Introduction
The cape gooseberry (Physalisperuviana L.) is also known as goldenberry, uchuva, uvilla, and aguaymanto, among many other names. It is an exotic fruit of the Solanaceae family that is distributed worldwide, with the greatest diversity in South America (Olmstead, 2013). P. peruviana is a tetraploid species (2n = 4x = 48) although genotypes with 2n = 2x = 24 have been reported, suggesting that the basic chromosome number is x = 12 (Rodríguez and Bueno, 2006; Liberato et al., 2015).
The cape gooseberry fruit is quite well regarded because of its nutritional value, which has high concentrations of vitamins A, C and B complex, along with phosphorus, calcium, and iron. Additionally, bioactive compounds, such as withanolides, phenolics, and ethanol derived substances, that are found in extracts from the leaves provide antifungal, antioxidant, antitumor and anti-inflammatory health benefits (Ligarreto et al., 2005; Wu et al., 2006). The cape gooseberry has gained commercial significance in Colombia, where it was widely introduced into cultivation in 1985, mainly for the domestic market as fresh fruit.
Colombia, one of the major producers of this fruit together with India, Vietnam, and South Africa, is the top exporter of this crop (Patiño et al., 2014).
Anther culture is an effective alternative technique for producing doubled haploid (DH) lines in cultivated crops (DH technology). The production of haploid plants from heterozygous genotypes, followed by chromosome doubling, allows for the fixation of traits without multiple selfing generations; this shortens the breeding time and facilitates the development of new varieties (Murovec and Bohanec, 2012).
In cape gooseberry breeding programs, the anther culture methodology was employed as a complement to conventional methods for homozygous line production (Suescún et al., 2011). However, in one study, only 28% of anther-derived plants presented spontaneous chromosome doubling (Sánchez, 2014). This is an important factor to consider in the application of anther culture technology because breeding programs require a large number of homozygous DH plants with a high level of fertility; therefore, it is necessary to induce chromosome doubling in the cape gooseberry.
Several doubling agents have been used to induce chromosome doubling in species from the Solanaceae family, including oryzalin (Chauvin et al., 2003) and trifluralin (Amiri et al., 2010). However, the most commonly used chemical agent to induce chromosome doubling is colchicine (Murashige and Nakano, 1966; Ojiewo et al., 2006; Amiri et al., 2010), which disrupts mitosis by inhibiting the formation of spindle fibers, resulting in chromosome doubling (Seguí-Simarro and Nuez, 2008). There are no reported protocols for colchicine-mediated doubled haploids in the cape gooseberry although Wenzel (1973), Díaz et al. (2008) and Franco (2012) reported high levels of au-topolyploidy after an in vivo treatment of tetraploid plants. In the present research, four haploid cape gooseberry genotypes were used to evaluate artificial chromosome doubling induced by a colchicine treatment and the effects on the number of chloroplasts per guard cell, number of chromosomes and fertility with the purpose of developing an efficient and reliable artificial chromosome doubling protocol for anther-derived cape gooseberry haploids.
Materials and methods
Plant material and growth conditions
Haploid cape gooseberry plants were produced with the anther culture technique. Four haploid genotypes: 16U490, 16U520, 16U793 and 16U802 were selected based on phenotypic observations in a greenhouse. They were sterile and exhibited a lower plant vigor than anther-donor plants. In vitro plants of the four genotypes were sub-cultured every four weeks in an MS medium (Murashige and Skoog, 1962) supplemented with 50 g L-1 sucrose, 0.1 mg L-1 indole-3-acetic acid (IAA, 3-IAA) and 0.5% (w/v) phytagel at pH 5.8 and incubated under light condition using a 16 h photoperiod at 21 ± 2°C.
Colchicine treatment and plant regeneration
Axillary buds from the haploid genotypes were excised and immersed for 2, 4 or 6 h in the dark, in an aqueous solution of colchicine at 5, 10 and 15 mM concentrations. The solution was dissolved in 2% dimethyl sulfoxide (DMSO) and sterilized with filtration using a 0.22 μm MILLEX® (Merck KGaA, Germany) membrane. Then, the removed buds were rinsed for 2 min, using three 10 mL changes of deionized sterilized water.
Fifteen axillary buds of every haploid genotype were used in each treatment. The same number of plants of each hap-loid genotype were grown in DMSO without a colchicine treatment, serving as controls. The control explants were maintained in a Murashige and Skoog (MS) medium, while the colchicine-treated buds were maintained in an MS medium supplemented with 0.1 mg L-1 of indole butyric acid (IBA) and sub-cultured once. In this step, the survival rate of the treated buds was evaluated.
Shoots that developed were transferred to Canadian peat and maintained in a moist chamber at a temperature of 24°C with a high relative humidity of 80% and normal light for 2 weeks; the shoots were then placed under shading for 2 more weeks under greenhouse conditions. The plants were transplanted to a soil-rice hull mixture (3:1), then irrigated and weeded as needed until they produced 4 to 5 flowers at a developmental stage that was suitable for evaluating the pollen fertility.
Chromosome and chloroplast number evaluation
The chromosome numbers (chrs) of the regenerated plants were determined with chromosome counting using root-tip meristem squashes, and the chloroplast number (chls) was determined with stomatal guard cell observations. For the squashes, root samples 1 cm in length were collected in the early morning from plants in the growth room. Each sample was placed in 0.25% colchicine solution dissolved in 2% DMSO for 3 h at room temperature. After rinsing 2-3 times with tap water, the root samples were transferred to deionized distilled water in a temperature-controlled water bath at 37°C for 1 h. Then, the roots were fixed in 3:1 ethanol-acetic acid and stored at 4°C for 12 h, hydrolyzed in 1N HCl for 25 min and rinsed again 4 to 5 times with tap water. The root tips were excised and squashed in 2% propionic orcein stain. The slides prepared for chromosome counting at the metaphase stage were examined under a Zeiss Axiostar-plus microscope (ZEISS, Germany), and the chromosome counts were done on about 25 nuclei.
In order to count the chloroplast number, the epidermis was peeled from the abaxial surface of the leaves and placed in a drop of 1:1 KI-I solution, previously dissolved in 70% ethanol and covered (but no squashed) with a coverslip. The total number of chloroplasts per guard cells was counted with a Zeiss Axiostar-plus microscope, observing at least 25 guard cells.
Pollen fertility
To check fertility, the pollen viability and in vitro pollen germination were determined in the anther-donor, haploid and colchicine-treated plants. First, the pollen was collected from 5 flowers per plant during anthesis, distributed on a glass slide with a drop of acetocarmine and covered with a coverslip. The pollen grains were observed through an optical microscope (Zeiss Axiostar-plus microscope x100 magnification) and subsequently classified as fertile or not fertile. The pollen grains with aberrant morphology, little staining and reduced and/or absent protoplasm were considered sterile, while those that displayed intact cell walls and deeply stained protoplasm were considered fertile. This evaluation was performed on 100 pollen grains per plant.
The in vitro germination was assessed with the hanging drop method. The pollen germination and pollen tube growth were determined by placing a small drop of germination media on a cover glass, and pollen grains were placed on the drops with a clean dissecting needle. The pollen was incubated under dark conditions at 25°C in a culture medium containing 0.1% sucrose, 0.1% boric acid (H3BO3), and 2 ml Tween 80 for 6 h at 21°C (Grisales et al., 2010). After this period, 200 pollen grains were counted in a random space with a Zeiss Axiostar Plus microscope to estimate the mean number of germinated and non-germinated pollen grains. For purposes of analysis, pollen grains whose tubes reached the same or greater length than their own diameter were considered vital or germinated (Báez et al., 2002).
Data analysis
The experiment design was completely randomized in a factorial arrangement of 4 genotypes χ 3 colchicine concentrations χ 3 exposition times, for a total of 36 treatments with 3 replicates. Each replicate was represented by a glass container with 4 buds. Analysis of variance (ANOVA) and Pearson correlation were carried out using the SAS software (Statistical Analysis System version 9.1.3, Cary, NC, USA). P-values of less than 0.05 were considered statistically significant.
Results and discussion
Survival rate and plant regeneration
The survival rate of the treated buds gradually decreased when treated with a high concentration of colchicine and a prolonged immersion period (Tab. 1). The regeneration rate of buds treated with colchicine at a concentration of 5-10 mM was above 40%, while a 33% survival rate was obtained with the 15 mM colchicine at 6 h for genotype 16U802. The colchicine delayed bud development and growth in the first month after treatment. However, after the subculture step, the treated buds grew normally. The treatment with 15 mM of colchicine significantly decreased the plant regeneration frequency. In addition, the highest plant regeneration frequency was achieved using the colchicine treatments of 5 and 10 mM for 2 h. Significant differences were detected in the plant survival rate as a result of the colchicine concentrations (P≤0.0001) and exposition time (P = 0.0257), while no significant differences between the genotypes (P = 0.2481) were observed.
Chromosome number
The anther-donor plants were tetraploid and showed 2n = 4x = 48 chromosomes (Fig. 1A), while the haploid plants contained gametic chromosome complement and presented 2n = 2x = 24 chromosomes (Fig. 1B). The regenerated plants from the treated buds presented an increase in chromosome number and a high frequency of chimeras, with different ploidy levels in the same tissue (mixoploid plants). Mixoploid plants were obtained in all of the colchicine treatments; however, they presented a dominant frequency of chromosomic numbers of 24, 36, 42 and 48 (Tab. 2).
TABLE 2 Comparison of traits in anther-donor, haploid and mixoploid genotypes of cape gooseberry.
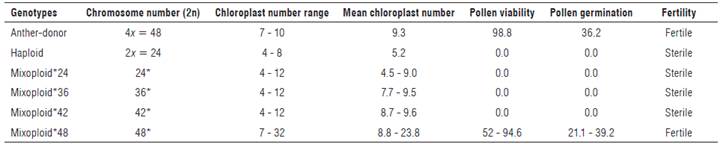
*Larger number of cells with the indicated chromosome number.
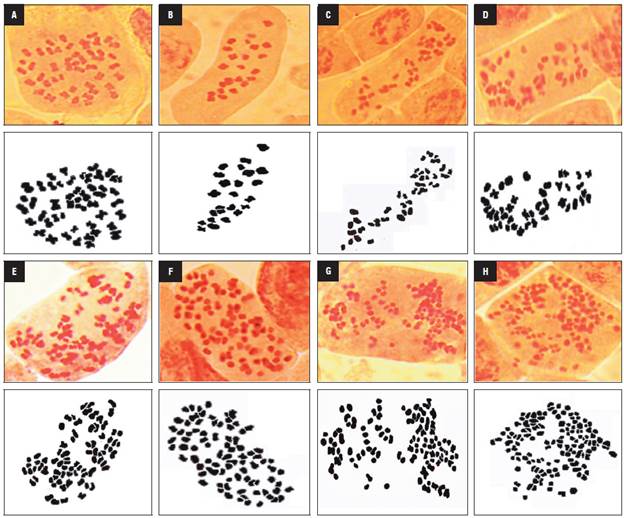
FIGURE 1 Somatic chromosomes in root-tip cells of cape gooseberry. A) Chromosomes of anther-donor genotypes with 48 chromosomes (chrs), B) haploid genotypes with 24 chrs and C) mixoploid genotypes with 39 chrs, D) 42 chrs, E) 78 chrs, F) 84 chrs, G) 102 chrs and H) 129 chrs.
In addition, aneuploid cells and cells with between 39 and 129 chromosomes were found in the mixoploid plants (Fig. 1C-H). The occurrence of aneuploid cells was significantly higher after the colchicine treatment with 15 mM for 2 h (13.6%), mostly in genotype 16U793. High numbers of chromosomes per cell, 102 and 129, (Figs. 1G-H) were obtained with the treatment with 15 mM colchicine for 2 h and 4 h, respectively; however, this treatment significantly decreased the frequency of regenerated plants (Tab. 1).
Chloroplast number
In the haploid plants, the number of chloroplasts (chls) ranged from 4 to 8, with an average of 5.2, while the tetraploid anther-donor plants had 7 to 10 chloroplasts. The mixoploid plants contained 4 to 32 chloroplasts per stomatal guard cell, with a mean that ranged from 4.5 to 23.8 (Tab. 2, Fig. 2). The chloroplast number was highest in one regenerated plant of genotype 16U793 (23.8 ± 3.3), followed by genotype 16U802 (18.4 ± 2.6), in the 15mM colchicine treatment with 2 h and 4 h, respectively.
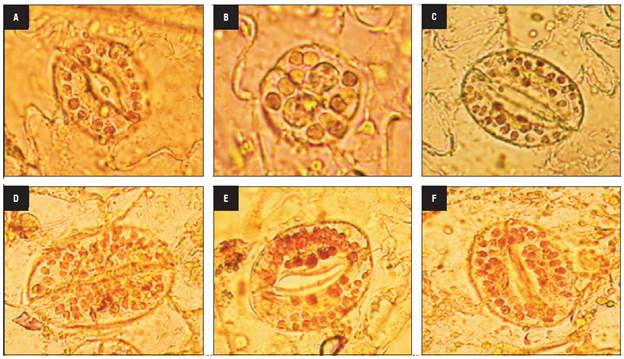
FIGURE 2 Chloroplast number per cape gooseberry stomatal guard cell. Chloroplast of anther donor A) Chloroplast of anther donor, B) haploid and C) mixoploid plants with 18 chls, D) 27 chls, E) 29 chls and F) 32 chls.
The mixoploid plants, with a dominant chromosome number of 24, 36 and 42, had 4 to 12 chloroplasts in the guard cell, with an average of less than 9.6, while the mixoploid plants with 48 chromosomes contained between 7 to 32 chloroplasts, with an average that ranged from 8.8 to 23.8. The Pearson correlation analysis showed a significant correlation (r = 0.61, P<0.0001) between the dominant chromosome number and the mean of the chloroplast number. This result suggests that the number of chloroplasts can be a useful marker for inferring the ploidy level.
Pollen fertility
The four haploid genotypes presented non-viable pollen grains with abnormal shapes and sizes that were lightly stained (Fig. 3A) and did not show in vitro germination as expected. Likewise, the mixoploid plants with 24, 36 and 42 chromosomes had non-viable pollen. In contrast, the mixoploid plants with 48 chromosomes were fertile, with pollen viability ranging from 52.04 to 94.6% (Fig. 3B) and had an in vitro germination percentage from 21.1 to 39.2% (Fig. 3C). The maximum germination percentage of -39% was reached with 10 mM colchicine for 4 h, followed by 10 mM colchicine for 6 h. The chromosome number correlated positively with the pollen viability (r = 0.72) and pollen germination (r = 0.73).
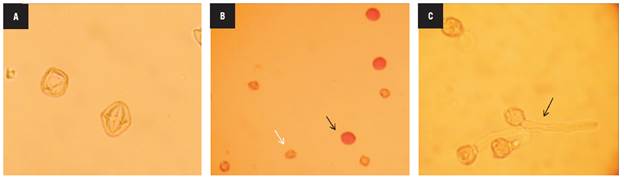
FIGURE 3 Pollen grain fertility of haploid and mixoploid cape gooseberry. A) Non-viable pollen grains of haploid genotypes, B) Viable and non-viable pollen grains of mixoploid plants, C) In vitro pollen germination of mixoploid plants (40x magnification).
The treatments of 5, 10 and 15 mM for 2 h produced the highest percentage of mixoploid fertile plants based on regeneration rates of 19, 13 and 16% for fertile plants, respectively. The third treatment (15 mM - 2 h) produced 70% partially fertile plants (defined as plants producing fertile and sterile flowers on different branches or consecutive nodes), while the 5 mM and 10 mM treatments for 2 h produced around 60% plants that were predominantly fertile (defined as plants having only fertile flowers). Consequently, the latter treatments were judged to be the optimal treatments. The mixoploid fertile plants reached 19% for 16U490, 16% for 16U520, 28% for 16U793 and 37% for the 16U802 genotype, which is indicative of genotype-specific response effects.
Morphological observations
Visual differences in the morphological characteristics, such as leaf size, flower bud shape, anther number, protruding pistil and fruit shape, were identified among the haploid and mixoploid plants with 48 chromosomes (Mixoploid*48). The leaf size of the haploid plants was smaller than in the mixoploid plants (Fig. 4A). The haploid flower buds were thinner and more elongated in shape than the mixoploid flower buds, which were rounded (Fig. 4B-C). The mixoploid flowers were bigger and sometimes presented six anthers, while the haploid plants always had five (Fig. 4D-E). The haploid plants did not produce seeds, but they formed small fruits in unfertilized flowers with an obcordate shape (Fig. 4F); while the fertile mixoploid plants produced normal ovoid-shaped fruit containing abundant seeds (Fig. 4G).
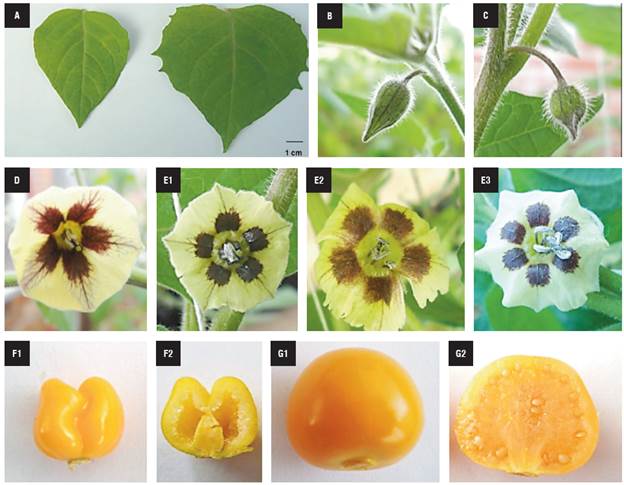
FIGURE 4 Morphological characteristics of haploid and mixoploid cape gooseberry. A) Haploid (Left side) and mixoploid leaf (Right side). B) Haploid and C) mixoploid bud flower. D) Haploid and E) mixoploid flower. F1) Haploid and F2) haploid fruit longitudinal cut. G1) Mixoploid and G2)mixoploid fruit longitudinal cut.
Haploid (Left side) and mixoploid leaf (Right side) (A). Haploid (B) and (C) mixoploid bud flower. Haploid (D) and mixoploid flower (E). Haploid (F1) and haploid fruit longitudinal cut (F2). Mixoploid (G1) and mixoploid fruit longitudinal cut (G2).
The applicability of a doubled haploid (DH) protocol is of great interest for cape gooseberry breeding programs; however, anther cultures provide a low frequency of spontaneous double haploid, as compared to haploid frequency. The regeneration of fertile DH plants from colchicine treated buds is a critical factor in breeding programs for the commercial production of this crop. The regeneration rate fluctuated from 33 to 100%, mainly because of high mortality after the colchicine treatment and different responses of the genotypes treated with the same colchicine concentration. The anti-microtubule colchicine not only led to mitotic restitution in the meristem, but also had severe effects on the viability of the material. This explains the high mortality in some treatments, as reported by Nattaporn and Sompong (2012) in orchids.
In this study, the colchicine application resulted in higher rates of chromosome doubling in all of the genotypes, especially in the 15 mM for 2 h treatment; however, different ploidy levels in the cells of the regenerated plants were also observed. These results are in accordance with those previously reported in cape gooseberry seedling treatments by Díaz et al. (2008) and in other plants, such as tobacco (Bürün and Emiroglu, 2008) and Brassica spp. (Smykalová et al., 2006). The chromosome doubling rate increased more significantly in the 16U793 genotype, suggesting genotypic dependence as described previously in wheat (Zamani et al., 2003) and Brassica spp. (Weber et al., 2005).
The chloroplast number in the stomatal guard cells of the haploid genotypes ranged from 4 to 8 chloroplasts, a lower value than in the anther-donor plants. Moreover, the mixoploid plants with 48 chromosomes presented more than 7 chloroplasts, the same as the tetraploid anther-donor plants. Additionally, a significant correlation was found between the number of chromosomes and chloroplast, suggesting that the chloroplast number can be an indirect and efficient indicator of ploidy in cape gooseberry plants. This correlation was also observed in tomato (Koornneef et al., 1989), pepper (Qin and Rotino, 1995) and sweet potato plants (Delgado-Paredes et al., 2017).
Furthermore, chromosome numbers and nuclear DNA contents in plant cell nuclei have been highly correlated in cape gooseberry plants and can be used as an index to estimate ploidy levels in cape gooseberry plants. Liberato et al. (2015) reported that cape gooseberry genotypes with 2n = 48 chromosomes presented between 5.77 and 8.12 pg, while a genotype with 2n = 24 chromosomes had 2.33 pg. Likewise, cytometry flow and chloroplast numbers have been correlated in watermelon (Sari et al., 1999) and poplar and black locust plants (Edwald et al., 2009), indicating that the technique of counting chloroplasts can be used effectively to determine ploidy levels and could replace chromosome counting and flow cytometry, especially when the number of samples is high.
Our results indicate that this in vitro approach can produce chimeric plants with sectors that are still haploid in predominantly fertile DH plants. These fertile plants are very useful for plant breeding programs, quickly producing new cultivars after a strict progeny test. The highest rate of fertile plants was observed for the 15 mM colchicine concentration when applied for 2 h, producing mostly partially fertile plants. However, the 5-10 mM for 2 h treatments generated mostly fertile plants, indicating that this treatment is the best for fertility recovery since it provided new genetic material for conventional plant breeding programs. Similar results were reported by Smykalová et al. (2006) in Brassica spp., who found inflorescences of chimeric plants containing both sterile and fertile flowers. Additionally, the 16U802 genotype presented more fertile plants (24%) than the other 3 genotypes, indicating that a genotype dependent factor was possibly involved.
In this study, the mixoploid plants had larger leaves and flowers and darker green leaves, but this could have been due to another attribute that was not accounted for, such as tolerance to an environmental stress that has been identified in this kind of plants (Kermani et al., 2003; Shao et al., 2003). Furthermore, the mixoploid flowers were bigger, probably because the cells with more ploidy grew more (Cheniclet et al., 2005) and maintained a constant ratio between the nuclear and cytoplasmic volume (Novakova et al., 2016). In addition, the haploid genotypes produced small fruits without seeds, as reported in haploid tomato plants (Ari et al., 2016).