. If a nearby body of water is suspected to be the source of contamination, the number of people using this water source is also included.
The TSIP protocol includes the calculation of the Blacksmith Index (BI) which uses the collected data to quantify and rank the health risk of a contaminated site based on the potential population at risk, severity of contamination, and intensity of exposure. The BI is intended to help decision makers understand and mitigate public health risks posed by toxic sites [29]. The following information is required to calculate the BI: population demographics, contaminant concentration at site (ppm), the screening threshold value for the contaminant, and the Persistence Factor (PF). Using an algorithmic formula, a value between 0-10 is generated, with 10 signifying the highest risk and 0 the lowest. The BI formula is shown below in Equation (1):
Sector population refers to the population estimate; sector test result refers to the pollutant concentration value as read by the XRF or other sampling device; screening threshold refers to the Environmental Protection Agency (EPA) threshold at which contamination risk from the pollutant is considered acceptably low; the PF or Persistence Factor refers to the population's distance from and time spent in the contaminated site using a Spatial Attenuation Factor (SAF) provided by the EPA.
The algorithm takes the following into account:
Health risks from ASGM operations primarily related to Hg vapor inhalation, which crosses the lung/blood barrier, are included. Secondary routes include ingestion of contaminated water, fish, poultry, or eggs.
The overall risk is a factor of both population exposed and the concentration of Hg inhaled or ingested.
A logarithmic scale is appropriate because contamination concentrations tend to increase exponentially rather than arithmetically at contaminated sites.
Greater weight is given to young children and pregnant women. Smaller body weight and greater activity can lead to higher exposure levels in children for the same air concentration, and Hg exposure to pregnant women and young children can lead to impaired neurological development of the fetus or child because their brains are in active development.
Greater weight is given to indoor air contamination because Hg vapors are more likely to linger and not attenuate rapidly after cessation of generating operation. Hg is more likely to condense and settle in indoor spaces, leading to longer exposure times as indoor spaces heat up during the day. People are also more likely to spend time in indoor locations compared to outdoor processing areas, particularly if operations or storage is done in homes or shops where people spend significant time.
The soil and water data obtained from the investigated sites are compared to available standards for those media, which is an integral variable when calculating the BI. Because Colombia does not have a legal reference limit for Hg in soil, the EPA reference values of 11 parts per million (ppm) for soil in residential and agricultural settings and 46 ppm for industrial settings are used [30]. For Hg in water, a reference value of 0.01 ppm, which is the legal limit established in Colombia through Resolution 631 of 2015, is used [31].
Information on a total of 72 mercury-contaminated sites in Colombia was included in the TSIP database during the study period from 2016-2021. Secondary information from official reports and scientific articles that included measurements of Hg in hair, blood, and fish was used for 18 sites, or 25% of the total. As secondary information can be less reliable and may be outdated, these sites were excluded from the analysis. For the remaining 75% (54 sites), primary site investigation information from the TSIP database was used. Of these sites, Hg contamination was not detected at 10 sites, which were therefore excluded from the analysis. After applying the appropriate exclusion criteria, 44 sites derived from primary investigations with documented mercury contamination were included.
Because Pure Earth often employs the XRF device, which cannot be used for water measurements, the database has a higher number of soil measurements. Additional water analyzes are carried out associated with additional objectives to rapid measurements.
3. Results
Table 1 shows the distribution of the 44 sites identified as being contaminated with Hg in this study, including a summary of selected primary information from the TSIP database.
The highest levels of Hg contamination (higher than 300 ppm) were detected in the departments of Antioquia and Cauca. Sites in Nariño and Bolívar also showed significant mercury concentrations (higher than 200 ppm). These findings are consistent with the high presence of ASGM, mostly rock mining, in these departments. On the other hand, sites in departments characterized by alluvial mining, such as Chocó, exhibited the lowest mercury concentrations.
The data reported on the concentration of Hg in Antioquia are predisposed to the risk that is contaminating the population.
Concentration studies in runoff from gold mining show concentrations of approximately 211 ppm [32], which they compared with values recorded in the study (467 ppm) in areas such as Antioquia with a nearby population, evidence of the criticality of the high concentration of mercury in this area of Colombia and the need for intervention plans.
Based on information from the TSIP database, the department with the highest population at risk is Antioquia, with 30,479 people distributed among 16 identified sites. Of the sites, 25% are located on industrial land, 18.75% on agricultural land, and 56.25% on residential land. The department with the second highest population at risk is Bolívar, with 15,592 people distributed among 6 identified sites, all on residential land. The study includes a total of 55,095 potentially impacted inhabitants in 7 departments.
Of the 44 sites, La Bocana, Buenaventura in the department of Valle del Cauca exhibited the highest BI value at 7.31. This value suggests that Hg contamination presents a greater risk to the population in this site compared to other sites included in the study. However, it is important to note that the BI is a rapid initial assessment rather than a detailed risk assessment. To strengthen criteria for decision-making and intervention strategies, a detailed assessment should be applied to sites with a BI value greater than 5.
Figure 1 shows a map of several contaminated sites identified through the TSIP protocol and their relative Hg concentration level.
Table 1 Findings of mercury-contaminated sites identified in Colombia
Source: www.contaminatedsites.org
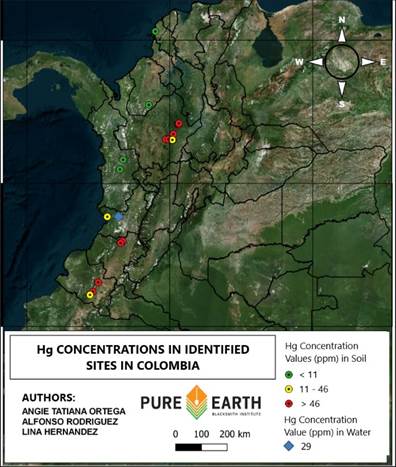
Source: www.contaminatedsites.org
Figure 1 Map of mercury concentrations at identified sites in Colombia
The overrepresentation of samples from Antioquia is due to the interest of local and national government entities as well as international organizations in diagnosing and intervening in areas of high Hg contamination.
Note that Antioquia has the most ASGM activity of all Colombian departments.
Table 2 shows the 10 most contaminated sites according to the BI calculated using data from the TSIP database. Of the 5 sites with the highest BI value, three are located where land use is classified as soil-residential, while the land use of the remaining two are classified as soil-agricultural and water-fishing. There is evidence that water bodies may display a higher BI value, as is the case of La Bocana, Buenaventura, which has the highest BI value of any site. This is because water appears to represent a higher risk than other sources of contamination, which increases the factor associated with the screening threshold in the BI formula. The sampled areas are mostly rural and tend to exhibit proximity to bodies of water.
La Cruzada is the site with the highest concentration of Hg in the soil at 467 ppm, with a BI value of 6.49. The estimated exposed population is 7,203 inhabitants, and 36.3% of the total population is located in the municipality of Remedios in the department of Antioquia. It should be noted that there are mines in the Remedios area that have been in continuous operation for more than 150 years and have produced more than 5 million ounces of gold [32].
Other sites with high Hg concentrations in soil include a site located in the municipality of Buenos Aires in the department of Cauca with a concentration of 340 ppm and a BI value of 4.62 and an area surrounding the Gualconda mine in Nariño with a concentration of 223 ppm in soil and a BI value of 5.36.
High Hg concentrations in both water and soil negatively impact the quality of life and health of nearby populations. Intervention in contaminated sites is defined by local governments or international agencies interested in carrying out mitigation or contaminant elimination actions. This study could serve as a decision instrument for government entities and other organizations to design interventions in accordance with the reported contamination.
The BI is a tool to prioritize sites for intervention and could serve as an input to aid the formation of intervention plans in Colombia and other countries. Other factors to create site contamination intervention plans include public policies, budgets, incidence, and impacts on ecosystems and people.
Table 2 The 10 most contaminated sites in Colombia according to BI calculation
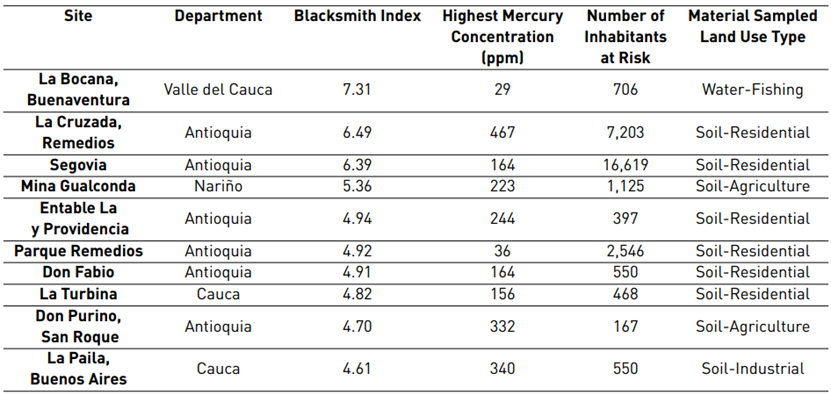
Source: www.contaminatedsites.org.
4. Conclusions and recommendations
Though many potentially contaminated former and current ASGM sites exist in Colombia, only 72 sites are registered in the TSIP database. Therefore, one recommendation is for central and regional authorities to expand the process of identifying and assessing mercury-contaminated sites in the country in order to create a more robust database. This will increase the accuracy of scoring high-priority sites and ensure sufficient data for intervention development, including analyses and costs. This document provides an analysis of gold mining sites in seven Colombian departments where Hg has been detected in soil and water, potentially impacting at least 55,095 people. These seven departments (Antioquia, Valle del Cauca, Cauca, Nariño, Bolívar, Córdoba, and Chocó) include regions with ASGM exploitation, which is known to be associated with Hg use.
Despite the government's efforts to move towards the elimination of Hg use, high Hg concentrations persist in residential and agricultural areas, negatively impacting local ecosystems and populations. Additional funds should be included in the national budget for the assessment of contaminated areas, interventions to remediate contaminated areas, and projects for the transition to mercury-free mining methods.
Of the 44 identified sites, La Bocana, Buenaventura in the department of Valle del Cauca is the site which, with a BI of 7.31, exhibited the greatest risk for inhabitants, requiring urgent intervention compared to other identified sites. Compared to La Bocana, the site of La Cruzada, Remedios in Antioquia presented higher Hg concentrations at 467 ppm but a lower BI of 6.49 due to a different Hg migration route and related impacts.
Contaminated sites were identified mainly in Valle del Cauca, Nariño, Chocó, Antioquia, and Cauca, which are among the departments in Colombia with the highest gold production as of 2020. However, site assessment should be expanded further in other departments such as Bolívar and Caldas where gold mining occurs but little measurement information exists. When planning future interventions for a contaminated area, additional analysis variables should be included, such as accessibility, the extension of affected land, demographic distribution of the population, public safety, support from regional entities and the community, and disaggregation of impact by age.
One advantage of the rapid assessment methodology is that it is intended to prioritize sites for further investigation based on semi-quantitative estimates of public health risk, being valid for prioritizing interventions and making decision tools for government agencies.
Future studies should include more Hg concentration measurements in water samples, including measuring concentrations in sediments, fish, and air near the water body, to expand the risk assessment calculation for people potentially exposed towater source.