Introduction
Italian zucchini (Cucúrbita pepo L. - Cucurbitaceae) is a vegetable-like fruit with an erect bushy growing habit, despite having an herbaceous stem. It is one of the most popular and appreciated vegetables in Brazilian cuisine (Nakada-Freitas, 2014; Azambuja et al., 2015). Zucchini is a summer squash that develops in autumn and spring, and also during the winter in places with a warmer climate (Filgueira, 2012).
The effects of nutrition and fertilization management practices on growth, development, and yield of zucchini, as well as other crops, has been one of the most studied agricultural aspects (Domingos et al., 2015). Nitrogen (N), which is responsible for several functions in plants and is directly linked to crop yield, is the main topic for most researchers (Oliveira et al., 2014).
Nitrogen is the second most required nutrient by plants (Viciedo et al., 2017). It is an essential macro-element that participates in the synthesis of amino acids, nucleic acids, secondary metabolites, and components of the chlorophyll molecule. In addition, this nutrient stimulates the photo-synthetic capacity of leaves by increasing the content of stromal and thylakoid proteins in chloroplasts (Akram et al., 2011). However, over-fertilization with nitrogen has negative effects, such as disordered plant growth, susceptibility to pathogens and insects, nutrient deficiency due to competition with other nutrients, auto shading, and increased production costs (Porto et al., 2012; Xu et al., 2012).
Rational nitrogen fertilization practices are of great importance, being essential the search for methods that boost the beneficial effects of this nutrient. Therefore, nitrogen application combined with silicon (Si) may provide higher yields (Artigiani et al., 2014), making it a viable alternative. Adequate Si supply may induce growth, resistance to biotic and abiotic stresses, and increase the photosynthetic capacity (Ferraz et al., 2014). In addition, Si application provides a better utilization of some nutrients by plants (Watanabe et al., 2001).
Chlorophyll a fluorescence can be a good indicator of the plant's physiological state. The absorbed light energy excites the chlorophyll molecule and the disposing of its available energy occurs through three alternative pathways which compete for the excitation energy (photochemical dissipation, fluorescence or non-photochemical dissipation). Thus, changes in one of these processes, as in photosynthesis for example, shall affect the other ones (Cendrero-Mateo et al., 2016).
Plants spend about 25% of their total energy on NO3 - assimilation (Rubio-Asensio et al., 2015). In C3 plants, this process would be partly coupled with photorespiration, which stimulates the transport of chloroplasts malate to the cytosol for Nicotinamide Adenine Dinucleotide Hydride (NADH) formation (Bloom, 2015). NADH is used by nitrate reductase to reduce NO3 - to NO2 -, NO3 - assimilation process and would be related in part to photorespiration. In this regard, Si accumulated near the stomata reduces the rate of transpiration (Oliveira and Castro, 2002), providing a direct relationship between transpiration and chlorophyll fluorescence and Si and N, making the photosynthesis process more efficient.
Chlorophyll fluorescence parameters are widely used in physiological studies since they are obtained by a non-destructive method that allows the qualitative and quantitative analysis of the absorption and use of light energy by the photosynthetic apparatus (Oliosi et al., 2017). Therefore, the aim of this study was to evaluate the interaction between nitrogen and silicon fertilization on growth, chlorophyll index and chlorophyll a fluorescence of zucchini plants.
Materials and methods
The experiment was carried out under field conditions in the agroecology sector of the Agrarian and Exact Sciences Department (DAE) of the State University of Paraiba (UEPB), Campus IV, in the municipality of Catole do Rocha, Paraiba, Brazil (6°20'38" S, 37°44'48" W, 275 m a.s.l.).
Zucchini seeds cv. Caserta were directly sown in planting pits, spaced at 1 x 1 m. After digging the pits of 30 x 30 x 30 cm dimension, 2 L of tanned cattle manure were added (Filgueira et al., 2012).
The soil of the experimental area was classified as eutrophic Fluvic Neosol, with a sandy loam texture (Embrapa, 2013). Before the installation of the experiment, soil samples were collected at a depth of 0-20 cm for physical and fertility analysis (Tab. 1) and the cattle manure chemical analysis was also performed (Tab. 2), according to the methodology proposed by Embrapa (2013). The soil was prepared by plowing sequenced by harrowing.
TABLE 1 Chemical and physical characteristics of the Eutrophic Fluvic Neosol used in the experiment.
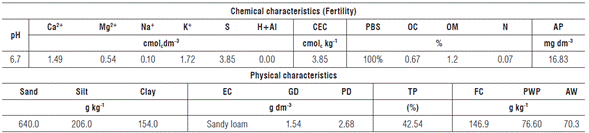
CEC: Cation exchange capacity; PBS: Percent base saturation; OC: Organic carbon; OM: Organic matter; AP: Assimilable phosphorus ; EC: Exchangeable cations; GD: Global density; PD: Particle density; TP: Total porosity; FC: Field capacity; PWP: Permanent wilting point; AW: Available water.
TABLE 2 Chemical characteristics of the cattle manure used in Catole do Rocha, 2019.

SOM: Soil organic matter; OC: Organic carbon; C/N: carbon-to-nitrogen ratio.
The treatments were distributed in a split-plot scheme in a randomized complete block design, with three replicates, and one plant per replicate. The plot was arranged by silicon levels (0 and 6 g/plant) and the subplots were five nitrogen levels (30, 60, 90, 120, and 150 kg ha-1). The nitrogen source used was urea (45% N). The silicon levels were based on an estimate of 60 kg ha-1. The product Bugram Protect®, which contains 100% pure silicon dioxide, pH 6.8 and 0.9% moisture, was used as a silicon source. The N doses were equally divided and applied to cover the soil twice, first at 15 days after planting (DAP) and then at 30 DAP. Foliar fertilization with Si was also divided into two applications, the first at 14 DAP and the second at 28 DAP. Thus, 540 g of silicon dioxide was diluted in 30 L of water in the first application and 540 g was diluted in 40 L of water in the second application. The silicon solutions were uniformly applied on the plants, using a 10 L capacity backpack sprayer, totaling 1.080 kg of silicon dioxide.
Micro-irrigation was performed through a drip irrigation system, spaced at 0.2 m and with a flow rate of 1.7 L per hour, at a service pressure of 147 KPa, using 16 mm drip tapes. The water was supplied through an Amazon well near the experiment site, and it was classified as moderately saline (1.2 dS m-1) (Ayers and Westcot, 1999).
The chlorophyll a fluorescence trials were performed at 35 DAP using a modulated fluorometer (Model OS-30p, Hudson, USA). Foliar tweezers were placed for 30 min before the readings to adapt the leaves to the dark. The initial fluorescence (F0), maximum fluorescence (Fm), variable fluorescence (Fv = Fm-F0) and quantum yield of photosystem II (Fv/Fm) were evaluated. Chlorophyll a, b and total contents were estimated by the non-destructive method using a portable chlorophyll meter (ClorofiLOG®, model CFL 1030, Porto Alegre, Brazil), and expressed as Falker Chlorophyll Index (FCI). For leaf, stem and total dry mass estimation, each part of the plant was packed in Kraft paper bags and dried in a forced-air circulation oven at 65°C until reaching constant weight. Subsequently, the material was weighed in a precision analytical balance (0.001 g), and the results expressed in g/plant.
The data were submitted to an analysis of variance by the F test at 5% probability. Polynomial regression analysis for the nitrogen factor and the Tukey test for the silicon factor were performed in case of significance. The statistical program R was used (R Core Team, 2018).
Results and discussion
Leaf dry mass (LDM), stem dry mass (SDM) and total dry mass (TDM) of zucchini were significant at 1% of probability in the interaction between the studied factors, nitrogen (N) and silicon (Si) (Tabs. 3 and 4).
TABLE 3 Summary of the analysis of variance by the mean-square values, from leaf dry mass (LDM), stem dry mass (SDM) and total dry mass (TDM) of zucchini (Cucúrbita pepo L.) under nitrogen doses and silicon leaf fertilization in Catole do Rocha, 2019.
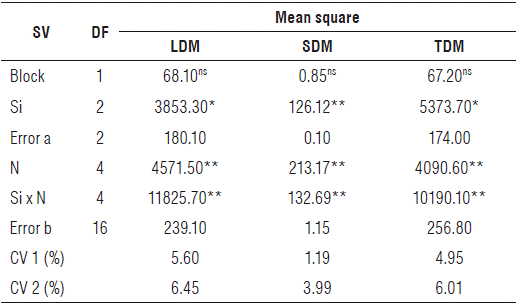
SV: Source of variation; DF: Degrees of freedom; CV: Coefficient of variation; ns: Not significant; ** and *: Significant effect by the F test at the level of 1 and 5% probability, respectively.
TABLE 4 Mean values for leaf dry mass (LDM), stem dry mass (SDM) and total dry mass (TDM) of zucchini (Cucúrbita pepo L.) under nitrogen doses and silicon leaf fertilization in Catole do Rocha, 2019.
Parameter | Silicon | Nitrogen (kg ha-1) | ||||
---|---|---|---|---|---|---|
(g/plant) | 30 | 60 | 90 | 120 | 150 | |
LDM | 0 6 | 242 a 185 b | 250 b 277 a | 191 b 257 a | 217 a 227 a | 355 a 195 b |
SDM | 0 6 | 26 a 21 b | 31 a 26 b | 46 a 27 b | 25 b 27 a | 18 b 23 a |
TDM | 0 6 | 268 a 206 b | 280 a 303 a | 236 b 283 a | 241 a 254 a | 372 a 217 b |
Means followed by equal letters in the same column do not differ statistically from each other by the Tukey test at 5% probability.
For LDM, an inverse behavior was observed in the treatments with and without Si, respectively. The highest increase in LDM was found in the treatments without Si and at the maximum N dose (150 kg ha-1), which corresponds to 338.6 g/plant. However, the LDM in the presence of Si reached its best value in the order of 265.4 g/plant, at the estimated dose of 87 kg ha-1 (Fig. 1A).
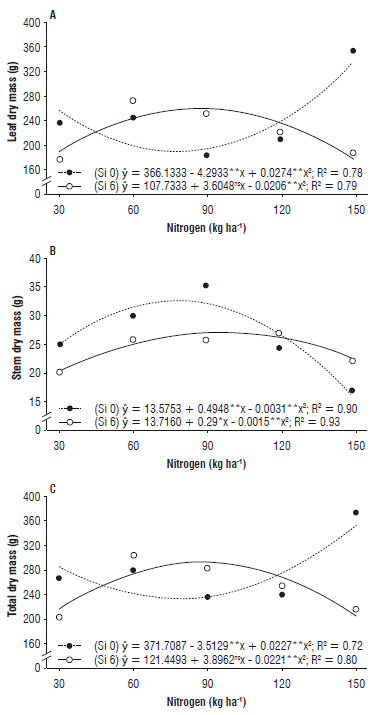
FIGURE 1 (A) Leaf dry mass, (B) stem dry mass, and (C) total dry mass of zucchini (Cucurbita pepo L.) under nitrogen doses and silicon leaf fertilization.
The values for SDM adjusted to a quadratic effect, both in the presence and absence of Si. The highest increase was registered at the N dose of 79.8 kg ha-1 in the treatments without Si, which corresponds to a 21.5% increase when compared to treatments under Si application, with values in the order of 33.3 and 27.4 g/plant, respectively (Fig. 1B).
For TDM, the results were similar to those of LDM. The best performance was obtained at the N dose of 150 kg ha-1 in the treatments without Si, corresponding to 355.5 g/plant, which may be related to the maximum increase observed in LDM (Fig. 1C).
The positive values obtained from nitrogen fertilization and the different morphological parameters may be due to the fact that this nutrient provides increases in the rate of carbohydrates conversion into protein and the size of the cells. This may lead to greater leaf expansion and higher growth rates, which directly influences the production of dry matter. Yasari et al. (2009) and Rodrigues et al. (2014) also registered positive results for dry mass production with increased N supply in paracress plants (Acmella olerácea L.), in which a linear increase was verified, with the highest efficiency registered at 112.5 kg ha-1 of N.
The decrease in dry mass of silicon-applied plants may be associated with the fact that silicon deposited on shoots is condensed by transpiration and subsequently transformed into amorphous silicon located in the cell wall of epidermal and vascular tissues, causing reduced water loss (Alsaeedi et al., 2019).
According to the results of the ANOVA, it was observed that there was a significant effect of the nitrogen doses on the chlorophyll contents and chlorophyll a fluorescence parameters. For the silicon factor, it was observed that there was a significant difference only for the fluorescence variables, except for the variable fluorescence (Fv). However, the interaction between the factors significantly affected all variables analyzed (Tab. 5).
TABLE 5 Summary of the analysis of variance by the mean-square values, for chlorophyll a (Cla), chlorophyll b (Clb), total chlorophyll (tCl), chlorophyll a/b ratio (Cla/Clb), initial fluorescence (F0), maximum fluorescence (Fm), variable fluorescence (Fv), and quantum yield of photosystem II (Fv/ Fm) of zucchini (Cucúrbita pepo L.) under nitrogen doses and silicon leaf fertilization in Catole do Rocha, 2019.
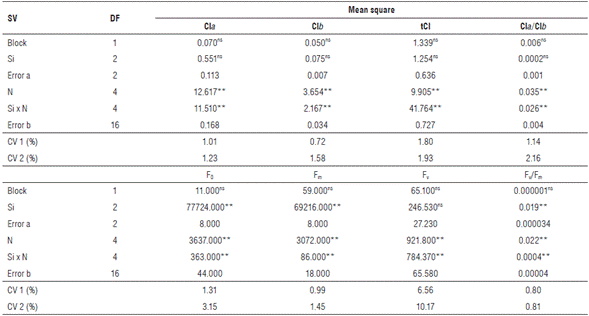
SV: Source of variation; DF: Degrees of freedom; CV: Coefficient of variation; ns: Not significant; ** and *: Significant effect by the F test at the level of 1 and 5% probability, respectively.
TABLE 6 Mean values for chlorophyll a (Cla), chlorophyll b (Clb), total chlorophyll (tCl), chlorophyll a/b ratio, initial fluorescence (F0), maximum fluorescence (Fm), variable fluorescence (Fv), and quantum yield of photosystem II (Fv/Fm) of zucchini (Cucurbita pepo L.) under nitrogen doses and silicon leaf fertilization in Catole do Rocha, 2019.
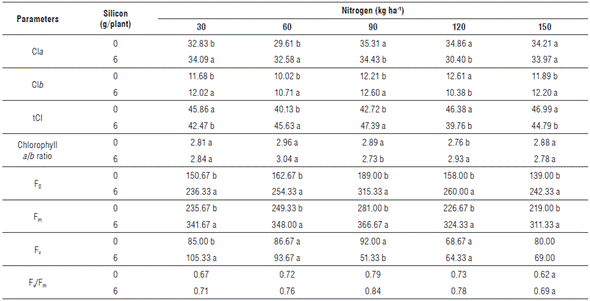
Means followed by equal letters in the same column and line do not differ statistically from each other by the Tukey test at 5% probability.
Chl a and Chl b values presented a quadratic effect both in the treatments with and without Si, with the highest index found at 146.1 kg ha-1 of N without Si application, evidencing a 5.6% increase compared to the treatment with Si. This behavior in the Si-containing treatments demonstrates FCI values for Chl a and Chl b in the order of 35.4 and 33.4, respectively (Fig. 2A).
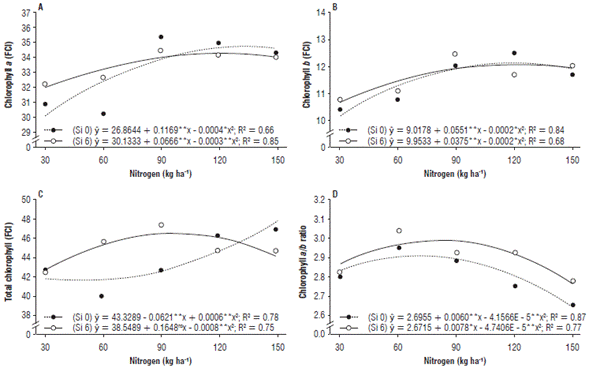
FIGURE 2 (A) Chlorophyll a, (B) chlorophyll b, (C) total chlorophyll and (D) chlorophyll a/b ratio of zucchini (Cucurbita pepo L.) under nitrogen doses and silicon leaf fertilization.
For Chl b, a similar behavior was observed, with the highest increase in the treatments without Si and under the N dose of 137.7 kg ha-1, with an FCI of 12.8. Under Si application, the N dose that provided the highest efficiency was 93.8 kg ha-1 (Fig. 2B). Since N is one of the main components of chlorophyll molecules, an adequate supply of this nutrient maximizes the indices of this photosynthetic pigment (Wang et al, 2014).
The total chlorophyll index increased linearly from the N dose of 51.7 kg ha-1, in the treatments without Si application. Under Si fertilization, the best performance of plants for this variable was found at 103.0 kg ha-1 of N, with a 46.8 FCI (Fig. 2C). However, it can be observed that, for the chlorophyll a/b ratio, the Si-containing treatments presented the best results, with the greatest increase at 82.3 kg ha-1 of N (Fig. 2D).
Corroborating this statement, Ávila et al. (2010) report a leaf chlorophyll synthesis increase in silicon-fertilized rice plants (Oryza sativa L.), especially at high doses of nitrogen. Contrary to this, Rodrigues et al. (2016) state that increased potassium silicate doses provide increases in chlorophyll a levels in tomato plants (Solanum lycopersicum L.) under high and low light conditions. However, the increase in chlorophyll a/b ratio may be associated with the greater synthesis of chlorophyll a, which is an indication that the plant was not experiencing stress.
It was observed that F0 and Fm showed a similar behavior, adjusting to a quadratic effect, and the best results were obtained in the treatments under Si fertilization, with values in the order of 288.5 and 355.2 /quantum at the N doses of 91.9 and 72.3 kg ha-1, respectively (Figs. 3A and B). This increase in F0 indicates that in the aforementioned N dose there was a reduction in the capacity of excitation energy transfer to the photosystem II (SÁ et al., 2015). Fm refers to the state in which the reaction centers of photosystem II reach their maximum capacity (Silva et al., 2014). Silicon accumulated near the stomata reduces leaf transpiration rates (Oliveira and Castro, 2002), a fact that may have benefited nitrate reductase in the photorespiration process, having increased chlorophyll indices in chloroplasts (Bloom, 2015).
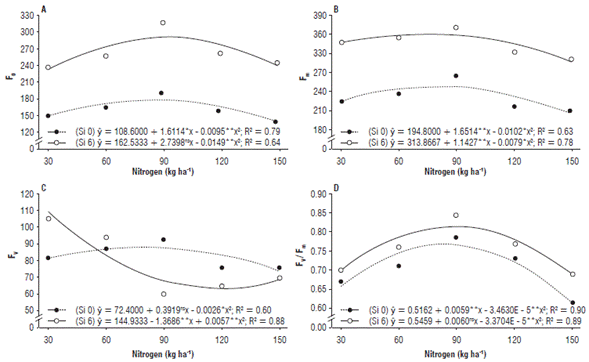
FIGURE 3 (A) Initial fluorescence (F0), (B) maximum fluorescence (Fm), (C) variable fluorescence (Fv), and (D) quantum yield of photosystem II (Fv/ Fm) of zucchini (Cucurbita pepo L.) under nitrogen doses and silicon leaf fertilization.
The Fv in the treatments with Si showed the best result at the N dose of 30 kg ha-1, with a value of 109.0 quantum electrons (Fig. 3C). For the Fv/Fm variable, the N dose of 89.0 kg ha-1 under Si application provided the highest value (0.81 quantum electrons), a 6.2% increase compared to treatments without Si (76 quantum electrons) (Fig. 3D).
Variable fluorescence refers to active potential energy in photosystem II, which is directly influenced by the maximum fluorescence (Sá et al., 2018). According to these same authors, these increases in fluorescence may be related to the higher content of chlorophyll in the reaction centers as a function of the N doses. Based on the Fv/Fm results, it can be stated that the N combined with Si induced a better energy stability compared to the treatments that did not contain Si (0.81), which is within those indicated as normal (between 0.75 and 0.85) in non-stressed plants (Neves et al., 2019).
Conclusions
The highest dry matter productions in zucchini plants were obtained in treatments without Si. The application of Si and N together positively influences the chlorophyll a/b ratio of zucchini plants. The interaction between Si and N positively influences the maximum fluorescence, variable fluorescence and quantum yield of photosystem II of zucchini plants.