Introduction
Maize (Zea mays L.) currently has a world production of 1,078,080,000 t per year. The United States is the principal producing country followed by China. In South America, Brazil is the main maize producer followed by Argentina and Bolivia (USDA, 2019). Colombia occupies fourth place with yellow maize production of 939,677 t in 2017, constituting the second largest cereal crop in the country (DANE, 2019). In Colombia, yellow maize crops represent about 326.326 ha nationwide (DANE, 2019). The province of Cundinamarca has 26,953 ha planted with yellow maize, and the Ubate Valley occupies the fifth place of planted areas (DANE, 2016).
The production of cereals like maize currently shows yield limitations due to various factors, such as environmental deterioration and climate change that increase pests and diseases in crops and threaten global food security (FAO, 2016). An important number of diseases in maize are caused by Fusarium species. Stalk rot is one of the diseases caused by Fusarium spp., and it is present in maize crops around the world (Fumero et al., 2015). Species of the genus Fusarium, among other organisms (that include fungi, bacteria and oomycetes) are related to this alteration and cause significant damage during the establishment stage of a maize crop (Shin et al., 2014). The symptoms of this disease are associated with affected internal stem tissue, characterized by brown streaks in the internodes at early stages and a pink discoloration in mature plants (Shin et al., 2014). Several Fusarium species, which include F. graminearum, F. temperatum, F. subglutinans, F. proliferatum and F. verticillioides, have been reported as associated with maize plants with symptoms of this disease (Shin et al., 2014; Gromadzka et al., 2016; Zhang et al., 2016; Gromadzka et al., 2018). Some of these fungal species can also produce mycotoxins and may be seed-transmitted, leading to significant yield losses and a decrease in seed quality (Leslie and Summerell, 2006).
The management of seed pathogens in maize can be achieved using several methods. Among these procedures, treatment with fungicides prior to planting is used to protect seeds from fungal infection as well as to limit fungal growth originating from seeds (Munkvold and O'mara, 2002). However, the control of fungal infection in cereal seeds has been managed by physical means such as thermal seed treatments (Coutinho et al., 2007).
There are many published studies on treatments for the control of seed pathogens. Much research around the world has been carried out on thermal seed treatments resulting in superior control of the incidence of pathogens (Daniels, 1983; Clear et al., 2002; Coutinho et al., 2007; Bennett and Colyer, 2010). Clear et al. (2002) evaluate the incidence of F. graminearum and the viability of wheat and barley sedes after treatment with dry heat at 50°C, 70°C, 60°C and 80°C. The authors find that dry heat treatments can eliminate F. graminearum completely from wheat seeds without a significant reduction of seed germination under treatments at 70°C and 80°C. In addition, F. graminearum is eliminated in barley seeds showing a significant reduction of germination under treatments of10 d at 80°C. Coutinho et al. (2007) evaluated the sanitary and physiological quality of maize seeds subjected to thermotherapy with hot water at 60°C for 5, 10 and 20 min. The authors report that heat treatments for 10 min and 20 min significantly reduced the percentage of occurrence of F. verticillioides in the seeds. Nevertheless, the longest thermal treatments (10 min and 20 min) significantly reduce the seed germination rate compared to treatments of 5 min. Bennett and Colyer (2010) evaluated treatments with dry heat at 60°C, 70°C and 80°C from 2 to 14 d and treatments with hot water at 90°C for 45 s to 180 s for disinfection of cotton seeds infected with F. oxysporum. The results show that as the temperature increases with dry heat, seed infection diminishes more quickly. However, treatments at 80°C result in significant alterations of seed germination and vigor. All treatments with hot water show a significant reduction in the incidence of infected seeds compared to untreated seeds. Treatments with water at 90°C for periods of less than 120 s and 150 s do not significantly reduce the vigor of seeds, but the treatments do reduce the incidence of infected seeds from 50 to 10%. These cited studies demonstrate that thermal treatment of seeds could be a promising alternative for controlling maize seed pathogens.
Regarding the use of chemical products for the control of pathogens of the genus Fusarium,Broders et al. (2007) evaluated under in vitro conditions the sensitivity of F. graminearum strains isolated from maize seeds to the fungicides azoxystrobin, captan, trifloxystrobin, and fludioxo-nil. In this study, fludioxonil at 1.0 mg L-1 completely controls the radial growth of F. graminearum colonies, while azoxystrobin and trifloxystrobin do not exert control even at higher doses (100 mg L-1). These authors also report that captan exerts greater control than strobirulins at a dose of 200 mg L-1 without generating a complete inhibition of the radial growth of this species.
Ivić et al. (2011) report prochloraz (1.0 mg L-1) as the most effective fungicide among carbendazim, tebuconazole, flutriafol, and metconazole for the inhibition of F. graminearum mycelia growth when F. graminearum, F. avenaceum and F. verticillioides isolates from wheat sedes are evaluated.
Solorzano and Malvick (2011) find that treatments of maize seeds with active ingredients fludioxonil and azoxystrobin improve the germination, emergence and yield of maize plants. Germination values of 81% are obtained under fludioxonil and azoxystrobin compared to values of 74% in control treatments. Emergency values of 89.6% and 85.4% are achieved under seed treatments with azoxystrobin and fludioxonil + mefenoxam respectively, compared to untreated seeds (79.6%). The highest yield values are obtained under treatments with azoxystrobin and fludioxonil compared to control treatments.
Shin et al. (2014) evaluated the in vitro efficacy of fungicides tebuconazole, difenoconazole, fluquinconazole, azoxys-trobin, prochloraz and kresoxim-methyl for the control of F. temperatum and F. subglutinans associated with stem rot in maize plants. As a result, the authors find that fungicides of the azole group tebuconazole and prochloraz completely inhibit F. subglutinans and F. temperatum mycelial growth at a dose of 10 µg ml-1. On the other hand, the fungicide kresoxim-methyl limits F. subglutinans and F. temperatum colony formation at concentrations of 0.1 and 0.01 µg ml-1, respectively. The four azole fungicides completely inhibit the formation of F. subglutinans colonies at higher doses (1.0 µg ml-1).
Some of the maize diseases in Colombia caused by fungi such as Fusarium have an impact on both crop yield and the quality of the seed used by farmers (Varón and Sarria, 2007). Diseases caused by Fusarium species have been reported affecting maize plants in the provinces of Antioquia, Cordoba, Cundinamarca, Santander, Tolima and Valle, showing symptoms associated with stalk and ear rot (Buriticá 1999; Arrieta et al., 2007; Varón and Sarria, 2007).
Nowadays, maize crops in the Ubate Valley in Cundinamarca show symptoms of stalk rot, causing low crop yields. Observations performed in the area in 2017 showed that the incidence of this disease can vary between 9% and 44%, and its impact on production may vary depending on the agronomic management of the crop. Information on the phytosanitary status of the seeds used by producers in this region is scarce and guidelines for seed management have not been defined despite the importance of maize seeds on the dissemination of Fusarium species (Duncan and Howard, 2010). Therefore, it is necessary to develop, evaluate and implement sustainable management practices for maize crops in producer areas. Due to the importance of the disease and the lack of management strategies regarding seed treatment, the objective of this study was to determine the effect of thermal treatments on Simijaca variety maize seeds in the presence of pathogens of the genus Fusarium. Additionally, the effect of thermal treatments on seedling growth and development was assessed. Finally, the in vitro response of F. graminearum and F. subglutinans isolates from maize plants was evaluated at different doses of commercial fungicides.
Materials and methods
The study was conducted at the Malherbology, Plant Clinic and Phytopathology laboratories of the Faculty of Agricultural Sciences at the Universidad Nacional de Colombia, Bogota campus, during the second and first semester of 2017 and 2018.
Evaluation of the effect of thermal seed treatments on the presence of pathogens of the genus Fusarium on seedling growth and development
Naturally infected maize (Zea mays L.) seeds of the regional variety Simijaca provided by farmers from the Ubate Valley in Cundinamarca were used as plant material. Prior to the treatments, the seeds were soaked in water for 4 h to facilitate heat conduction in the seed tissues (Daniels, 1983; Coutinho et al., 2007). The seeds were then subjected to thermal treatments with two heat sources, hot water and dry heat at temperatures of 50°C, 55°C and 60°C to control for the presence of Fusarium in maize seeds, following the methodologies of Bennett and Colyer (2010). An evaporator (Water B-480, BÜCHI Labortechnik AG, Switzerland) was used for seed treatment with hot water. In this case, the seeds were wrapped in gauze and immersed in water at the desired temperatures for 5 min (Coutinho et al., 2007). For treatments with dry heat, the seeds were placed in a heat chamber (Model FD 23, BINDER, Germany) at the three temperatures to be evaluated for 10 min (Bennett and Colyer, 2010). Once the heat treatment was carried out, treated and untreated seeds (controls) were disinfected with 5% sodium hypochlorite for 1.0 min, 70% ethanol for 3 min, and sterile distilled water for 2 min following the methodology proposed by Murillo and Munkvold (2008). Seeds were then incubated in Blotter chambers consisting of plastic boxes with absorbent paper moistened with sterile distilled water kept at room temperature (± 20°C) for 10 d (Warham et al., 2008). Each chamber contained 40 seeds, and two chambers were used per treatment. A total of seven treatments were evaluated using untreated seeds as controls. The experiment was replicated three times.
The methodologies of Bennett and Colyer (2010) were adapted for the evaluation of the effect of thermal seed treatments on maize seedlings growth and development. The variables of germination percentage, seedling length and fresh weight of 80 seedlings were evaluated 10 days after treatment (dat). The germination percentage was calculated according to Khodarahmpour (2012). Seedling length was measured from the coleoptile to the longest root with a caliper (Lopes et al., 2018), and fresh weight of 80 seedlings was estimated with a precision balance scale (EWB 220-2M, Kern, Germany).
The presence of pathogens of the genus Fusarium in maize seeds was evaluated by the severity index in terms of mycelial colonization of the seed, using a diagrammatic severity scale of five grades (Machado et al., 2013). Visual assessments were performed at 10 dat in search of symptoms and signs of the pathogen. Those seeds that had the classic "Starburst" symptom, which consists of white striae in the pericarp as a product of cell disintegration accompanied by white or pink mycelium colonizing the grain, were considered as affected (Murillo and Munkvold, 2008; Duncan and Howard, 2010). Finally, to corroborate the presence of fungi of the genus Fusarium in the treated and untreated (control) maize seeds, seeds were sown in a selective medium of Potato Dextrose Agar (PDA) + benzoxazolin-2(3H)-one (BOA), reported for maize pathogens of the species F. graminearum, F. subglutinans, and F. verticillioides due to their capacity to degrade BOA and grow on the surface of the amended agar (Vilich et al., 1999; Glenn et al., 2001; Leslie and Summerell, 2006). Subsequently, for the confirmation of the Fusarium species, hyphal tipping was performed from mycelium grown in the PDA+BOA media for the evaluation of coloration and structures formed in PDA and Carnation Leaf Agar medium (CLA) following Leslie and Summerell (2006) and Warham et al. (2008).
In vitro evaluation of the effect of commercial fungicides on isolates of pathogens of the genus Fusarium
Four monosporic isolates were used (143A and 26B of F. graminearum, and 45D and 186A of F. subglutinans), which were previously obtained from maize plants showing symptoms of stalk rot in commercial and semi-commercial crops of the Ubate Valley in 2017. These isolates were used to evaluate the effect of different commercial fungicides on the inhibition of radial growth at 20 days after sowing (das) and the inhibition of conidial germination at 24 h. The active ingredients and commercial doses of the study were as follows: fludioxonil + metalaxyl-M (100 ml/100 kg of seed), tebuconazole + trifloxystrobin (16 ml/100 kg of seed), prochloraz + difenoconazole (1.5 ml/100 kg of seed) and carboxin + captan (125 g/100 kg of seed). These active ingredients are used in maize crops and some are registered for seed treatment (ICA, 2019). Three doses were evaluated: 0.5, 1.0 and 1.5 the commercial doses (CD) of each of the fungicides of interest. The methodologies used by Nisa et al. (2011) were adapted for the evaluation of radial growth inhibition of the colonies. For this purpose, the fungicides were added after the PDA medium was autoclaved and cooled down to 50°C. Then, disks of 5 mm diameter were extracted from 10-day colonies of each of the isolates to be evaluated and placed in the center of Petri dishes with PDA medium (CM0139, Oxoid®) amended with the concentrations of each of the four fungicides for evaluation (Nisa et al., 2011). Petri dishes with PDA medium without fungicides were used as controls. Three plates were used per treatment and placed in an incubator (MLR-351H, SANYO, Japan) at 24°C ± 2.0°C. The experiments were replicated three times. The radial growth of the colonies was measured every 48 h during 20 d to calculate the percentage of inhibition by Equation 1 after Plascencia et al. (2003):
where:
% Ix: percentage of radial inhibition.
Xc: mean radius (mm) of the control colony.
Xi: mean radius (mm) of colonies in media with fungicide.
The methodology for the evaluation of conidial germination inhibition was adapted from Steinkellner et al. (2005). Sterile 12-well culture plates (Nest® Cat. No. 712001, China) were used. In each well, 1000 µl of the fungicide solutions were placed in a mixture with 200µl of the conidial suspension, maintaining the respective doses to be evaluated. Macroconidia for the suspensions of each of the isolates were taken from 8-day cultures in the CLA medium (Retana et al, 2018) incubated at 22°C ± 2.0°C (MLR-351H, SANYO, Japan). The suspension was adjusted to 5x105 macroconidia ml-1 sterile distilled water using a haemocytometer (Steinkellner et al., 2005). Three wells were used per treatment and the plates were incubated at 24°C ± 2.0°C in the dark under permanent agitation at 200 rpm (Inkubator 1000 - Unimax 1010, Heidolph, Germany) (Steinkellner et al., 2005). Conidial germination was determined after 24 h by randomly evaluating 100 conidia per well of each treatment under a microscope (CX 31, Olympus, Japan). Conidia were considered germinated if the germ tube length was as long as the spore (Steinkellner et al., 2005). Subsequently, the percentage of conidial germination inhibition of each treatment was determined to estimate the efficacy of fungicides. This percentage was estimated using Equation 2 taken from Plascencia et al. (2003):
where:
% Is: percentage of inhibition of conidial germination.
% Sc: percentage of germinated conidia in control at 24 h.
% St: percentage of germinated conidia in media with fungicide at 24 h.
Statistical analysis
The evaluation of the thermal seed treatments was performed under a completely randomized factorial design with seven treatments and three replicates per treatment. For in vitro tests with commercial fungicides, a completely randomized factorial design was proposed with four treatments and three replicates per treatment. The results obtained were subjected to an ANOVA for all the trials with a value of P≤0.05. The differences between the mean results were calculated with the Tukey test (P≤0.05). The Levene test was performed to check the homoscedasticity of the obtained data and thus be able to perform an analysis of variance. Data were analyzed with the statistical package R Studio® version 3.5.1.
Results and discussion
Effect of thermal treatments of maize seeds on pathogens of the genus Fusarium
The symptoms and signs that were observed and evaluated for the determination of the severity index are presented in Figure 1. The presence of fungi of the genus Fusarium in treated and untreated maize seeds was corroborated by sowing seeds in PDA + benzoxazolin-2(3H)-one (BOA) medium (Fig. 2). As shown in Figure 2, in all the media amended with BOA no growth of yeasts, bacteria, and filamentous fungi was observed, showing the exclusive growth of Fusarium species. These fungi could correspond to the species F. graminearum, F. subglutinans, and F. verticillioides because of their growth on the BOA amended agar medium (Vilich et al., 1999; Glenn et al, 2001).
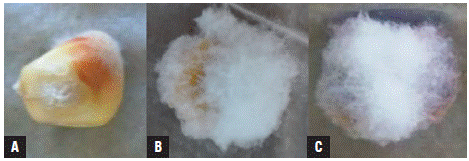
FIGURE 1 Mycelial colonization in maize seeds of the regional variety Simijaca 10 days after treatment under Blotter chamber conditions. A) detail of the “starburst” symptom or white striae in the pericarp as a product of cell disintegration with pink coloration on the pericarp. A, B and C) white and pink mycelium colonizing the maize seed.
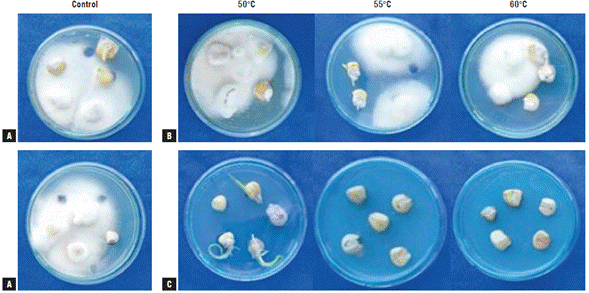
FIGURE 2 Maize seeds of the regional variety Simijaca subjected to heat treatments by two methods at temperatures 50°C, 55°C, and 60°C. A) control. B) dry heat treatment. C) hot water treatment. The figure shows colony growth of pathogens of the genus Fusarium in Potato Dextrose Agar (PDA) + benzoxazolin-2(3H)-one (BOA) medium 15 days after sowing (das).
In addition, cultures of these colonies in PDA medium initially exhibited abundant white mycelium that later changed to orange or purple colorations with dark violet close to black pigmentations in the agar. Orange sporodochia and macroconidia with three to four septa, curved apical cells and poorly developed basal cells (as described for F. subglutinans) were observed in CLA medium (Leslie and Summerell, 2006). Other colonies showed the growth of yellow or orange mycelium with a feathery appearance that eventually formed reddish pigments on the agar. The presence of pale orange sporodochia containing macro-conidia with five to six septa moderately curved, with the ventral parts straight and the dorsal parts arched, the basal cells in the shape of a foot and the straight apical cells corresponded to species of F. graminearum were observed in CLA medium as reported by Leslie and Summerell (2006).
The statistical analysis showed significant differences between the heat sources and the different temperatures evaluated with interaction between these two factors (P<0.05) for the variable severity index (P=0.00323). The effect of thermal treatments on the seed severity index in terms of the percentage of area colonized by Fusarium fungi is shown in Figure 3. According to the results, the severity index 10 dat with hot water compared to dry heat corresponded to 3.2% and 16.8% at 50°C, 2.0% and 9.9% at 55°C and 1.4% and 8.0% at 60°C, respectively, in contrast to values of 15.6% in the control treatment (Fig. 3). The severity index was significantly lower in seeds treated with hot water at the three evaluated temperatures and in seeds treated with dry heat at 60°C compared to untreated seeds (control).
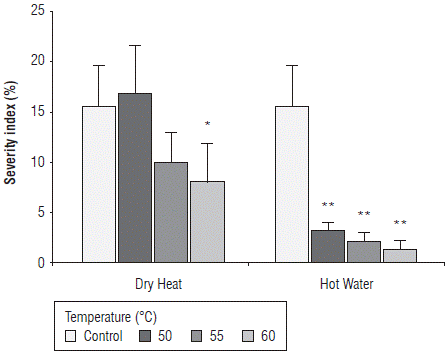
FIGURE 3 Effect of thermal treatments applied by two methods, dry heat and hot water, at temperatures 50°C, 55°C, and 60°C on the severity Index of maize seeds of the regional variety Simijaca in terms of percentage of mycelial colonization under laboratory conditions 10 days after treatment (dat). Significant differences between treatments and control are either denoted as * (P<0.05) or ** (P<0.01). Bars represent the standard error.
The results show that the severity index in seeds decreases as the treatment temperature increases under both methods. From the heat treatments evaluated in this study, the treatment with hot water at the three evaluated temperatures was the most effective in limiting the presence of Fusarium in naturally infected maize seeds. These results agree with those reported by Bennett and Colyer (2010) who found that infection in seeds decreased more rapidly with increases in the incubation temperature. A significant reduction in the incidence of infected seeds (10%) compared to non-treated seeds (50%) was obtained with hot water for 105 s. Similar results were also obtained by Rahman and Emran (2008) who report that the lowest incidence of F. moniliforme (4%) was found in seeds treated with hot water at 52°C for all the evaluated maize varieties. Coutinho et al. (2007) also report that treatments with hot water at 60°C can control F. verticillioides in maize seeds. In this study, as the time of heat treatment increased, the incidence of the fungus decreased especially for 10 min and 20 min of treatment.
Clear et al. (2002) found that treatments with dry heat for 15 d at 60°C, 5 d at 70°C and 2 d at 80°C eliminated F. graminearum completely from wheat seeds without a significant reduction of germination at 70°C and 80°C. Additionally, F. graminearum was completely eliminated in barley seeds only after 21 d at 60°C, 9 d at 70°C or 5 d at 80°C, showing a significant reduction of germination after 10 d at 80°C. These results show that to achieve complete control of some Fusarium species using dry heat treatments, it would be necessary to treat seeds for several days.
The observed decrease in the severity index of seeds in the hot water treatments could be due to the fact that the temperature acted against the contaminating fungi present in the seed (Rahman and Emran, 2008). Fungal death inside the seed can be generated due to thermal stress or desiccation (Clear et al., 2002). As the temperature increases, it manages to penetrate the seed more deeply eliminating the pathogens that have colonized its interior (Rahman and Emran, 2008).
Effect of thermal treatments of maize seeds on the variables of seedling growth and development.
The statistical analysis showed significant differences between the heat sources and the different temperatures evaluated, with interaction between these two factors (P<0.05) for the variables of seedling length (P=1.35e-11) and fresh weight (P=0.0435).
Seed germination percentages were significantly higher under dry heat treatments (71.7%) compared to hot water treatments (49.4%). Treatments at 60°C showed significantly lower values (41.6%) compared to those obtained at 50°C, 55°C and control (61.9%, 67.9% and 69.3% respectively) (Fig. 4A). The length of maize seedlings was significantly higher in dry heat treatments compared to hot water treatments, with 13.8 cm and 11.7 cm at 50°C, 14.1 cm and 12.1 cm at 55°C, and 13.4 cm and 7.0 cm at 60°C respectively (Fig. 4B). Regarding fresh weight, the highest values were obtained under dry heat treatments compared to hot water treatments with values of 110.4 g and 92.4 g at 50°C, 107.6 g and 93.1 g at 55°C, and 112.0 g and 86.7 g at 60°C, respectively (Fig. 4C). Fresh weight was significantly lower under hot water treatment at 60°C (Fig. 4C). Similar results were reported by Nega et al. (2003) and Rahman and Emran (2008), who underlined that treatments with hot water at 50°C for 20 min to 30 min and up to 53°C for 10 min may result in a significant decrease in infection of seed-borne pathogens without affecting seed germination. However, higher temperatures can generate a decrease in the percentage of germination of sensitive crops. Nega et al. (2003) reported that seedlings of treated seeds were smaller than seedlings of untreated seeds. This is, possibly, due to the loss of nutrients during the treatment, resulting in a delay in the normal development of the seedling that can affect the seedling length and fresh weight. Coutinho et al. (2007) reported that thermal treatments at 60°C can significantly reduce the germination rate of maize seeds by 30% when subjected to treatment for more than 5 min. These authors reported an alteration in the electrophoretic pattern of the enzymes esterase and malate dehydrogenase, demonstrating a loss of integrity of the membrane system such as the mitochondrial membrane, caused by exposing seeds to a high temperature. The reduction in germination percentages obtained in this study could be due to a loss of the integrity of the seed membrane system with the increase of temperatures. This allows a higher leaching of exudates, which include essential metabolites for the processes of germination and subsequent growth of seedlings (Coutinho et al., 2007).
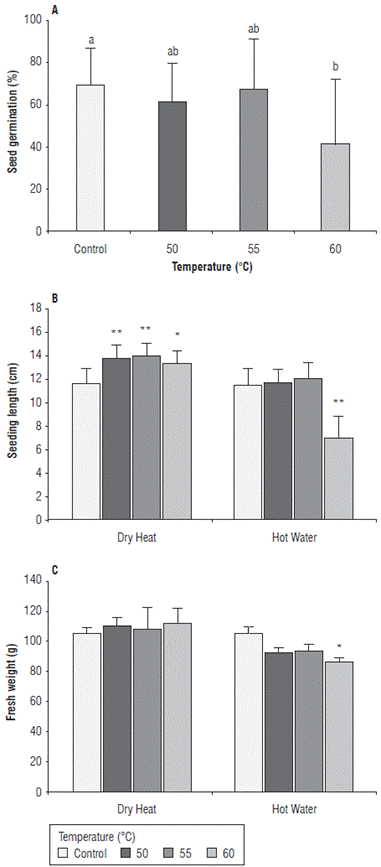
FIGURE 4 Effect of heat treatments applied by two methods, dry heat and hot water at temperatures 50°C, 55°C, and 60°C on A) germination percentage, B) seedling length, and C) fresh weight of maize seedlings of the regional variety Simijaca under laboratory conditions 10 days after treatment (dat). Significant differences between treatments and control are either denoted as * (P< 0.05) or ** (P<0.01). Bars represent the standard error.
In this study, treatments with hot water and dry heat at 55°C were the most effective in reducing the colonization of maize seeds by pathogens of the genus Fusarium without significantly affecting seedling germination and length. These results were based on the severity indexes obtained and the growth and developmental variables of maize seedlings. Dry heat treatments require higher temperatures and exposure times for generating a significant decrease in seed infection similar to that obtained with hot water (Fig. 3), hindering their implementation in large-scale commercial operations (Bennett and Colyer, 2010).
In vitro effect of fungicides on radial growth and germination of conidia of fungi of the genus Fusarium associated with stalk rot in maize
The inhibition of the radial growth of F. subglutinans and F. graminearum colonies showed an interaction between the different evaluated factors. The isolates 45D and 186A of F. subglutinans showed 100% inhibition of radial growth under prochloraz + difenoconazole and carboxin + captan at the three evaluated doses. Additionally, isolate 45D of F. subglutinans showed 100% inhibition with tebuconazole + trifloxystrobin at the three evaluated doses (Tab.1). The results coincide with that reported by Shin et al. (2014), who stated that the most effective fungicides against the mycelial growth of F. subglutinans were prochloraz and difenoconazole, which belong to the azoles group.
TABLE 1 In vitro effect of fungicides on the inhibition of radial growth (%) of F. subglutinans (45D and 186A) and F. graminearum (26B and 143A) colonies with commercial fungicides fludioxonil + metalaxyl-M, tebuconazole + trifloxystrobin, prochloraz + difenoconazole and carboxin + captan at doses 0.5, 1.0 and 1.5 CD (commercial dose). Mean values in the same column followed by different letters are significantly different according to the Tukey test (P<0.05).
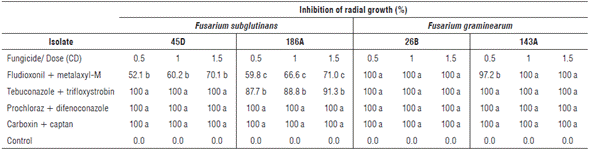
F. graminearum isolates 143A and 26B showed 100% inhibition of radial growth under the treatments prochloraz + difenoconazole, carboxin + captan, and tebuconazole + trifloxystrobin at the three evaluated doses. Complete inhibition of these two isolates was also observed with fludioxonil + metalaxyl-M at doses of 1.0 and 1.5 CD (Tab.1).
Prochloraz + difenoconazole, carboxin + captan and te-buconazole + trifloxystrobin at the three evaluated doses were also the most effective in inhibiting the radial growth of the evaluated isolates of F. graminearum. In contrast, a complete radial inhibition of this species was found with fludioxonil + metalaxyl-M at doses of 1.0 and 1.5 CD. The obtained results match the ones reported by Jones (2000), Broders et al. (2007), Ivić et al. (2011) and Shin et al. (2014).
Jones (2000) found that 1.0 mg L-1 of fludioxonil inhibits F. graminearum mycelial growth by 100%, as did tebuconazole at a dose of 100 mg L-1. Broders et al. (2007) reported that fludioxonil is the most effective fungicide in inhibiting F. graminearum mycelial growth with complete inhibition at 1.0 mg L-1 compared to azoxystrobin and trifloxystrobin (25 and 35%, respectively). Captan at a dose of 100 mg L-1 showed inhibition of up to 20% of F. graminearum mycelial growth, which is higher than treatments with strobirulins at the same dose. However, these last two active ingredients do not provide a complete inhibition of this species.
Ivić et al. (2011) reported that prochloraz is the most effective fungicide at controlling F. graminearum, F. avenaceum and F. verticillioides, inhibiting growth by 50% at a dose of 0.1 mg L-1, while flutirafol is the least effective even with doses higher than 8.51 mg L-1. Metconazole is more efficient compared to carbendazim and tebuconazole, inhibiting growth by 50% at a dose of 1.66 for F. graminearum. Car-bendazim inhibits fungal growth by 50% at a dose of 1.41 mg L-1., and tebuconazole at 2.57 mg L-1 for F. graminearum.
Shin et al. (2014) found that the fungicides of the azole group tebuconazole, difenoconazole, fluquinconazole and prochloraz are the most effective in inhibiting F. subglutinans mycelial growth at a dose of 10 µg ml1. These fungicides were more effective than strobilurins (kresoximmethyl and azoxystrobin) at the same dose, with 100% inhibition with azoles and 60% with strobilurins. At the same dose, the most effective fungicides to inhibit 100% of F. temperatum mycelial growth were tebuconazole and prochloraz.
The counting of conidia germinated in fungicide-amended media is one of the most widely used methods for evaluating fungicide efficacy (Matheron and Porchas, 2000; Shin et al., 2014). In this evaluation with the different isolates, the results showed interaction between the evaluated factors. F. subglutinans isolates 45D and 186A showed significantly higher values of conidial germination inhibition under fludioxonil + metalaxyl-M at the three evaluated doses (Tab. 2) compared to the dose 0.5 CD of prochloraz + difenoconazole and carboxin + captan in the two isolates, and 0.5 CD of tebuconazole + trifloxystrobin in isolate 45D. For F. graminearum isolate 143A, the inhibition values were significantly higher under fludioxonil + metalaxyl-M and tebuconazole + trifloxystrobin at the three evaluated doses compared to prochloraz + difenoconazole and carboxin + captan at the three evaluated doses. For F. graminearum isolate 26B, there were no significant differences between the evaluated treatments, showing 100% inhibition of co-nidial germination (Tab. 2).
TABLE 2 In vitro effect of fungicides on the inhibition of conidial germination (%) of F. subglutinans (45D and 186A) and F. graminearum (26B and 143A) with the active ingredients fludioxonil + metalaxyl-M, tebuconazole + trifloxystrobin, prochloraz + difenoconazole and carboxin + captan at doses 0.5, 1.0 and 1.5 CD (commercial dose). Mean values in the same column followed by different letters are significantly different according to the Tukey test (P<0.05).
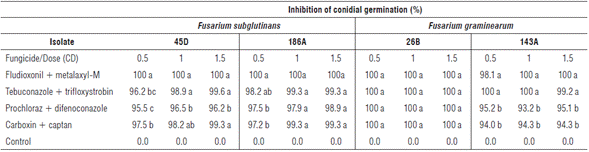
These results agree with the ones reported by Munkvold and O'mara (2002), who observed that fludioxonil and difenoconazole are significantly more effective in the control of F. subglutinans and F. graminearum species compared to fungicides such as captan. However, fludioxonil is significantly more effective than difenoconazole in inhibiting F. graminearum isolates. Shin et al. (2014) reported that the fungicide kresoxim-methyl (strobilurin) completely inhibits F. subglutinans and F. temperatum colony formation at concentrations of 0.1 and 0.01 ml-1 respectively. The four azole fungicides tested (difenoconazole, fluquin-conazole, prochloraz and tebuconazole) only completely inhibit the formation of F. subglutinans colonies at higher doses (1.0 ml-1). The inhibition of conidial germination with fungicides is essential for reaching chemical control of pathogenic fungi in plants since the spread of the disease and colonization of plants is mainly mediated by these reproductive structures of the pathogens (Shin et al., 2014).
The different responses to the same active ingredient between Fusarium species and even between isolates of the same species are phenotypic characteristics that vary between fungal populations (Müllenborn et al., 2008). Differences between isolates of the same species exposed to the same chemical compound could imply a decrease in the sensitivity to the active ingredient or resistance between the evaluated isolates (Ivić et al., 2011). In this study, fludioxonil, from the phenyl-pyroles group, caused greater inhibition of conidial germination of the species F. gramiearum and F. subglutinans possibly due to its action mechanism. This active ingredient alters MAP proteins in the transduction of osmotic signals causing inappropriate activation of the Hog1 MAPK, which is a protein involved in the reproduction of filamentous fungi (Degani, 2015).
Demethylation inhibitors (DMI fungicides), such as pro-chloraz, have become one of the most utilized fungicide groups in agriculture, being effective in controlling diseases in wheat and soybeans. They are also used in seed treatments, especially for the control of Fusarium spp. (Ivić et al., 2011). In this research, prochloraz at the three evaluated doses was also effective in controlling the assessed Fusarium species associated with maize seeds.
Although the results obtained are a contribution to the management of stalk rot in cold-climate maize producing areas, similar studies with a higher number of isolates of these pathogens are necessary. Additionally, in vivo studies to determine the effect of thermal seed treatments and research on fungicides at the commercial crop level are required. If the best treatments found in this study generate a lower yield loss due to stalk rot, they could be implemented in combination with cultural practices that may contribute to the sustainability of maize crops in areas affected by this problem.
Conclusion
Treatments with hot water (5 min) and dry heat at 55°C (10 min) were the most effective in controlling Fusarium spp. colonization of maize seeds of the regional variety Simijaca, without generating a significant reduction of the germination percentage, length and fresh weight of seedlings. The highest values of seed germination, length and fresh weight of maize seedlings were obtained under dry heat treatments. Commercial doses of the evaluated fungicides generated 100% inhibition of radial growth of F. graminearum isolates. Commercial doses of prochloraz + difenoconazole and carboxin + captan caused 100% inhibition of F. subglutinans isolates. Prochloraz + difenoconazole and carboxin + captan at the three evaluated doses were the most effective in inhibiting the radial growth of the isolates of the two evaluated Fusarium species, with no less than 90% effect on conidial germination inhibition. Fludioxonil + metalaxyl-M at the three evaluated doses generated a complete inhibition of conidial germination of all the assessed isolates. Based on the results of this study, active ingredients such as prochloraz + difenoconazole and carboxin + captan at commercial doses could be promising treatments of maize seeds of regional variety Simijaca for the control of pathogens of the genus Fusarium associated with stalk rot.