Introduction
Yamu (Brycon amazonicus) is a fish species that has the potential to be farmed because of the meat quality and omnivorous habits that promote food efficiency (Useche et al, 1993). However, the fish farming and supply of yamu meat is still developing although there has been significant progress for issues of reproduction, feeding and cannibal ism control at the nursery stage (Arias, 2006), but there are only a few studies on the technological properties and processes that affect yamu meat quality.
It is known that texture is important in determining the quality of fish meat (Ando et al, 1999; Suarez-Mahecha et al, 2007) because losses in this property suggest changes in technological, sensory and microbiological properties. Studies have shown that fish meat loses texture after 24 h of storage on ice (Oka et al, 1990; Toyohara and Shimizu, 1988). The softening of fish meat has been studied in ma rine fish (Ladrat et al, 2003; Morrison et al, 2000; Wang et al., 2009) and freshwater fish species (Ando et al, 1999; Ma and Yamanaka, 1991; Suarez-Mahecha et al., 2007; Wang et al., 2011); however, these studies have focused on highly commercial species (Suárez-Mahecha et al., 2007). When stored at low temperatures, yamu meat has rapid softening. Currently, it is not known with certainty which factors cause this or through which mechanisms (Ladrat et al., 2003). Authors have suggested that texture losses in fish meat in cold storage is caused by the effect of proteases (Verrez-Bagnis et al., 1999), especially cathepsins, calpains and hydrolytic enzymes such as elastase and collagenase (Chéret et al., 2007).
It has also been reported that, after storing hake fillets and whole hakes at -8°C for 12 months, there was a substan tial increase in the pH (Simeonidou et al., 1997). Another functional property that is affected when fish meat is in cold storage (<0°C) is the WRC because it depends on the moisture and stability of the muscular structure (Sánchez-Valencia et al., 2014). Altering this property has negative effects on the marketing of meat because weight loss during storage and during cooking results in immediate rejection by consumers.
The aim of this study was to evaluate the effect of cold storage on yamu meat (Brycon amazonicus), depending on temperature, shelf life and origin of fish, on the protein profile, microstructure and technological properties such as the WRC, pH and texture.
Materials and methods
Biological material
The yamu (Brycon amazonicus) fishes were obtained in the Department of Meta. One group was obtained from an aquaculture farm located in the department of Meta (4°16'17.1"N and 73°29>39.0» W, municipality of Cumaral) (farmed); the second group was caught in a natural envi ronment (4°32'13.9"N and 71°50'47.2"W), municipality of Puerto Gaitan, San Miguel district (fished) (Meta river). The fish (6 from the river and 7 from the farm) were ki lled with a blow to the head and immediately sent to the laboratory in polystyrene coolers with ice packed in plastic bags, avoiding direct contact between the ice and fish. In the laboratory for meat and derivatives processes, the fish were washed with cold water (5±1.5°C), eviscerated and filleted; randomly, fish fillets from each source were assigned to 3 groups of 8 fillets each for subsequent analy sis. The first group had fresh samples that were analyzed immediately after filleting. The second group had samples stored at 0±1.5°C. The third group had samples stored at -8±1°C. All of the samples were analyzed during storage for 13 and 61 h.
Optical microscopy
In order to analyze changes in the muscle structure and microstructure of the yamu meat in cold storage, a histolo-gical analysis was carried out. The samples were taken at the epaxial level (back) of the fillets and then cut into 2 x 2 x 2 mm pieces, observing the muscle fibers transversely. The pieces were placed in 1.5 mL vials and fixed in 3% buffered formalin for 2 d. They were then dehydrated in a graded series of alcohols (50, 60, 70, 80, 90, and 99%), followed by xylene. The samples used in the optical microscopy were embedded in paraffin and cut into microtome (4|im) and stained with Masson trichrome (MT). For this process, the samples were washed with distilled water and immersed in Weigert hematoxylin for 5 min, then washed with tap water at 5°C and immersed in ponceau fuchsin for 5 min and immersed in phosphomolybdic acid for 5 min. This solution was changed to an Aniline Blue solution for 5-7 min. Finally, the tissues were analyzed under a microscope (Leica DM750 P, Leica Microsystems, Heerbrugg, Switzer land) and images were taken at 40X in order to highlight the collagen fibers.
Scanning electron microscopy (SEM)
The samples for this procedure were fixed in 3% glutaral-dehyde buffer for 72 h, then dried at the critical point using an EK 3150 for 15 min and covered with gold film using a Quorum, Q150R ES. To proceed with the SEM (FEI, Quanta 200-r), the samples were mounted on individual slides. The images were captured at 4000X.
Water retention capacity (WRC) of the yamu meat samples
The WRC of the yamu meat samples was estimated fo llowing the methodology proposed by Sánchez-Alonso (2012), for which 3 g of meat were taken and wrapped in two filter papers (Whatman No. 1, 110 mm diameter) and placed in a falcon tube. Subsequently, they were centrifuged for 15 min at 3,000 g. Then, the paper was removed and the samples were weighed. The WRC was expressed as a per centage of water retained by the sample after centrifugation.
Analysis of the yamu meat texture
The texture was evaluated using a Stable Micro System texture analyzer (TA.XT2, Surrey, UK), with a flat end cylinder (10 mm in diameter, P/10 type) that was pressed against the muscle tissue. All of the tests were done at 5°C, keeping the fillets in Petri dishes on ice. Three cubes (2 x 2 x 1.2 cm) were taken from each fillet, from the top of the sideline. The response variable was the maximum cutting force (g g-1 sample). A compression test was done before the test: 1.00 mm s-1, test speed: 1.10 mm s-1, speed after test: 10.00 mm s-1, distance between the cylinder and sample: 15.0 mm, compression of the sample: 40.0%. The conditions were the same for each sample and seven measurements were taken for each meat portion (Larsson et al., 2014).
pH determination for the yamu meat samples
This parameter was analyzed following the methodology described by Mohan et al. (2007), in which a homogenized sample was held with cold deionized water (0±0.5°C) 1:6, using a homogenizer (Ultra-Turrax IKA T-25, Campinas, Brazil) at 10,000 rpm for 1 min. Once the homogenate was obtained, the reading was taken with a pH meter (17±1.5°C) (JENWAY®, JW-3505, Staffordshire, UK). Five measure ments were taken for each homogenate.
Extractions of yamu muscle tissue proteins
The protein extraction was done by modifying the method proposed by Cao et al. (2006). A one gram sample was homogenized in a homogenizer (Ultra-turrax IKA T-25, Campinas, Brazil) with 4 volumes of distilled-deionized water for 30 seconds, then centrifuged at 5,000 g and 4°C for 5 min; the supernatant was removed and the precipitate was washed with a cold high ionic strength solution (50 mM phosphate buffer, pH 7.5) for 30 s, then the homogenate was centrifuged at 5,000 g and 4°C for 15 min; the precipitate was suspended in 4 volumes of cold 50 mM phosphate buffer, cold pH 7.5 and repeated the centrifugation process two more times. The supernatant was discarded, a final centrifugation was performed at 3,000 g for 15 min and the precipitate was diluted with 50 mM phosphate buffer and 500 mM NaCl pH 8. The protein extracts were stored at -40 ± 0.5°C.
Protein quantification
The protein was quantified as suggested by Torres et al. (2012), for which 600 mL of each protein extract were used and the required volume of 1% NaCl was added to complete 1 mL; then 1 mL of Bradford reagent was added (Sigma-Aldrich, Saint Louis, MO) to wait for color deve lopment for ten minutes and the absorbance was read at 450/590 nm.
SDS-PAGE Electrophoresis (polyacrylamide gel electrophoresis with dodecyl sulfate sodium)
The electrophoresis was done under denaturing conditions (SDS-PAGE) (Mini Vertical Electrophoresis Tetra-PRO-TEAN® Cell, Bio-Rad, Hercules, CA). The separating gel was 10% and the gel concentration was 5% (w/v). A standard molecular weight of 20 to 210 kDa was used and the stai ning was done with G-250 colloidal coomassie blue (CBB).
Statistical analysis
The data (pH, texture and WRC) were subjected to an ANOVA to establish whether or not there were differences between the levels of each of the factors. For differences in the levels of the factors, a Tukey test was used. In order to find the effect of the interactions between the factors, an analysis of multifactorial variance was conducted, with the response variables texture (Force cutting), pH and WRC; meanwhile the controlled variables were time and tempe rature of storage. SPSS 19.0 ® (2010) was used.
Results
Scanning electron microscopy (SEM)
The scanning electron microscopy analysis of the yamu meat for the effect of cold storage of farmed and fished fillets can be seen in figure 1.
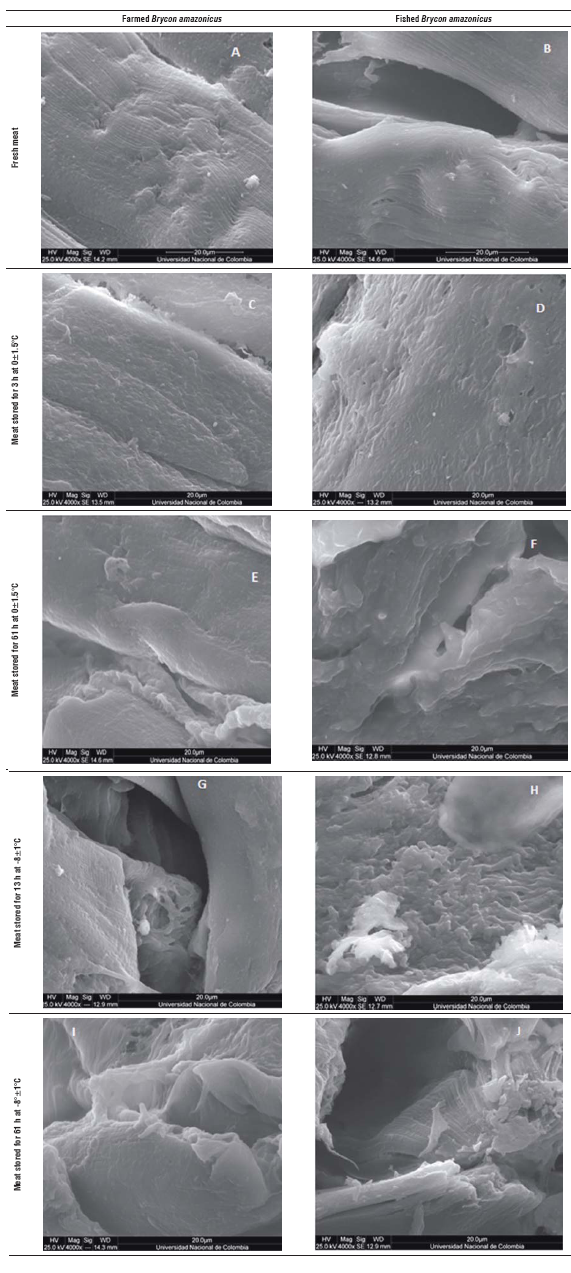
FIGURE 1 Scanning electron microscopy images of Brycon amazonicus meat with 4,000 X magnification. A. Fresh farmed meat; B. Fresh fished meat; C. Farmed meat stored for 13 h at 0±1.5°C; D. Fished meat stored for 13 h at 0±1.5°C; E. Farmed meat stored for 61 h at 0±1.5°C; F. Fished meat stored for 61 h at 0±1.5°C; G. Farmed meat stored for 13 h at -8±1°C; H. Fished meat stored for 13 h at -8±1°C; I. Farmed meat stored for 61 h at -8±1°C; J. Fished meat stored for 61 h at -8±1°C.
At the microstructural level, the yamu muscle fiber samples that were analyzed when the fish was fresh dem onstrated myosepta, myocommata and integral muscle fibers (Fig. 1A and B). Meanwhile, in the samples main tained at 0°C for 13 h (Fig. 3C and D), there were changes in the organization of the muscle fibers, the structure had architectural loss and there was a bond loss between the myotomes. The samples stored at this temperature for 61 h (Fig. 1E and F) showed a greater loss of muscle architecture and collagen degradation. The separation effect between the muscle fibers and general myofibrillar structure presented amorphous forms.
The samples stored at -8±1°C (Fig. 1 G, H, I and J) had damage caused by the cold in the muscle structure that was higher; this tissue had pronounced invaginations and the myofibrils lost the original structure because of the effect of the interfiber collagen degradation. This phenomenon is accentuated with increasing the storage period under cold conditions; the samples stored for 61 h had greater detachment between the myosepta and full deformity of the primary structure of the muscle. In addition to this technique, it was possible to see slight differences between the muscular structures of the farmed fish and fish from the river, such as myofibrils and connective tissue. The tissue from the river fish had pronounced fissures starting at 61 hs when stored at 0±1.5°C (Fig. 1D).
Optical microscopy
Figure 2 shows the images of the optical microscopy of the yamu meat (Brycon amazonicus) for the effect of cold storage of the fish fillets, from the farm and river.
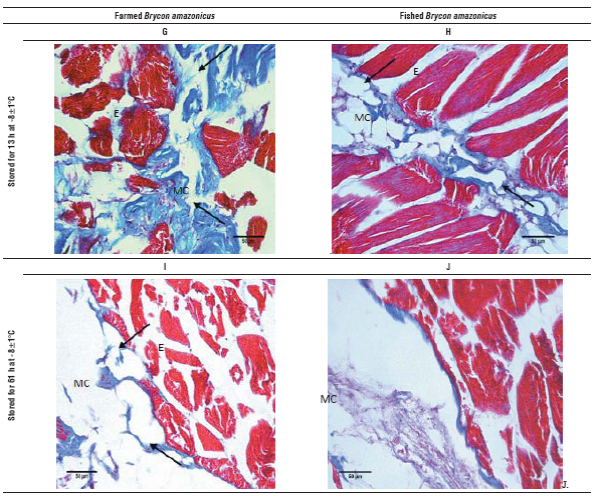
FIGURE 2 Optical microscopy images of yamu Brycon amazonicus meat stained with Mason trichrome, 40X magnification. A. Fresh meat from farming; B) Fresh meat from river; C) Farmed meat stored for 13 h at 0±1.5°C; D. Meat from the river stored for 13 h at 0±1.5°C; E. Farmed meat stored for 61 h at 0±1.5°C; F. Meat from the river stored for 61 h at 0±1.5°C; G. Farmed meat stored for 13 h at -8±1°C; H. Meat from the river stored for 13 h at -8±1°C; I. Farmed meat stored for 61 h at -8±1°C; J. Meat from the river stored for 61 h at -8°C. Ruptured areas are marked with arrows, MC is myocommata and E is endomysium.
Fresh meat cuts show a compact structure of muscle fi bers, with little separation of endomysium. However; in the myocommata of the river fish, in addition to a mild disorder, an interfibrilar separation effect was observed that was more pronounced in the myocommata than in the farmed fish (Fig. 2A and B). The effect of the low tempera ture and storage time on the tissue was evidenced by the degradation of larger areas. When the samples were stored for 13 h at 0°C (Fig. 2C and D), the separation between the endomysium, myosepta and myotomes (arrows) was higher than in the fresh samples, showing a minor effect on the river fish, probably because these were older, factor that affects collagen composition. Moreover, when the cold storage time was equal to 61 h (Fig. 2E and F), the tissue from the farmed fish showed greater involvement in the histological structure and myocommata, showing almost total degradation. While the muscle samples of the river fish, although they had interfibrilar collagen loss, had a defined structure.
Water retention capacity (WRC) of yamu meat samples
The water retention capacity values (WRC) of the yamu meat under different storage conditions can be seen in figure 3.
The WRC of the yamu meat was affected by the storage time (P<0.05) where the highest value, besides the value for the fresh meat, was seen in the fillets of the river fish, stored for 13 h at 0±1.5°C and the lowest value was seen in the river fish fillets stored for 61 h at 0±1.5°C (29.7%). Moreover, the origin of the fish (river or farmed) and stor age temperature treatment had no impact on the variation of the WRC (P>0.05); the interactions between the treat ments did not have an effect on the WRC either (Fig. 3).
Texture analysis of the yamu meat
The texture results for the yamu meat under different storage conditions are in figure 4.
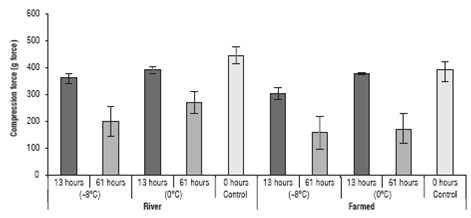
FIGURE 4 Texture analysis of the yamu meat subjected to different storage conditions. The errors bars correspond to standard deviation.
The values indicated that the texture of the yamu meat was affected both by the origin of the fish and by the cold storage period (P<0.05); there was also a significant effect from the interaction of the 3 factors on the texture of meat. The origin only affected the texture when the storage time reached 61 h. At 13 h of storage, there were no significant differences (P>0.05) for texture between the farmed fish and the river fish.
Determination of pH in the yamu meat
The analysis of the pH values of yamu fillets stored under different conditions are in figure 5.
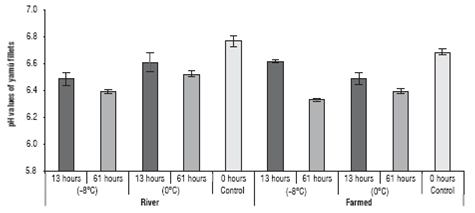
FIGURE 5 pH variation in the yamu fillets stored at different temperatures. The errors bars correspond to standard deviation.
The pH values were affected by two of the three factors (origin and treatment time), as well as by the interaction of all factors (P<0.05). However, the origin of the fish had an important effect on the pH variation of the fillets after storage for 61 h.
The values showed that the pH of the farmed fish meat ranged from 6.54±0.07 to 6.37±0.03 when stored for 13 and 61 h, respectively. The river fish had a pH of between 6.51±0.08 and 6.48±0.09 for fish preserved at 13 and 61 h, respectively.
Protein degradation
The protein profile analysis of tissue extracts of yamu meat (Brycon amazonicus) under different storage conditions is in Fig. 6 and 7.
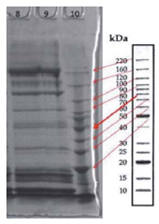
FIGURE 6 Protein profile of tissue extracts of yamu meat (Brycon amazonicus). Lane 8: protein profile of Brycon amazonicus from the ri ver, lane 9: protein profile of farmed Brycon amazonicus.
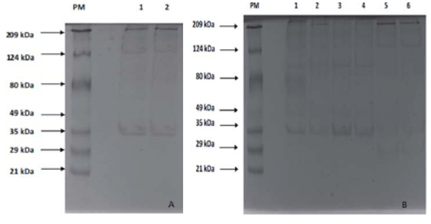
FIGURE 7 10% SDS-PAGE Electrophoresis. Colloidal Coomassie was used for staining; 30 /jg of protein were placed in each lane. Fig. 7A shows the protein profiles of the samples stored at 0±1.5°C for 13 h. PM lane: molecular weight marker. Lane 1: Fresh meat from the river; lane 2: farmed fresh meat. Fig. 7B: PM lane: molecular weight marker; lane 1: meat from the river stored at 0±1.5°C for 61 h; lane 2: farmed meat stored at 0±1.5°C for 61 h; lane 3: meat from the river stored at -8±1°C for 13 h; lane 4: farmed meat stored at -8±1°C for 13 h; lane 5: meat from the river stored at -8±1°C for 61 h; lane 6: farmed meat stored at -8±1°C for 61 h.
The electrophoresis showed 14 bands. Slight degradation was observed of these proteins in the samples with cold storage (Fig. 7A), with a greater effect on the samples pre served for 61 h at a lower temperature (-8±1°C) (lanes 5 and 6). The 222 kDa band (Fig. 7B, lane 2), similar to the heavy chain myosin, was in all of the treatments and, although the intensity of the band decreased, lower molecular weight bands were seen (212.69 kDa; 172.87 kDa; 171.96 kDa; 147.05 kDa; 135.96 kDa, 124.32 kDa, and 112.44 kDa) in the samples with cold storage. In this sense, it is possible to conclude that there was no complete degradation. In the samples preserved at 0±1.5°C for 13 h, there was not a defined band of a molecular weight similar to that of actin (~45 kDa), but the appearance of 34.06 kDa and 30.30 kDa bands evidenced a phenomenon suggesting actin degrada tion. Bands representing a-actinin (~105 kDa) stopped appearing after storage for 13 h (Fig. 7A and 7B); however, a similar weight band appeared in the samples of the river fish and farmed fish stored at 0±1.5°C for 61 h and -8°C for 13 h, respectively. This band did not have the intensity of the actin bands reported in the literature or found in the initial profiles, indicating that it is a fragment of another protein with a higher molecular weight.
Discussion
The scanning electron microscopy and optical analysis results showed that, at a lower temperature, the effect on the Brycon amazonicus muscle tissue was greater. The loss of collagen and the formation of interfiber spaces and their size were the effect of low temperatures, showing significant difference between the treatments when comparing the samples stored at -8±1°C and 0°C during the same storage time. The samples stored at -8±1°C (Fig. 2 and 3) showed a loss of myocommata and interfibrilar tissue and the formation of spaces in the myocommata and between the myomeres. At this temperature, the effect of the origin of the fish was irrelevant since the chilling effect was dramatic and similar in all of the fish. This phenomenon of degrada tion of myofibrils connective tissue in Brycon amazonicus meat subjected to processes of cold storage is a phenomenon that has been reported previously for different species of fish with cold storage at different temperatures and for different times (Caballero et al., 2009; Ofstad et al., 2006; Papa et al., 1997; Taylor et al., 2002) and is explained as an effect of proteoglycan degradation of the matrix, where the cause is the structural decomposition of the collagen network, generating muscle structure failure (Ofstad et al., 2006). Similar results were reported by others authors for sea bream fillets (Sparus aurata) stored at 4°C for 2 d and for salmon fillets (Salmo salar L.) kept on ice for 1 d (Taylor et al., 2002).
For the water retention capacity, the values differed from those obtained for European hake fillets (Merluccius mer-luccius) stored at -40±1.5°C for 25 weeks, where the water retention capacity, although reduced in capacity, remained above 40% (Sánchez-Alonso et al., 2012). There were dif ferences in the values obtained for this species, stored at different temperatures for different periods of time (0-48 weeks), which reported that the effect of storage time also affected the WRC of that species (Sánchez-Valencia et al., 2014). The fact that the WRC values reported by these au thors are greater than those obtained in our research clearly shows that the WRC of yamu meat undergoes dramatic changes when it is subjected to cold storage processes, es pecially at temperatures below 0±1.5°C. This phenomenon is caused by alterations in the protein/water caused by exposure of the aliphatic groups in the protein secondary structure complex (Careche et al., 1999). These events oc cur as a result of protein degradation that is not controlled with storage temperature, which clearly explains why only the storage time causes a reduction in the WRC because, although the fillets were kept at temperatures below 0°C, the enzymatic action that hydrolyzes the protein complex that helps retain water still remains active.
The texture analysis of the yamu meat showed values ob tained with the compression force of the yamu meat that were higher than those reported for trout (Oncorhynchus mykiss) kept on ice for 5 d and which were between 32 to 116 (g-force) (Godiksen et al., 2009); for Brycon cephalus, after storage at -3°C for 12 h, values between 96 and 128 (g-force) were reported (Suárez et al., 2007) and for six distinct species stored at 5°C for 72 h (Ando et al., 1999). However, these values are lower than those reported for Atlantic salmon (Salmo salar) frozen and chilled pre and post mortem rigor, which ranged between 846.4 and 1070.7 (g-force) (Einen et al., 2002). Likewise, the values of this research coincide with those reported by Liu et al. (2013), who reported average values of 350 and 430 g for grass carp meat stored at -3°C and 0°C, respectively. Thus, the yamu meat showed a more drastic change than other authors reported, coinciding with Suarez-Mahecha et al. (2007), indicating that the meat of animals from the Brycon genus can be highly affected by cold storage, more so than the meat of other fish species. This loss of texture is highly related to the decrease in the WRC because hydrolyzed myofibrillar proteins lose the structure that gives support and rigidity to the meat and in effect, the resistance to compression analysis is reduced.
The pH values were different from those reported by other authors (Jain et al., 2007; Ocano-Higuera et al., 2009) who worked with rohu (Labeo rohita) and dogfish shark (Mus-telus lunulatus) stored on ice for 8 and 18 d, respectively. These researchers showed that the pH of the meat of these species increased as storage time passed; however, in our research, the pH of the meat decreased over time. In addi tion, the results were similar to that reported for cod (Gadus morhua) kept at 0, 5, 10, and 15°C for 400 h (Heising, 2014). This decrease in pH suggests that the fish were subjected to cold storage before rigor mortis began and the decline was the result of the conversion of glycogen to lactate during postmortem glycolysis (Einen et al., 2002).
Biochemical changes play an important role in the texture of fish fillets. In particular, the rapid post-mortem acidifi cation of anaerobic glycolysis and a low final pH have been associated with a loss of texture (Ang and Haard, 1985; Kiessling et al., 2004), possibly because of the reduced strength of the connective tissue, the denaturation of pro teins and increased proteolysis (Ofstad et al., 2006).
The behavior of myofibrillar protein analysis of Brycon amazonicus with cold storage (0°C and -8°C) was similar for the river fish and the farmed fish, but by storing the fillets longer and at lower temperatures, the appearance of lower molecular weight bands (71.08 and 64.78 kDa) was evident, suggesting some degradation of higher molecular weight proteins, such as troponin (~78 kDa) (Fig. 7b, lanes 3-6).
The protein patterns were similar to those reported by other authors (Ball and Johnston, 1996; Ladrat et al., 2003; Lund and Nielsen, 2001; Ogata et al., 1998; Swartz et al., 1997), within which proteins have been reported, such as heavy chain myosin (WHC ~200 kDa) (Cao et al., 2006; Liu et al., 2013), a-actinin (~105 kDa) (Ladrat et al., 2003),
actin (~45 kDa) (Cao et al., 2006) and troponin (~78 kDa) (Ladrat et al., 2003).
Conclusions
There was degradation of proteins, such as heavy chain myosin, actin and a-actinin, in the cold storage of the yamu meat. In addition, the origin of the fish and the storage temperature had a significant effect on the texture changes, and the storage time had a significant effect on the three technological characteristics of the meat. The storage temperature did not significantly affect the water retention capacity.