Introduction
The IPCC (Intergovernmental Panel on Climate Change) reports for the Northwest of Mexico indicates significant thermal variations with temperature increases between 2°C and 4°C for the next 20 years (IPCC, 2014). In this regard, the national and international scientific community state that some crops will show variations from 10% to 30% in their physiological and agronomic performance (Giménez et al., 2021; Sadeghi et al., 2022). The Yaqui Valley is one of the Mexican northwestern regions with significant temperature increases, and wheat (Triticum durum Desf.) is one of the most negatively affected crops (Garatuza-Payán et al., 2018).
Some studies of climate change impact on wheat have explained the effects of temperature increase on morphological, physiological, biochemical, molecular, and agronomical indicators (Argentel-Martínez, Arredondo et al., 2019; Asseng et al., 2019; Liu, 2019; Impa et al., 2021). Some other studies are based on parameterized models, using controlled and semi-controlled experimental approaches (Asseng et al., 2015; Ahmed et al., 2017; Chenu et al., 2017; Zhao et al., 2019), but few studies have explained the possible variations of root morphology and the associated microbial diversity under climate change scenarios. In this context, the associated microbial diversity contributes significantly to mineral nutrition by increasing the availability of some nutrients, mainly nitrogen, phosphorus, and also, the maintenance of humidity in the rhizosphere (Begum et al, 2019).
In the Yaqui Valley, the region with the highest wheat production in Mexico, with more than 41% of the national production (Argentel-Martínez, Arredondo et al., 2019), some studies explored the relationship between climate change and microbial diversity (Parra-Cota et al., 2018; Ingraffia et al., 2019) but none reported the arbuscular mycorrhizal fungi (AMF) effects on wheat crop and their relationship with climate change. Hence, wheat production vulnerability predicted for the next ten years in the Yaqui Valley due to climate change scenarios (Garatuza-Payán et al., 2018) could be exacerbated if beneficial microorganism diversity decreases in the region, where the contents of soil organic matter are very low (Parra-Cota et al., 2018).
For this reason, the present study aimed to evaluate the effect of canopy temperature increase of 2°C on the final root density and the colonization percentage and diversity of the symbiotic association AMF-wheat under field conditions in the Yaqui Valley, Sonora, Mexico.
Materials and methods
Experimental area and treatments
This experiment was carried out during three crop cycles (2016-17, 2017-18, 2018-19) in order to evaluate the effect of a canopy temperature increase of 2°C on agronomic performance of wheat under field conditions (30o27'50.83" N, 60o73'93.24" E) in the Instituto Tecnológico de Sonora, México (Garatuza-Payán et al., 2018; Argentel-Martinez, Garatuza-Payán et al., 2019). Five adjacent plots with increased temperature in relation to the ambient canopy temperature were established with a randomized design during the 2016-17, 2017-18, and 2018-19 crop cycles, using the crystalline wheat variety CIRNO C2008. Treatments consisted in increasing canopy temperature by 2°C (warming treatment) above the ambient temperature (control treatment). At the end of the experiment, arbuscular mycorrhizal fungi (AMF) colonization was evaluated.
The soil in the experimental area was classified as compacted vertisol (González et al., 2003). The national classification of this soil correlates with Halic Haplusterts according to the soil genetic classification methodology developed by Soil Taxonomy (Bockheim et al., 2014), which also correlates with the classification proposed by World Reference Base (Mosleh et al., 2017).
For higher experimental accuracy, a soil chemical analysis was carried out in the Edaphology Laboratory of the Technological Institute of Sonora (Tab. 1) following the Official Mexican Norm (NOM-021-RECNAT, 2000), which specifies fertility, salinity, and classification of soils. The main chemical feature was the electrical conductivity (EC) value of 1.96 dS m-1, which classified this soil as saline (Nell & Van Huyssteen, 2014). The soil organic matter content (OM) was low, suggesting a decrease in microbial diversity (Ding et al., 2014).
Temperature manipulation and control
A temperature increase of 2°C in the crop canopy was applied 15 d after seedling emergence in all crop cycles using six thermal radiators per plot (FTE-1000 model, 1000W, 240 V, 245 mm long x 60 mm wide, Mor Electric Company Heating Association Inc. Comstock Park, MI, USA (Kimball, 2015). The radiators formed a regular hexagon which effectively increased canopy temperature with respect to the adjacent plot at ambient temperature; this was based on an electronic integrative and degradative algorithm that simulates a climate change scenario for the Yaqui Valley for the next 20 years (Kimbal, 2015).
The temperature was controlled using infrared temperature sensors (IRTS Apogee Instruments Inc., Logan, UT, USA) installed on both the control and warming plots (five plots, respectively) with an inclination degree of 45° with respect to the soil surface, covering a circle of r = 1.5 m at the center of the plots. Warming was done at a height of 1.20 m of the canopy and started 15 d after the seedling emergence until the harvest (Garatuza-Payán et al., 2018).
Soil preparation, seed sowing, and agronomic management
Soil preparation was carried out by the traditional method in the three experimental crop cycles, following the specifications of the technical instructions for wheat in southern Sonora (Figueroa-López et al., 2010), in order to achieve a soil granular structure.
The sowing was carried out on December 7th; 8th and 16th of 2016, 2017, and 2018, respectively, with a URBON-GH sowing machine at a depth of 8 cm, with a towing capacity of six furrows with three rows each, regulated to a density of 170 kg ha-1.
Prior to sowing, bottom fertilization was carried out to a standard of250 kg ha-1 of urea + 50 kg ha-1 of mono-ammonium phosphate fertilizer (MAP, 11-52-00). The fertilizer was incorporated during the furrow conformation.
The second and third nitrogen fertilizations were applied immediately before the first and second irrigations at a dose of 50 kg ha-1 of urea during tillering and booting phenological phases, respectively. All irrigations were applied at an average depth of 14 cm and at an average irrigation interval of 25 d, when the soil had approximately 75% of field capacity.
Initial and final root density measurements
Four soil rhizosphere samples were extracted from each plot at soil depths of 0-20 cm, 20-40 cm, and 40-60 cm in both treatments at leaf growth phenological phase (30 d after emergence) and during grain ripening (kernel hard). Subsequently, the soil was separated from the roots with water; the roots were cut at the base of the stem and the root volume was measured by volumetry with a glass measuring cylinder that contained an initial volume of 50 ml of water (Tennant, 1975).
Spore quantification and mycorrhizal colonization
During the ripening phenological phase, three samples of plants and rhizosphere soil were taken from each repetition of treatment to quantify the number of AMF spores present on roots and determine the percentage of mycorrhizal colonization. Three replicates of 20 g of soil from each soil sample were processed by the wet sieving technique (Gerderman & Nicolson, 1963) and sucrose gradient (Walker et al., 1982) to obtain and quantify the AMF spores. Only viable spores were considered in the quantification, discarding broken, damaged and/or parasitized spores. The root material was washed with distilled water and the thinnest root samples were cut into fragments of 4 cm in length. Later they were stained by the Trypan blue method (Phillips & Hayman, 1970).
The stained roots were mounted in a gridded Petri dish (1 x 1 cm) to perform colonization quantification under the stereoscope (4X) using the intercepts method (McGonigle et al., 1990). Counts were performed in duplicate. The presence of any fungal structure (arbuscules, vesicles, or hyphae) in the interior of the roots was considered an indicator of colonization (Sangabriel-Conde et al., 2014).
Statistical analysis
After verifying the fulfillment of the theoretical assumptions of normality and variance homogeneity (Komolgorov, 1933) of each evaluated variable (the initial and final root densities and the number of spores by kg of soil), the means, standard deviation, and standard error were calculated. The crop cycles were taken as repetitions due to the similarity of the data. The means were compared following a hypothesis test for continuous quantitative variables, using the theoretical distribution of t-Student for P<0.01. Warming stress intensity (WSI) was determined for both variables evaluated following the formula proposed by Fernández (1993): WSI = 1-(variable under warming/variable under control). Subsequently, the variables were compared using a t-Student hypothesis test. For all analyses, the statistical program STATISTICA version for Windows (StatSoft, 2014) was used.
Results
The presented data in each treatment are the result of the average of the three crop cycles due to the absence of significant differences (P= 0.08322). The highest root density (51%) was concentrated at a depth of 0-20 cm in the control treatment; however, at this depth, in the warming treatment, the root density was only 27%. In contrast, warming grouped the major root percentage (52%) at a depth of 20-40 cm. The present result indicates that the imposed warming, although intended to create a canopy warming (Kimball, 2015) , caused a greater root penetration, which perhaps could contribute to increased mineral uptake available at deeper strata (Tab. 2). On the other hand, at a depth of 40-60 cm, only 16 and 15% of the total volume of roots was found for the control and warming treatments, respectively.
TABLE 2 Initial and final root density at different soil depths (0-20; 20-40; 40-60 cm) under warming and control treatments [(t value): calculated t value; df: degrees of freedom; p: error probability].
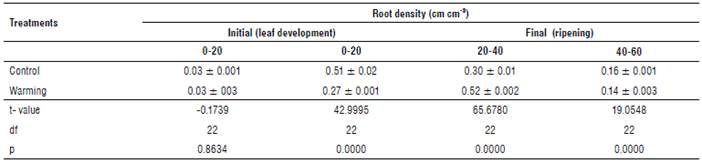
Root growth is critical for crops to use soil water under water-limited conditions. A study conducted by Oussible et al. (1992) to investigate the effect of available soil water on root and shoot growth under deficit irrigation in a semiarid environment demonstrated the relationship between root density and leaf area. In the present study, the increase in the deep root system under warming was a morphological mechanism activated to avoid water stress. This mechanism helps to increase water and mineral uptake during adaptation to water stress due to warming conditions (Chen et al, 2019).
There are many factors that contribute to regulating AMF colonization, such as root density, root exudates, and the availability of nutrients in the rhizosphere (Smith and Read, 2008). In this study, the decrease in root density in the warming treatment was determined according to the obtained AFM colonization percentage.
Arbuscular mycorrhizal fungi colonization and the number of spores in soil
There was a highly significant difference (P=0.0002) in the percentage of AMF colonization in the roots of the crop because of the imposed warming (Fig. 1), with a reduction of 16.7% in the warming treatment with respect to the control treatment. The colonization percentage obtained in the control treatment agrees with Ingraffia et al. (2019), who found approximately 29-30% colonization in wheat.
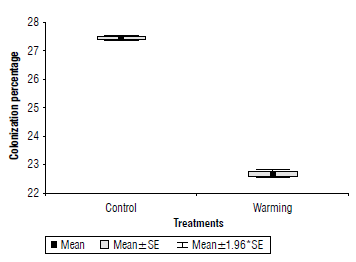
FIGURE 1 Colonization percentage of AMF in wheat under warming and control treatments. SE: standard error of the mean.
This result indicates the adverse effect of temperature increase, as is predicted for Yaqui Valley, in the reduction of fungal diversity and a significant reduction in the colonization percentage. According to Schalamuk et al. (2006), changes in soil management can result in crop species without AMF or without certain AMF species because they: (i) cannot tolerate new soil conditions; (ii) are not able to infect the host plant under these conditions; or (iii) are not able to compete with other species of AMF fungi that have become dominant due to new growth conditions (Sieverding, 1991). These results confirm the result obtained in our study (Fig. 1) by reducing the presence of AMF spores by the applied treatment.
The number of spores per 1 kg of soil also decreased by 38.5% (P=0.00031) because of the warming imposed (Fig. 2); the standard deviation of the control treatment was higher, but this variability did not negate the significant differences found.
Warming stress intensity for AMF colonization and the number of spores in soil
When analyzing the intensity of stress in the evaluated variables, greater (18%) sensitivity was found in the percentage of colonization, with highly significant differences with respect to the number of spores per 1 kg of soil (Fig. 3).
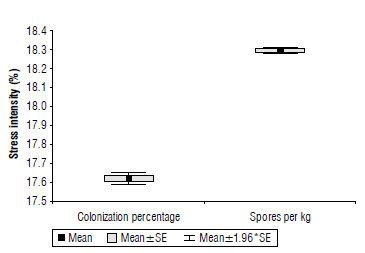
FIGURE 3 Stress intensity for colonization percentage and the number of spores per kg of soil. SE: standard error of the mean.
Stress intensity is a calculated parameter that indicates the severity of stressful conditions on biological variables (mainly root length and plant height) and measures tolerance to some stressful conditions. The present study demonstrated that AMF spores can prevail in the soil, but due to the warming effect, their symbiosis capacity and diversity decrease. This result could affect the availability of some mineral nutrients and water for plant development, reducing agronomic performance of wheat under warming conditions.
Conclusions
Greater root density in the warming treatment was found at greater depths (20-40 cm) than in the control treatment. This response constitutes a morphological tolerance mechanism for heat stress in the variety used in the experiment. Warming caused a 38% decrease in the presence of arbuscular mycorrhizal fungi on wheat roots. The imposed warming generated stress intensities of 18 and 17% in the number of spores per kg of soil and colonization percentage, respectively.