Introduction
From a nutritional point of view, maize (Zea mays L.) is one of the most important cereals in the world. Maize constitutes the nutritional basis for humans in many developing countries, and it is also the energy concentrate par excellence of intensive poultry and livestock feeding systems (Permuy, 2013). In Cuba, maize grain production has been affected by economic problems and by the use of inadequate soil practices and crop management that sometimes leads to significant environmental degradation.
Obtaining acceptable crop yields requires the application of large quantities of mostly nitrogen fertilizers. The use of chemical compounds as a means of fertilization in agriculture is a common practice among farmers. However, its indiscriminate use significantly alters the balance of the organic and living soil constituents (Vidal et al., 2015). In agricultural production systems based on crop rotation, maize is intercropped with some leguminous crops. This technique allows the maize to take advantage of the benefits provided by microbial communities that are associated with the leguminous rhizosphere. This nutrient contribution is one of the main benefits helping to decrease the application of the mineral fertilizer load (Martín and Rivera, 2015).
Biofertilizers based on microorganisms that promote plant growth are considered a component of integrated plant nutritional management (Vessey, 2003) and allow substituting part of the inorganic fertilizers in many crops. The success in the use of these biopreparations lies (besides other aspects) in obtaining strains that are compatible with the crop of interest (Hernández et al., 2004) and that are efficient for obtaining the desired yields.
The rhizobia constitute a group of diazotrophic microorganisms that have been mainly studied for their symbiotic association with leguminous plant roots and on which differentiated organs called nodules are produced. In these structures, these bacteria perform biological nitrogen fixation (BNF), mediated by nitrogenase protein complex activity (Luyten and Vanderleyden, 2000). Currently, rhizobia are considered plant growth promoting rhizobacteria (PGPR) because they have the ability to promote non-leguminous plant growth at the rhizosphere level or as endophytes without nodule formation (Sessitsch et al., 2002). Several studies demonstrate these microorganisms' capacity to colonize non-leguminous plant rhizospheres such as rice, lettuce, tomato, pepper, wheat and maize (Bécquer et al., 2011; García-Fraile et al., 2012; Flores-Félix et al., 2013; Hernández, 2015). However, rhizobia used in these trials were isolated from legume nodules and not from the crop rhizosphere itself.
In Cuba, the presence of rhizobia associated with maize plants has not been reported; and, therefore, there are no biopreparations based on these microorganisms for crop biofertilization. Taking into account this background, the aim of this study was to identify maize rhizospheric rhizobia.
Materials and methods
Studies were carried out in the Department of Plant Physiology and Biochemistry at the Instituto Nacional de Ciencias Agrícolas (INCA) in Cuba and at the Centro de Investigaciones Biotecnologicas del Ecuador (CIBE), Escuela Superior Politecnica del Litoral (ESPOL).
Sampling
Two maize cultivars were sampled: Raúl and Canilla. They were cultivated in a Ferralitic Red Leached soil (Hernández et al., 2015) at "El Mulato" farm and at the Centro Nacional de Sanidad Agropecuaria (CENSA), respectively. Both centers are located at San Jose de las Lajas, Mayabeque province, Cuba. The bean (Phaseolus vulgaris L.) was the rotation crop. At both sites five random sampling points were established and two plants were taken from each of them for a total of10 plants per cultivar. The extraction was performed taking a volume of soil of20x20x20 cm. Samples were placed separately in polyethylene bags and stored in the laboratory at 4°C until they were used.
Rhizobia isolation from Raúl and Canilla maize cultivar rhizospheres
Rhizobia isolation was conducted from rhizospheric soil and the maize rhizoplane using the methodology of Knief et al. (2012). For rhizospheric soil isolation, 1 g samples of soil were taken and serial dilutions were performed (10-110-6). One hundred suspensions were grown in YMA (Yeast Mannitol Agar) medium at pH 6.8 with congo red. Plates were incubated at 28°C for 10 d.
For rhizoplane isolation, roots were cut into 1 cm long portions and placed in Erlenmeyer flasks with 10 ml of sterile distilled water. Flasks were maintained while stirring for 1 h at 150 rpm. The same methodology was used later with dilutions from rhizospheric soils.
Roots from the previous methodology were placed on plates with LB (Luria Bertani agar) medium and incubated at 28°C for 24 h. Subsequently, visible bacterial growth around the roots was collected and cultivated in YMA medium at pH 6.8 with Congo red. Plates were incubated at 28°C for 10 d. Strains obtained were stored at -20°C in the INCA bacterial culture collection.
Cultural and morphological characterization
The color, size (mm) and mucus of the colonies were determined as proposed for rhizobia (Wang and Martínez-Romero, 2001). Colonies with whitish coloration, semi-translucent, mucous or dry, and all those that absorbed YMA medium red pigment at its center were selected at different culture stages. Cultures that grew over a period of 1- 3 d were considered as fast growth, and those up to 10 d as slow growth. Colonies with diameters between 1-2 mm and 2- 4 mm were considered small or large, respectively. These were purified by successive passes and kept at 4°C in tubes with YMA medium. Cell morphology and the presence of spores were determined by gram staining.
Biochemical characterization
Eight biochemical tests were applied to each isolate: acid-base production, citrate utilization, oxidase, catalase, urease, hydrogen sulfide production (H2S), gelatinase, and an oxidation-fermentation test.
To determine acid-base production isolates were cultured in YMA medium at pH 6.8 with bromothymol blue indicator (0.5% in sodium hydroxide (NaOH) 0.016 N). Subsequently, plates were incubated at 28°C for 10 d. Cultures that changed medium coloration from green to yellow were taken as positive results for acid production. Base production was found in the isolates that changed medium coloration from green to blue.
The use of citrate as carbon and energy sources was determined in tubes with Simmons citrate agar medium (Koser, 1923). Tubes were incubated at 28°C for 10 d. A change in medium coloration from green to yellow was interpreted as a positive result.
Determinations of oxidase and catalase activity were performed by the methods of Kovaks (1956) and Graham-Parker (1964), respectively.
To determine urease enzyme production, Christensen's urea agar medium (Christensen, 1946) was used. Tubes were incubated at 28°C for 10 d. A change in medium coloration from yellow to pink was considered positive urease and negative urease was considered when the medium remained yellow.
Hydrogen sulfide production (H2S) was determined in TSI agar medium (Triple Sugar Iron) (MacFaddin, 2000). Tubes were incubated at 28°C for 10 d. The presence of a black precipitate at the bottom of the tube was interpreted as a positive result.
The gelatinase activity test was performed according to Leboffe and Pierce (2010) in nutrient gelatin medium (Difco Laboratories, 2009). Tubes were incubated at 28°C for 48 h. Those isolates that hydrolyzed the medium were taken as positive gelatinase and the others that maintained the medium in the solid state were considered as a negative gelatinase.
The type of energy metabolism (respiratory or fermentative) was performed following Hugh-Leifson's method (Hugh and Leifson, 1953) in O-F basal medium (Winn et al., 2006). Two tubes were used per isolate, one under anaerobic and the other one under aerobic conditions. Tubes were incubated at 28°C for 48 h. Isolates that totally changed medium coloration from green to yellow in both tubes were considered positive for anaerobic metabolism. Those that remained green in the anaerobic tube and then changed partially or totally to yellow in the other tube were considered positive for oxidative metabolism.
DNA extraction
Extraction of the genetic material was performed by alkaline lysis (von Post et al., 2003). A colony of the axenic culture was taken and placed in an Eppendorf tube with 40 of NaOH at 0.20 M. It was then heated in a microwave at 10% power or 700 W for 1 min.
Nucleic acid quantification by spectrophotometry was performed using the NanoDropTM 2000 at 260 nm; the ratios 260/280 and 260/230 were calculated in order to determine DNA concentration and quality.
PCR amplification
Universal primers forward 27F (5'-AGAGTTTGATCCT-GGCTCAG-3') and reverse 1492R (5'-GGTTACCTT-GTTACGACTT-3') (Criollo et al, 2012) were used for 16S rDNA amplification. This procedure was carried out in a mini MS thermal cycler (Major Science, USA) under the following amplification parameters: initial denaturation 95°C for 10 min, additional denaturation for 1 min at 92°C and 35 cycles of 72°C each for 2 min. Amplification results were verified by electrophoresis in 1% agarose gel. PCR product sequencing was carried out by the Sanger method of Macrogen® (Republic of Korea).
Editing sequences and identification
Sequence quality analysis was performed through the FinchTV program (version 1.4.0, Geospiza Inc., Seattle, WA, USA). Consensus sequences were obtained with the MEGA-X software (version 10.0.4, Molecular Evolutionary Genetics Analysis) (Kumar et al., 2018) and compared with the NCBI (National Center for Biotechnological Information) database, using the BLASTn (Basic Local Alignment Search Tool - nucleotide, Maryland, USA) program (Benson et al., 2015). Alignments with an e-value lower than 1x10-5 and with a similarity and coverage greater than 80% of amplified region were considered as a hit.
Results and discussion
Rhizobia isolation from the maize cultivars Raúl and Canilla rhizosphere
A total of 81 isolates were obtained consisting of 46 strains of the Raúl cultivar and 35 strains of the Canilla cultivar. Forty-seven came from the rhizoplane equaling 58.3% and the rest came from rhizospheric soil. The rhizoplane is the rhizosphere zone most influenced by radical exudates and where their concentration is at a maximum (Atlas and Bartha, 2005). This is an attractive area for microbial colonization (Kapulnik, 2002). In addition, radical exudates from grasses provoke chemotactic responses in rhizobac-teria such as rhizobia (van Rossum et al., 1995). Vanillic, p-coumaric, m-coumaric and cinnamic acids excreted by rice plants (and others) are important carbon sources for rhizobia and allow their saprophytic survival in this crop rhizosphere (Heidarzade et al, 2010).
Cultural and morphological characterization
The 81 isolates complied with cultural descriptions proposed for rhizobia (Wang and Martínez-Romero, 2001). Taking into account the large number of isolates with similar morpho-cultural characteristics to those described for the rhizobia group, gram stain was used as a second discrimination criterion. Cellular morphology and staining responses allowed separating isolates into seven groups (Tab. 1).
TABLE 1 Bacterial groups established according to their morphological and staining characteristics.
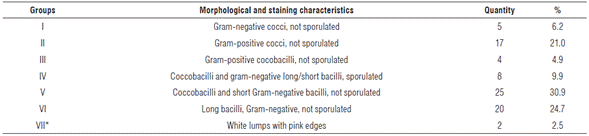
*There was no response to gram staining. Probably not bacteria.
Group number V was the most representative with a 30.9% of total bacteria detected. Coincidentally, morphological and staining isolate characteristics included in this group agree with those described by Frioni (1990) for rhizobia. In addition, these isolates showed a rapid growth rate (24 h) under used conditions. Rhizobia usually show fast growth rates with the exception of the genus Bradyrhizobium, which can take 7 to 10 d to grow (Berrada and Fikri-Benbrahim, 2014).
Biochemical characterization
Acid production in YMA medium with bromothymol blue was determined in 24 isolates, and only one showed base production (results not shown). Acid excretion is a characteristic shared by some rhizobia genera with fast growth rates, such as Rhizobium, Allorhizobium and Sinorhizobium (Berrada and Fikri-Benbrahim, 2014). One of the organic acids produced by rhizobia is indoleacetic acid (IAA), which promotes plant growth, since it allows cellular elongation, root initiation, and the formation of root hairs (van Loon, 2007).
Phosphate solubilization in soils constitutes another important function of these acids, which increase nutrient availability, an aspect that is advantageous for the plant (Zúñiga et al., 2013). Regarding base production, although less frequent, it has taxonomic value in slow-growing rhizobia identification, such as the genus Bradyrhizobium. In addition, this phenomenon intervenes in other mineral solubilization (Sugumaran and Janarthanam, 2007).
Eight isolates grew and produced a change of color in Simmons medium (Tab. 2), which indicates the capacity to metabolize sodium citrate (MacFaddin, 2000). Generally, rhizobia respond negatively to this assay (Sadowsky et al., 1983); this could be due to citrate molecule complexity in terms of its functional groups volume (three carboxyl groups), compared to other carbon sources used by these microorganisms that basically include sugars, alcohols and organic acids (Gaurav et al., 2016). The YMA medium includes mannitol, sucrose or glycerol as the sole carbon sources. These guarantee rhizobia multiplication with a suitable nitrogen source and under certain parameters of pH and temperature (Hernández and Nápoles, 2018).
TABLE 2 Isolate rhizosphere biochemical characterization of the maize cultivars Raúl and Canilla.
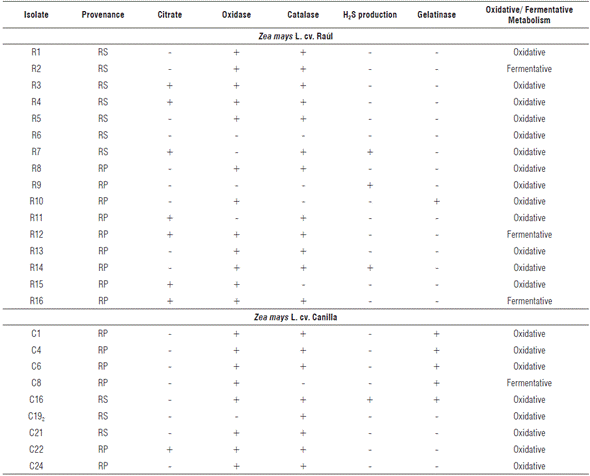
RP: rhizoplane, RS: rhizospheric soil.
Rhizobia are: citrate negative (-), oxidase positive (+), catalase positive (+), no H2S production (-), gelatinase positive (+), urease positive (+), and have oxidative metabolism (usually).
Cytochrome oxidase enzyme activity was observed in 20 isolates and the same number for catalase activity (Tab. 2). Similar results were obtained by Sadowsky et al. (1983), which show that both slow-growing and fast-growing rhizobia share the positive results of these trials. However, urease enzyme production that is positive for rhizobia (Sadowsky et al., 1983), is negative in all isolates (results not shown).
Hydrogen sulfide precipitation was seen in four isolates. Rhizobia lack the capacity to reduce the sodium thiosulfate present in TSI medium, so there is no production of H2S. Gelatinase activity was seen in six cases (Tab. 2), which could correspond to some types of rhizobia, since this is a biochemical characteristic of the group (Sadowsky et al., 1983).
The ability to metabolize glucose by fermentation was seen in four isolates that produced a change in medium coloration produced by organic acid excretion (Tab. 2). Oxidative metabolism was much superior to the previous variant with 21 isolates grown in aerobic conditions. Some gram-negative bacteria metabolize glucose through aerobic respiration; and, as a result, small amounts of weak acids are formed during Krebs cycle and the Entner Doudoroff pathway (Winn et al., 2006) that promote medium color changes, from green to yellow. In addition, this test allowed the determination of motility, since acid production is low, color changes are only observed in the tube's upper levels. In this case, total medium discoloration to yellow was observed in 100% isolates, which shows bacterial displacement throughout the tube.
Identification by 16S rDNA gene sequencing
In biochemical tests performed on rhizospheric isolates, null urease enzyme activity was determined that contradicts the biochemical pattern usually present in rhizobia. In this way, it could be concluded that none of the isolates belong to this group of microorganisms. However, molecular biology confirmed these results (Tab. 3).
Eight bacterial genera were identified from twenty-five isolates; five corresponded to the genus Rhizobium for 20% (Tab. 3.).
The most representative genera were Pseudomonas and Stenotrophomonas with 32 and 28%, respectively. The genera Enterobacter, Starkeya, Achromobacter, Delftia and Flavobacterium were identified, each one with 4% representation.
Most of these genera have been described as part of various crops' rhizospheric microbiota, including maize. These include mainly Pseudomonas, Stenotrophomonas and Enterobacter (Hernández et al., 2004; Morales-García et al., 2011; Granada et al., 2015; Yang et al., 2017; Gaviria-Giraldo et al, 2018).
The capacity of Rhizobium for rhizospheric and endophytic colonization of horticultural crops (García-Fraile et al., 2012; Flores-Félix et al., 2013) and grasses (Chabot et al., 1996; Gutiérrez-Zamora and Martínez-Romero, 2001; Sessitsch et al., 2002) has been studied. However, microorganisms that were used in these studies were isolated from legume nodules and not from the crop's rhizosphere.
Isolates obtained in this study come from Red Ferralitic soils, which have a low percentage of organic matter, and this translates into poor nitrogen content (Hernández et al., 2015). Maize requires high doses of nitrogen for growth and development, so it is common to rotate it with leguminous crops that enrich soil nitrogen concentration (Martín and Rivera, 2015). The presence of beans (Phaseolus vulgaris L.) as a predecessor crop to maize from which the isolation was performed and the type of soil characteristics constitute two important factors that explain the rhizobia population presence associated with this plant rhizosphere. Bean establishes a greater symbiotic interaction with Rhizobiaceae family representatives, fundamentally with the genus Rhizobium (Ramírez-Bahena et al., 2008; Dall'Agnol et al., 2013), an aspect that could explain the presence of this genus in the crop's rhizosphere. In this way, Rhizobium can be demonstrated as a common component of maize rhizospheric microbiota.
Conclusions
In the maize rhizosphere, planted in Red Ferralitic soil in Mayabeque province, Cuba, there are rhizobia populations of the genus Rhizobium spp. Biochemical characterizations are important elements for microorganism identification. However, with the development of molecular techniques, a more accurate and reliable taxonomic diagnosis can be made.