Introduction
The tropics have thermal uniformity, without marked temperature seasons. A reduction in temperature with increasing altitudes means that these zones have altitudinal thermal "floors" (Fischer & Orduz-Rodríguez, 2012). Since plants can grow satisfactorily only in certain temperature ranges (Das, 2012), they can only grow in a certain altitude range where the main component is temperature.
Under the colder conditions of the Andean highlands, fruit development is prolonged, and the time to harvest is increased (Mayorga et al., 2020), which is why larger and better quality fruits can be generated, as compared to lower altitudes, as seen in feijoa (Parra-Coronado et al., 2015, 2016, 2017a), banana passion fruit (Mayorga et al., 2020), and apples (Fischer et al., 2016; Gutiérrez-Villamil et al., 2022). In the tropics, each fruit crop has its optimal altitude range for meeting ecophysiological demands (Fischer & Orduz-Rodríguez, 2012).
The phytochemical compounds of fruits are considerably affected by climatic site conditions and the interaction between environmental conditions and varieties (Zeng et al., 2020). The environmental conditions of a particular site are crucial for fruit quality and are the basis for the development of commercial crops in a region or country (Fischer & Orduz-Rodríguez, 2012). Fruits and vegetables, which are key parts of a healthy diet, are highly exposed to the magnitude of climate change, where increased temperatures reduce production and quality, especially in the tropics and subtropics (Shukla et al., 2019).
However, global warming may also increase fruit production in some areas. Fischer and Melgarejo (2021) and Tito et al. (2018) pointed out that an overly high increase in temperature in the Andes, which would affect the growth and development of fruit trees, could be avoided by planting crops in higher altitude areas. In other parts of the world, such as India, the optimum altitudes for apple cultivation of 1,200-1,500 m a.s.l. in the 1980s shifted to 1,500-2,500 m a.s.l. in this century, with maximum elevations even exceeding 3,500 m a.s.l. (Sahu et al., 2020). However, Van Leeuven and Darriet (2016) stated that, in the case of wine grapes, the movement of vineyards to higher altitudes as the result of global warming has a high economic and social cost. Of course, there are also the possibilities of choosing species and varieties more adapted to the phenomenon of climate change (Yohannes, 2016) without moving to higher altitudes, apart from plant breeding programs such as the combination of genetic modification of high yield germplasm with proper crop management (Fischer et al., 2022). But these topics were not the objective of this review.
Ecophysiological studies are very important for finding adaptation strategies for fruit trees given changing environmental conditions (Sánchez-Reinoso et al., 2019). Given the fact that highlands over 1,500 m a.s.l. comprise almost a quarter of the planet's land surface (Mengist et al., 2020) and those tropical altitudes can be "escape zones" for global warming, the objective of this review was to characterize the effect of increasing altitudes, with an emphasis on the Andean tropics, on fruit quality to facilitate decisions for future research and for more adaptive production.
Methods
Information from different databases was used following the PRISMA guide (Preferred Reporting Items for Systematic Reviews and Meta-Analysis), applying the modified methodology of Page et al. (2021). The keywords "altitude" and "fruit quality" were used, in English and Spanish, which generated 500 article titles in the "Google Academic" database, of which 74 were used (14.8%). The SciELO database only had 19, with 3 (15.8%) used. Science-Direct listed 565 titles, of which 28 (15.8%) were used, and the Redalyc had 4,268 articles, with 57 (1.3%) useful for this review. To ensure better topicality, greater emphasis was placed on publications from 2015 onwards; however, some studies from previous years were included because of their importance.
In a second filter, research conducted at only a single altitude was excluded, with a final total of 22 studies that compared fruit quality at least two different altitudes.
Tropical altitude and climatic characteristics
Since the tropics have thermal uniformity, the largest fluctuation occurs in the highlands during the 24 h cycle of a day, where daytime can be described as summer, and night can be described as winter (Fischer, 2000).
Climatic changes with increasing tropical altitudes that affect the growth, development, and quality of fruit trees include a reduction in temperature, about 0.6 to 0.7°C per 100 m (Benavides et al., 2017), and partial pressure of gases such as CO2, O2, and N2 and water vapor (Fischer & Orduz-Rodríguez, 2012). There is also reduced precipitation which has an inverse relationship with radiation (Benavides et al., 2017), while the visible UV, infrared radiation, and wind increase with altitude (Fischer & Orduz-Rodríguez, 2012). Since the atmospheric layer that filters solar rays is thinner in high areas, UV radiation increases between 10 to 12% with each increase of 1,000 m in altitude (Benavides et al., 2017). This greater incidence of solar radiation at high elevations increases soil temperature (Fischer et al., 2022), favoring plant growth. In addition, the microclimate of a particular site can vary because of other factors, e.g., the gradient of decreasing temperature with altitude can be modified by location, time of day (Benavides et al., 2017), and cloudiness (Paull & Duarte, 2011).
This reduction in temperature with increasing altitude leads to zones that are suitable for fruit cultivation, classified in altitudinal thermal floors as described by Paull and Duarte (2011) in the equatorial zone as: (1) the hot zone (0-1,000 m a.s.l.; "warm climate altitudinal zone"); (2) the temperate zone (1,000-2,000 m a.s.l.; "temperate climate altitudinal zone"); and (3) the cold zone (>2,000 m a.s.l.; "cold climate altitudinal zone"). These authors pointed out that, in these zones, the temperature depends on latitude, wind pattern, and precipitation, among other factors.
For a specific area such as Colombia, there are recommended altitude ranges for fruit trees (Tab. 1). These ranges can even be specific to the varieties of a certain fruit species, e.g., the optimal altitude ranges in the Department of Boyacá in Colombia for the 'Anna' apple are 1,700 to 2,800 m a.s.l. (Fig. 1) (Gutiérrez-Villamil et al., 2022), for the 'Triunfo de Viena' pear 2,400 to 2,800 m a.s.l., for the Japanese plum 'Beauty' 2,600 to 2,800 m a.s.l. (Fischer, 2000), and for feijoa 1,800 to 2,700 m a.s.l. (Parra-Coronado et al., 2019).
TABLE 1 Recommended altitude ranges for Colombian Andean fruit cultivation.
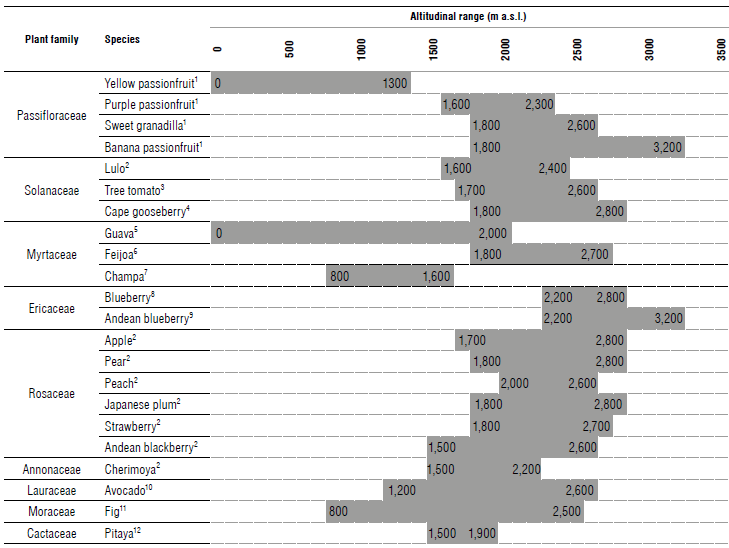
1 Fischer and Miranda (2021); 2Fischer and Orduz-Rodríguez (2012); 3Bonnet and Cárdenas (2012); 4Fischer and Melgarejo (2020); 5Fischer and Melgarejo (2021); 6Parra-Coronado et al. (2019); 7Balaguera-López et al. (2022); 8Cleves (2021); "Medina et al. (2015); 10Carvalho et al. (2015); 11Fischer, Almanza-Merchán and Piedrahíta (2012); 12Corredor (2012).
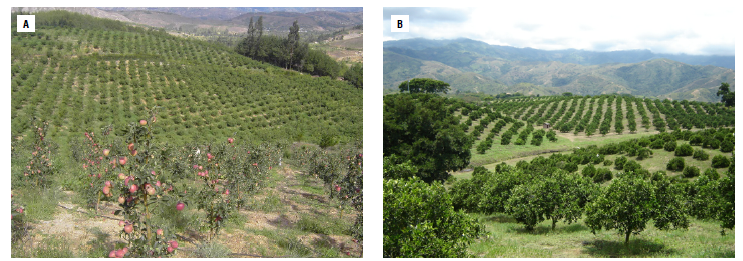
FIGURE 1 Apple orchards in the A) Boyacá highlands and B) citrus fields in the Valle department of Colombia.
Likewise, there are recommendations for a narrower range, such as those in Table 1, which may have better production results, for example, cape gooseberry at 2,200 to 2,400 m a.s.l. (Fischer & Orduz-Rodríguez, 2012), purple passion fruit at 1,700 to 2,000 m a.s.l. (Ocampo et al, 2020), fig at 2,400 to 2,500 m a.s.l. (Fischer, Almanza-Merchán & Piedrahíta, 2012), and feijoa at 2,100 to 2,600 m a.s.l. (Duarte & Paull, 2015). On the other hand, at greater proximity to the equator, temperatures increase, which is why fruit trees grow at higher altitudes there. For example, in Ecuador, there are cape gooseberry, tree tomato, and Andean blackberry crops up to an altitude of 3,300; 3,000, and 3,200 m a.s.l., respectively (Carrillo-Perdomo et al, 2015).
It is important that crops are located within altitude limits because this guarantees good physiological and productive performance, e.g., in an ecophysiological study on passion fruit in the Department of Huila (Colombia) grown at 2,060 and 2,270 m a.s.l., the predawn values of the maximum photochemical efficiency of photosystem II (Fv/Fm >0.86) confirmed that the plants were not exposed to stress conditions and the two altitudes are suitable for the cultivation of passion fruit in this region (Fernández et al., 2014). Fruit cultivars outside their optimal altitude range do not generate economic profitability, and plants at a higher altitude grow too slowly, with lower yields and qualities. At too low altitudes, they grow too fast, without developing their typical and stable post-harvest quality (Fischer & Parra-Coronado, 2020).
Adaptation of fruit trees to highland conditions
Cultivars originating from regions near the equator and/or higher altitudes, as the case of Andean fruit crops, may have developed a greater tolerance to UV-B radiation (Caldwell et al., 1980). A good example of the adaptation of fruit trees to higher altitudes is the study by Voronkov et al. (2019), who found that the levels of phenolic substances and polyunsaturated fatty acids increased in the skin of apples with altitudes increasing from 300 to 1,200 m a.s.l. in the Caucasus. This increase in phenols protects fruits against high UV radiation because of their powerful antioxidant effects, while unsaturated fatty acids retain the fluidity of the fruit's cell membranes within the physiological range (Voronkov et al., 2019).
In cape gooseberry plants, Fischer et al. (2007) found that plants formed a more superficial root system at a higher altitude (2,690 m a.s.l.) in Boyacá (Colombia) than at 2,300 m a.s.l. to take better advantage of soil warming by the midday sun. In addition, this species, which originated in the Andean highlands, can increase the number of leaf stomata at higher altitudes to compensate for the lower concentrations of CO2 and O2 at these conditions (Fischer & Melgarejo, 2020), along with dense pubescence in all aerial organs to counteract the high UV radiation and nocturnal cooling of the atmosphere (Fischer et al, 2021).
Plants at high elevations develop a lower height because UV light decreases auxin production (Fischer & Melgarejo, 2014) and affects the synthesis of gibberellins in the internodes (Buchanan et al., 2015). In addition, fruit trees develop a smaller leaf area because of the greater solar radiation and increased temperature resulting from climate change (Fischer, Almanza-Merchán & Ramírez, 2012). Moreover, the cuticle as well as the leaves becomes thicker because of the increased number of parenchyma layers that better resist UV light (Fischer & Miranda, 2021). Within this context, varieties with higher photosynthetic performance and lower susceptibility to photoinhibition are better adapted to high elevation belts (Fischer et al., 2016).
For plant breeders, climatic adaptation is the requirement that a new variety must meet, and fruit trees are continuously modified through genetic selection to adapt to new environments, such as light intensity, photoperiod, cold and heat, type of soil, and moisture condition. Many of these requirements change with altitude and latitude (Sherman & Beckman, 2003). It is noteworthy to mention the existence of a genotype-by-environment interaction, as shown in the case of guava fruits, where a significant genotype x environment interaction was found for sugar and organic acid content in four genotypes at different altitudes in the department of Santander in Colombia (Solarte et al, 2014).
Effect of altitude on fruit quality
In general, for the comparison of different altitudes, the intrinsic characteristics of each site must be considered, e.g., slope, acidity, and organic matter content of the soil, among others, along with the climatic factors (Brenes-Gamboa, 2017). Table 2 shows some studies (22) that compare the effect of different altitudes on the physical and chemical components of fruits. Pérez and Melgarejo (2015) confirmed that the environmental conditions must be very close to the optimum to achieve the highest possible yields and qualities on fruit plantations, which are determined by the genetic potential of the species.
The physical characteristics of fruits
Physical features, such as weight, size, shape, firmness, and color, along with the duration of the reproductive phenological phase, depend first of all on the genetics of the species and variety; the potential is expressed to the maximum under optimal climatic conditions of the crop (Fischer, Ramírez & Almanza-Merchán, 2012), which favor physiological processes, such as photosynthesis, transpiration, translocation of photoassimilates, respiration, and metabolism, which are crucial to the internal and external quality and postharvest longevity (Ladaniya, 2008).
The duration of fruit development until physiological maturity depends to a high degree on the temperature of the cultivation site; the lower the temperature during the growing season, the later the fruit will ripen (Moretti et al., 2010). Thus, feijoas at 1,800 m a.s.l. and an average temperature of 18.3°C took 155 d, while at 2,580 m a.s.l., (12.3°C) they took 180 d (Parra-Coronado et al., 2015); cape gooseberries took 66 and 75 d at 2,300 (17.4°C) and 2,690 m a.s.l. (12.5°C), respectively (Fischer et al., 2007). Posnette (1980) reported that the growth cycle of 'Lacatan' banana fruits in Jamaica increased by 1 month with each 100 m increase in altitude. Temperature, as one of the most important climate variables, affects the growth and development of fruit trees by regulating the length of the different phenological stages, i.e., fruit development is prolonged at higher altitudes because of decreased temperatures (Parra-Coronado et al., 2015; Mayorga et al., 2020). Ramírez et al. (2018) stated that the length of fruit development is highly dependent on climatic differences and particularly on the temperature. These authors mentioned that higher mean temperatures enhance fruit growth rates and decrease the time to maturity, whereas lower average temperatures tend to extend time necessary for fruit growth and maturity. Fischer, Ramírez and Almanza-Merchán (2012) emphasized that carbohydrate transport to the fruits and the rate of biochemical reactions catalyzed by enzymes (Moretti et al., 2010) are highly temperature-dependent.
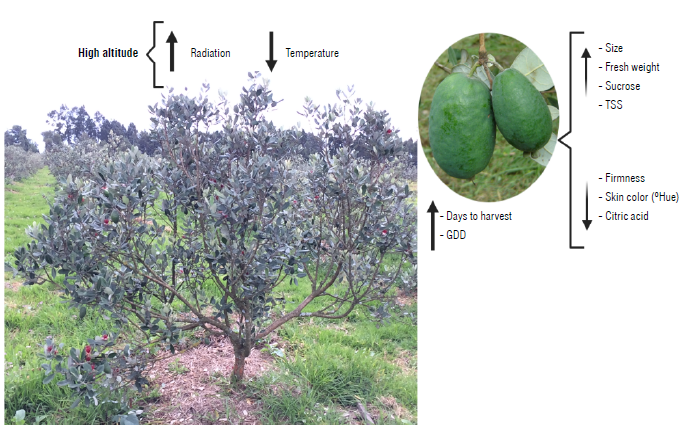
FIGURE 2 Representation of the effect of a higher altitude (2,580 vs. 1,800 m a.s.l.) on development and quality characteristics of feijoa [Acca sellowiana) fruits (Parra-Coronado, Fischer & Camacho, 2015; Parra-Coronado et al., 2017a, 2019, 2022). GDD: growing degree days; TSS: total soluble solids.
Several researchers compared growing degree days (GDD) during fruit development at different altitudes, with more physiological data and better adaptation to the temperature of the site, e.g., feijoa at 1,800 m a.s.l. requires 2,965±113 GDD (167.5 ± 4.97 calendar days) from flower bud to harvest and, at 2,580 m a.s.l., requires 2,337±124 GDD (210.25 ± 10.08 calendar days) (Parra-Coronado et al., 2015) (Fig. 2). Bugaud et al. (2006) found that for bananas, at higher elevations (300 m a.s.l. vs. 50 m a.s.l.), the sum of temperatures (measured in GDD) was higher, which is why fruit fingers developed with greater thickness and density (Tab. 2), and the green life was shorter.
TABLE 2 Effect of increasing altitude on the physical and chemical properties of fruits grown in various countries at the time of harvest.
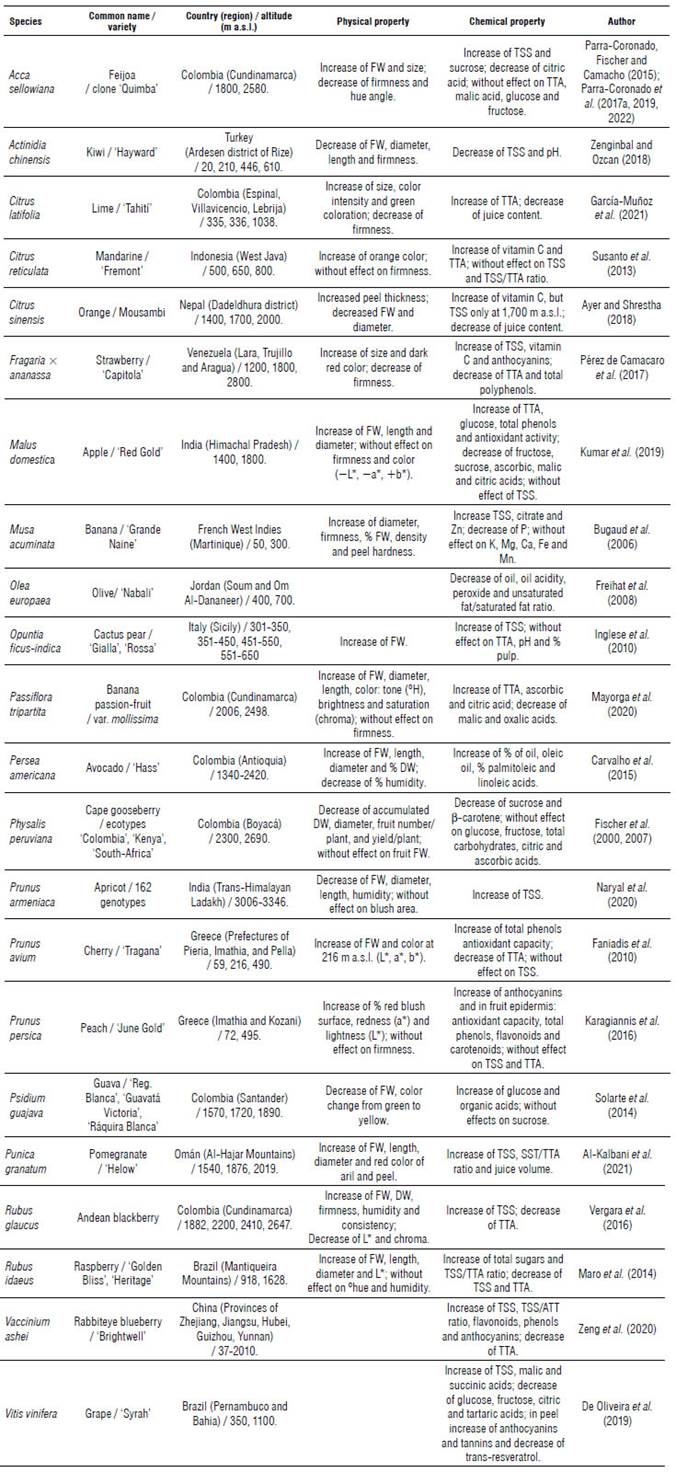
FW: fresh weight; DW: dry weight; TSS: total soluble solids; TTA: total titratable acidity.
Of the 22 studies in Table 2, the fresh weight (FW) and size of the fruit increased in 12 studies (e.g., feijoa, banana passion fruit, Andean blackberry, lime, cactus pear) with increasing altitude, while in five studies, these properties decreased (e.g., cape gooseberry, guava, kiwi); the conditions of the sites must be within the optimal range required by these fruit species (Fischer et al., 2016), especially for temperature, precipitation and solar radiation (Fischer, Ramírez & Almanza-Merchán, 2012). In this regard, Viera et al. (2019) indicated that the effect of location on fruit weight and blackberry production was due to climate and soil characteristics.
Possibly, the development of fruits with a smaller size and lower weight at lower elevations occurs because of accelerated development driven by high temperatures, as compared to higher elevations of the same species. Therefore, there is a shorter time for accumulation of photoassimilates, along with a smaller daylight integral, as observed by Mayorga et al. (2020) in banana passion fruit. The increase in fruit weight may be highly related to cooler nights at high altitudes (Fischer et al., 2016) which reduce maintenance respiration in fruits and energy costs, favoring carbon balance and dry weight (DW) accumulation in these organs (Gariglio et al., 2007).
Apart from temperature, precipitation and soil moisture play the most important role in fruit filling because of their direct effect on the rate of fruit growth and translocation of carbohydrates to the fruit (Fischer, Ramírez & Almanza-Merchán, 2012). According to Mendoza et al. (2017), precipitation and soil moisture promote the reproductive phase of crops in the Andes to a greater degree (73.4%), as compared to temperature (19.3%) and solar radiation or photoperiod (3.2%). In cape gooseberries, a decrease in precipitation (837 vs. 302 mm year-1) with the increase of altitude (2,300 vs. 2,690 m), could also have contributed to smaller fruits, number of fruits, and yield per plant (Tab. 2), although there was additional irrigation (Fischer et al., 2007). In the case of feijoa, Parra-Coronado et al. (2017a) found an opposite behavior for the size and quality of fruits, which were greater at higher altitudes (1,800 vs. 2,580 m a.s.l.) and with less rainfall (1,493 vs. 765 mm year-1) although plants were near the upper recommended altitude limit at 2,700 m (Tab. 1).
An increase in altitude only increased fruit firmness in two studies, e.g., in blackberry (Vergara et al., 2016), while in four studies, firmness decreased (e.g., in strawberry; Pérez de Camacaro et al., 2017). Four authors observed no effect on this property (e.g., in mandarin; Susanto et al., 2013). This non-uniform effect of altitude on firmness could be because this quality characteristic depends on various factors, such as fruit morphology, cell wall composition, Ca concentration, starch concentration, ethylene production, respiratory intensity, and enzymatic activity related to softening, among others (Yahia & Carillo-López, 2019), factors that can be differentially regulated depending on altitude and crop management (fertilization, irrigation).
Two studies looked at the thickness of the epidermis, as in the case of oranges (Ayer & Shrestha, 2018) and the hardness of the epidermis in bananas (Bugaud et al., 2006), which increased with higher altitude (Tab. 2), possibly promoted by higher solar radiation in these sites, which increases the number of parenchyma layers and cuticle for greater resistance against UV light (Fischer & Miranda, 2021). Additionally, Osterloh et al. (1996) pointed out an increase in the firmness of deciduous fruits growing in mountainous regions, which shortens the ripening processes and leads to a longer shelf-life and better aroma than those from valleys. In the case of the 'Kristal' guava in Indonesia, fruits from altitude (550 m a.s.l.) stood out as being "crispier", while those from a valley (200 m a.s.l.) were softer and heavier (Musyarofah et al., 2020).
These observations coincide with the increase in the quality of the 'Anna' apple at 2,500 m a.s.l. vs. lower elevations in Duitama (Colombia), with a thicker cuticle and epidermis, making the fruits less susceptible to pathogens and insect pests, along with a better red coloration because of increased anthocyanin synthesis (Fischer et al., 2016). This reaction agrees with the report by Campos and Quintero (2012) for banana passion fruit grown in the Colombian highlands (Tab. 1), which developed a thicker epidermis and was more resistant to anthracnose than fruits from lower sites.
Fruit color changes with increases in solar radiation at higher altitudes (Fischer & Orduz-Rodríguez, 2012); a greater intensity of color and green coloration were found in Lima Tahiti (Tab. 2) (García-Muñoz et al., 2021), probably because of the role of light in the biosynthesis of chlorophylls. In another citrus, mandarin cv. Fremont, the orange color increased (Ayer & Shrestha, 2018) because of the mentioned parameter. The higher altitude was also responsible for a greater increase in the intensity of red color in strawberries (Pérez de Camacaro et al., 2017) and pomegranates (Al-Kalbani et al., 2021) because of the increase in the concentrations of anthocyanins that, apart from these two crops mentioned, have also been found in peaches (Karagiannis et al., 2016), blueberries (Zeng et al., 2020) and grapes (Oliveira et al., 2019) (Tab. 2). Light is a determining factor in the accumulation of pigments (carotenoids and anthocyanins) in fruits (Yahia & Carrillo-López, 2019). It has been shown that light is an important component that affects the expression of genes related to the synthesis of pigments and also regulates their accumulation by controlling the light signaling apparatus (Azari et al., 2010; Ruiz-Sola & Rodríguez-Concepción, 2012).
Apart from light, temperature is a key factor for the red color of fruits; day temperatures around 18-24°C promote fruit growth (Fischer & Orduz-Rodríguez, 2012), depending on the species and variety, while cool night temperatures (e.g., 10°C) promote red coloration by anthocyanins in the fruit epidermis, as reported by Musacchi and Serra (2018) for the case of red apples, while Fischer et al. (2016) mentioned that cool nights promote the coloration of wine grape berries. These temperature contrasts can easily be found at tropical altitudes.
Parra-Coronado et al. (2017a) measured color during the growth of feijoa (cv. Quimba) fruits as a function of hue angle (H°), which varies from 180° for the pure green color to 0° for the pure red color, and found that feijoa remained a green fruit, with small decreases in H° for the two altitudes (1,800 and 2,580 m a.s.l.), with values at the time of harvest of 122.9 ± 2.0 and 125.0 ± 2.1 H°, respectively. Although an increase in temperature with a decrease in altitude accelerates the ripening process, degradation of chlorophyll and reduction of H° in the skin of feijoa fruits do not change its color because of the genetics of the fruit, which only varies within a color tonality.
Chemical characteristics of fruits
Total soluble solids (TSS), mainly soluble sugars, organic acids, and vitamins, increased in nine fruit species (e.g., feijoa, Andean blackberry, and cactus pear) at increased altitudes, as seen in Table 2. In two fruit species, kiwi (Zenginbal & Ozcan, 2018) and raspberry (Maro et al., 2014), they decreased with increasing altitude. The increase in TSS may be related to the increase in photosynthesis with altitude because of the higher luminosity (Mayorga et al., 2020) if the temperature is still in the optimal range of the crop and/or with the lower respiratory carbohydrate loss as a result of the lower night temperature at high elevations (Fischer et al., 2016). For the reduction of total soluble solids (TSS) in kiwi, Zenginbal and Ozcan (2018) found that the conversion of starch into sugar decreased due to lower temperatures at altitude of 610 m a.s.l. Temperature can influence total sugar content in yellow and purple passion fruit that are grown at different altitudes and temperature conditions (Viera et al., 2022). In addition, low night temperatures can limit the transport of carbohydrates to fruits (Fischer, Almanza-Merchán & Ramírez, 2012), as seen by Tombesi et al. (2019) in grapevines exposed to a night temperature of 15°C as compared to 25°C.
The total titratable acidity (TTA), which is the quantification of the organic acids contained in the cell juice, decreased in five species at increased altitude, especially in the berries (blackberry, raspberry, blueberry) (Tab. 2). In four species (lime, mandarine, apple, banana passionfruit), the TTA increased with elevation, possibly because the lower temperature limited the respiration rates of fruits that use organic acids as a substrate (Batista-Silva et al., 2018). In strawberries, blackberries, and blueberries, increases in TSS were recorded in association with a decrease in TTA with increased altitude, and the TSS/TTA ratio (maturity index) increased (Tab. 2), which could be related to the conversion of organic acids into sugars by the gluconeogenesis process (Famiani et al., 2015).
In apple, cherry, peach, and blueberry, increases in phenols were detect at higher altitude; in the latter three species, an increase in flavonoids was also reported (Tab. 2), which is a very important protection mechanism against UV radiation (Cheynier et al., 2013). On the other hand, total phenol contents decreased with higher altitude only in strawberries (Pérez de Camacaro et al., 2017). These secondary metabolites are essential phytochemical substances in the plant, which are activated by the light spectrum, acting as defense compounds and protectors against UV radiation (Salazar-García et al., 2016).
Likewise, the antioxidant capacity and activity increased with increased altitude in peaches (Karagiannis et al., 2016) and apples (Kumar et al., 2019), possibly generated by the increase in phenols, flavonoids, and ascorbic acid (especially in citrus fruits, strawberries, and banana passion fruit) (Tab. 2). These secondary metabolism substances are powerful antioxidants (Ouzounis et al., 2015).
In two (Tahiti lime and orange) of the three citrus species studied (Tab. 2), a decrease in juice content was found with increasing altitude, e.g., a 14.8% lower juice content was produced in Tahiti lime fruits at 1,038 m as compared to 336 m altitude (García-Muñoz et al., 2021). Possibly, this occurred because the climatic conditions were not adequate for the juiciness of the fruit, taking into account the multidimensionality of climatic factors in a given site (Zandalinas et al., 2021). The volume of juice also increased in pomegranates, according to the increase in weight and size of the fruits with altitude (Al-Kalbani et al., 2021) (Tab. 2).
In fruits with high lipid content, such as the olive tree, the oil content decreased (Freihat et al., 2008), while it increased in avocados (Carvalho et al., 2015) with rising altitude (Tab. 2). In the latter fruits, the contents of oleic, palmitoleic, and linoleic fatty acids increased at higher elevations. Environmental factors at different altitudes influence the respiration pattern of avocados during ripening on the tree, affecting the metabolism of the fruit that is responsible for the composition and quantity of fatty acids (Carvalho et al., 2015). The increase in altitude to values above 2,300 m a.s.l. was reported by Henao-Rojas et al. (2019) as a favorable one for the fruit quality of this species, while Ramírez-Gil et al. (2018) mentioned the altitude range for avocado is between 1,400 and 2,600 m a.s.l.
There are very few studies that reported a lack of an effect from increasing altitude on fruit quality; one on highbush blueberries, comparing elevations of 217 and 636 m a.s.l. in Portugal, found no statistical differences for the concentrations of free sugars, organic acids and vitamin C (Correia et al., 2016).
In the two studies that evaluated the content of antioxidants in the epidermis of fruits, such as total phenols, flavonoids, and carotenoids in peaches (Karagiannis et al., 2016) and anthocyanin and tannins in grapes (Oliveira et al., 2019), antioxidants increased with increasing altitude, confirming the importance of these protective substances in the skin of fruits that attenuate excessive UV radiation (Caldwell et al., 1998). Interestingly, in bilberries (Vaccinium myrtillus), a direct relationship was found between altitude and antioxidant activity; in the range between 900 and 1,450 m a.s.l., the relationship between altitude and total anthocyanins was inversely proportional, while the dependence became proportional from 1,500 m a.s.l. (Papanov et al, 2021).
In general, if the highest altitude of studied sites was very close to or above that recommended for the crop (Tab. 1), the physical and chemical components of the fruit quality decreased or there were no differences between the sites, as observed in the case of cape gooseberries at 2,690 vs. 2,300 m a.s.l. in Colombia (Tab. 2) (Fischer et al., 2000, 2007).
In feijoa, the accumulated solar radiation during fruit growth (8,918 vs. 11,082 W m-2) at the two altitudes (1,800 vs. 2,580 m a.s.l.) affected its size and quality at harvest (Fig. 2) (Parra-Coronado et al., 2017a). At higher altitudes (greater accumulated solar radiation), the feijoa fruits presented a larger size, higher content of TSS and less firmness of the epidermis. According to Parra-Coronado et al. (2017a), the higher content of TSS, and greater FW produced at higher altitudes could be explained by "the higher rate of transpiration related to greater irradiance, which would provide a prolonged entry of water and nutrients to the fruit", indicating that the greater availability of light increases and extends the transport stream from the xylem to the fruits. Furthermore, photosynthesis in adjacent and well-illuminated leaves near the fruit is promoted by the attraction of photoassimilates from the fruit (Fischer, Almanza-Merchán & Ramírez, 2012).
Physiological disorders of fruits at high altitudes
Fruit trees grown at altitudes above their optimal range can suffer abiotic stress, especially from low temperatures (chilling and freezing stress, Fig. 3A), and oxidative stress from high UV radiation, which can cause large production losses (Madani et al., 2019). On clear nights, the surface of the outer tissues of plants and fruits cool below air temperature because of heat exchange, leading to damage to cell membranes and intracellular compartments (Voronkov et al., 2019). In addition, sudden changes in temperature between day and night at altitudes can generate physiological disorders, such as fruit cracking (Fischer, Balaguera-López & Álvarez-Herrera, 2021), as reported by Miranda (2020) for sweet granadilla.
During recent decades, radiation has increased steadily, especially UV-B (280-320 nm) (Van Leeuwen & Dariet, 2016). Fruit trees, growing at higher altitudes and especially near the equator, suffer from prolonged solar radiation affecting the epidermis of the fruit, as observed in crops such as mango, kiwi, pineapple, avocado, sweet granadilla (Fig. 3B) and deciduous fruit trees (Fischer, 2000; Fischer & Orduz-Rodríguez, 2012), and from a higher concentration of potentially harmful UV-B (Benavides et al., 2017).
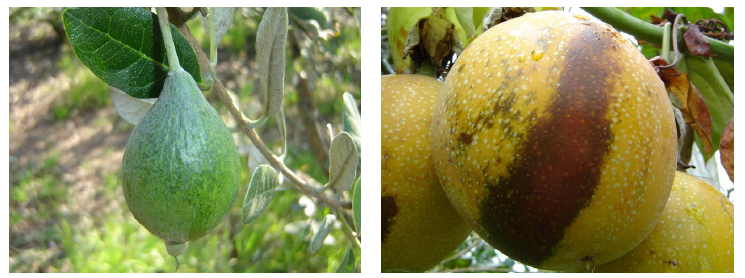
FIGURE 3 A) Chilling injury in feijoa (Acca sellowiana) and B) sunburn in sweet granadilla (Passiflora ligularis) in Colombian highlands.
Fruit sunburns which are common at altitude sites are further promoted by prolonged dry periods due to climate change and the effect of "El Niño" in northern South America (Fischer et al., 2016). Damage caused by photo-inhibition results when the chlorophylls in the thylakoid membrane of the chloroplasts absorb more light energy than the photosynthesis process can use and induce damage to the photosystem II and degradation of D1 protein (Casierra-Posada, 2007).
Direct solar radiation increases not only the temperature but also evapotranspiration through the fruit surface,causing an accelerated loss of moisture and increasing susceptibility to fruit cracking (Ikram et al., 2020; Fischer, Balaguera-López & Álvarez-Herrera, 2021). For example, in the case of Japanese plums (Prunus salicina), excessive radiation manifests itself as a brown to yellow discoloration on the surface of the fruits, which, in severe cases, can result in necrotic spots and cracking of the fruit epidermis (Makeredza et al., 2018).
In sensitive plants, prolonged UV-B radiation affects photosynthetic activity and plant growth by damaging DNA, membranes, proteins, and lipids (Hideg et al., 2013). However, normal (natural) levels of UV-B radiation can reduce abundant vegetative growth and the incidence of pathogens, and favor secondary metabolism from greater synthesis of anthocyanins, carotenoids, and flavonoids, improving flavor, aroma and color in the case of grapes (Fischer et al., 2016).
Fruits are capable of developing protection mechanisms against high UV-B radiation, such as increased synthesis of phenylpropanoids (flavonoids, e.g., anthocyanins) in the epidermis that can absorb this radiation and serve as antioxidants (Caldwell et al, 1998).
Conclusions
In this review of most information from Colombia, higher altitudes are characterized by decreased temperatures and increased solar radiation. Because of global warming, highlands will become important for planting fruit trees. The effect of altitude on the quality components of fruit trees depends, firstly, on the species and variety and, secondly on whether the climatic conditions are within the range of optimal conditions for the fruit crop growth.
In the 22 studies found in the systematic review of four bibliographic databases that compared fruit quality at least two altitudes, increased altitudes showed prolonged fruit development in most cases, and increased fresh weight and size, while, in some fruit species, fruit firmness decreased. In general, the higher radiation in the upper zone promoted the intensity of the fruit color. The total soluble solids content of the fruits increased with altitude in most cases, probably because of the increase in photosynthesis resulting from higher solar radiation. For total titratable acids, there was no clear trend for altitude, and it depended more on the fruit species and the site conditions. In various fruit species, increasing altitude means the content of antioxidants (mainly phenolics) is increased in the fruit flesh and epidermis as a reaction to increasing UV light. Physiological disorders in the highlands include stress caused by high UV radiation (oxidative stress) and too low temperatures (chilling and freezing stress).
In general, if altitude is at or above the recommended range for a fruit species, there are no positive effects on fruit quality. Varieties with higher photosynthetic performance and lower susceptibility to photoinhibition are better adapted to high altitudes.
The authors suggest undertaking more studies in different altitudes of the Andean region with all fruit species and varieties in correlation with the climatic conditions for a better understanding of fruit production and quality. These results, in the face of climate change, would serve as an input for new plant breeding programs and improved management of these crops.