Introduction
Couscous is a North African meal prepared with wheat and chia, which are highly appreciated for their nutritional flour and considered in some countries as a rice substitute content, especially by gluten-free consumers (Boukid et al., with better nutritional qualities given its higher protein 2018). Additionally, from the farmer perspective, pseudocontent. However, around 2% of the world population is in- cereals are known for their high adaptability and low needs tolerant to wheat main protein gluten (Kaushik et al., 2015).
Nowadays, consumers are interested in natural and unconventional foods as a consequence of the problems seen in human health and nutrition. There is a renewed interest in pseudo-cereal grains: amaranth, quinoa, buckwheat, and chia, which are highly appreciated for their nutritional content, especially by gluten-free consumers (Boukid et al., 2018). Additionally, from the farmer perspective, pseudo-cereals are known for their high adaptability and low needs in terms of irrigation, fertilization, and energy, turning them into attractive products to grow in the rural areas (Santra & Schoenlechner, 2017). These pseudo-cereals are nowadays being used as raw materials or as functional ingredients in modern diets (Boukid et al., 2018).
Quinoa, an Andean pseudo-cereal with a protein content of around 14% (Navruz-Varli & Sanlier, 2016), has received attention as a wheat substitute for people with gluten intolerance (Jacobsen, 2003). Most importantly, it could satisfy the nutritional requirements of the population as it has all essential amino acids, a high content of a range of vitamins and minerals, and health-beneficial phytochemicals such as saponins, phytosterols, and phytoecdysteroids (Koziol, 1992; Jacobsen, 2003; Navruz-Varli & Sanlier, 2016). Quinoa is considered a high potential crop due to the rapid expansion of its harvested area. As a result of its nutritional status, its high resilience to agro-ecological extremes and its high tolerance to drought and salinity, quinoa is nowadays cultivated or tested in over 95 countries (Jacobsen, 2003; Ruiz et al, 2014; Bazile et al., 2015).
Traditional couscous is a mixture of wheat flour and water and can be made by hand or using specific machinery. Some research has been done aiming to mechanize couscous production and to improve its nutritional value. For example, Debbouz and Donnelly (1996) studied extrusion technology to obtain uniform couscous grains; Hafsa et al. (2015) studied the feasibility of the use of different equipment (e.g., vertical and horizontal mixers, fluidized bed reactors and spray dryers) as mechanical technologies for the agglomeration process. Other studies have focused on using different ingredients to improve the agglomeration process or the nutritional value of couscous. For example, Barkouti et al. (2012) analyzed the influence of different binding agents in the physicochemical properties of couscous. Their study demonstrated that any of the studied agents could be used for nuclei association during the agglomeration stage. In the nutritional area, Benatallah et al. (2008) analyzed the use of three different formulas to obtain gluten-free couscous. Their product was made of a mix of rice semolina with pea, chickpea, or fava bean. The results showed that the three mixtures could be used for couscous production, but a consumer acceptance test showed a preference for traditional wheat couscous followed by the rice-fava bean mix. Demir et al. (2010) replaced wheat flour with different percentages of chickpea flour and the results showed that a 50% replacement didn't affect the product's sensory and functional properties. More recently, Chemache et al. (2019) analyzed the agglomeration properties of manioc, corn, amaranth, buckwheat, and quinoa flours, obtaining positive results for the last four.
Jacobsen (2003) suggested that the advantageous properties of quinoa must be exploited through the development of new products and adequate process technologies; to date, its use as a substitute for couscous preparation has not yet been researched. This paper aimed to address this gap and offer an alternative gluten-free product with high nutritional value that could be broadly consumed by humans, including those with gluten intolerance. Quinoa alone was used as the main ingredient for the couscous preparation, given its high protein content, nutritional value, and agglomeration capacity. The study identified the best conditions for the agglomeration, cooking, and drying stages to obtain a suitable product and compared the final product's functional, chemical, and sensorial properties with those of a commercial wheat couscous.
Materials and methods
The commercial quinoa (Chenopodium quinoa Willd., Tunkahuan variety) grains (without saponin) provided by Cereales Andinos Cia. Ltda (Ecuador) were used to obtain the flour for the couscous preparation. Polysorbate 20 (Sigma Aldrich) at different concentrations was used as a binding agent for the flour-water mixture during the agglomeration process. The study used a commercial non-flavored local wheat couscous for chemical and sensorial comparisons.
Preparation and characterization of the quinoa flour
Fine and coarse quinoa flours were obtained using two milling processes. For the fine flour, clean grains (without saponin as certified by Cereales Andinos Cia. Ltda.: certification code: QUI02-5152301) were milled using a pin mill (ALPINE, UPZ 160, Germany). The flour passed through a vibrating sieve (WS TYLER, TX-29, USA), and the material collected between sieves 70 and 140, with a particle size between 106 and 250 µm, was used as fine flour (INEN, 2013). For the coarse flour, grains without saponin were milled using a disc mill (ELECTROLUX, N4, Sweden) and passed through ASTM sieves 20 and 40 to collect the material with a particle size between 425 and 850 µm. Proximal analysis of the quinoa flour was carried out according to the Association of Official Analytical Chemists (AOAC, 2005) for moisture (925.10), ash (923.03), protein (2001.11), fat (920.39), and the International Association for Cereal Science and Technology for crude fiber (ICC-STD#113) (ICC, 1972).
Conditions for the agglomeration process
The agglomeration process is a critical step-involving wetting and mixing the fine and coarse flour, leading to a particle size enlargement. According to Silva Castro (2014), this process has three stages: a first stage where the liquid comes into contact with the flour and forms the agglomerate's nuclei; a second stage where these nuclei are compacted and enlarged due to the collisions against each other, against dry flour particles or the against the container surface; and a final stage where the agglomerates start breaking down due to particle impacts. The agglomeration process was carried out according to the procedure of Barkouti et al. (2012), with some modifications. First, 150 g of fine and coarse flour (ratio according to the experimental design) were homogenized for 2 min at 136 rpm using a mixer with a flat beater (HOBART, N50, USA). With the mixer switched on, the polysorbate solution was sprinkled on the mixture at 0.32 g s-1 using a compressor device (THOMAS, AS-06, China).
After this wetting process, the product was mixed for 30 additional sec. Finally, once the agglomerates were formed, they were sieved (ASTM sieves 10, 20, and 40) for 2 min to collect particles of the correct size. According to the Codex Alimentarius (FAO & OMS, 2007), the optimal size of the agglomerated particles should be between 850 and 2000 µm.
Four factors at two levels each were studied using a 2x experimental design to find the optimal conditions during the agglomeration process. The factors were: a) ratio of fine/coarse quinoa flour: 70/30 and 75/25, b) moisture of the mixture: 35 and 40%, c) polysorbate concentration in the solution used to wet the mixture: 0 and 3%, and d) temperature of the polysorbate solution: room temperature (approx. 20°C) and 70°C. The output variable for selecting the best agglomeration conditions was yield, calculated as the ratio of the weight of the particles between 850 and 2000 µm and the initial weight of the mixture.
An ANOVA test was performed (P<0.05) to evaluate the significant effect of the factors under study and their interactions on the yield of the agglomeration process. Additionally, mean values of all treatments were compared using a Duncan multiple comparison test.
Conditions for the steam-cooking and drying processes
The steam-cooking and drying of the agglomerates aim to improve their stability. The use of heat promotes the starch gelatinization and the cross-linking of the proteins, which in turn reinforce the product's structure (Cuq et al., 2011).
The agglomerates obtained under the best conditions selected in the previous stage were placed in a thin layer on a perforated stainless-steel tray, which was put into a steam cooker (MIRMEG, Ecuador) at 92°C. The cooked product was then dried in a stove (POL-EKO, SLW 115 ECO, Poland) and sieved using an ASTM sieve 20.
Three factors at two levels each were studied using a 2x3 experimental design with one central point to find the optimal cooking and drying conditions. The factors were: a) cooking time: 15 and 30 min, b) drying time: 60 and 120 min, and c) drying temperature: 50 and 70°C. The output variables were the functional properties of the final product: Water Absorption Index (WAI), Swelling Power (SP), and Water Solubility Index (WSI), as described by Anderson et al. (1969). The selected conditions maximized the WAI and SP and minimized WSI, which help preserve the structure of the quinoa flour agglomerates.
Multiple ANOVA tests (P<0.05) were performed to evaluate the significant effect of the factors under study on the functional properties of the final product (e.g., WAI, SP, WSI). A multiple comparison test was used to compare the means of the functional properties obtained for the different treatments. The technique of multiple response variable optimization was used to identify the optimal cooking and drying conditions that maximize WAI and SP and minimize WSI.
Functional, chemical and sensory properties of quinoa vs. wheat couscous
The functional (e.g., WAI, SP, WSI), chemical, and sensory properties of quinoa couscous prepared at the selected agglomeration, cooking, and drying conditions were analyzed and compared with the properties of commercial wheat couscous.
Additionally, a micrograph showing the morphological characteristics of the quinoa couscous was obtained with a scanning electron microscope (ASPEX, PSEM eXpress, USA) and compared with the micrograph for wheat couscous published by Hafsa et al. (2015).
For the chemical properties that quantify the nutrients in the product, a proximal analysis was carried out according to the Association of Official Analytical Chemists (AOAC, 2005) and the International Association for Cereal Science and Technology (ICC, 1972).
For the sensory analysis, 12 semi-trained panelists tasted quinoa and commercial wheat couscous (Martino Prestige Couscous) samples and stated their overall preferences. Additionally, applying a paired comparison between the two samples, the panelists evaluated the color, flavor, odor, texture, and the presence of odd flavors or aromas (Hernández, 2005). Couscous samples were prepared using hot water at 92°C (relation water/couscous of 1:1). The selected panelists were persons involved in the food field that had taken at least one course on sensory analysis. Panelists were trained to detect, recognize and describe the characteristics of interest of this product. For this training, two couscous samples (different from the ones analyzed in this study) were used.
Results and discussion
Characterization of the quinoa flour
The results for the proximal analysis of the quinoa flour (Tunkahuan variety) are presented in Table 1, together with published data reported by Navruz-Varli and Sanlier (2016) for quinoa, wheat, and rice used for comparison purposes.
TABLE 1 Chemical characterization of quinoa flour (author data), compared to other grains highly consumed in developing countries (values are based on dry matter).
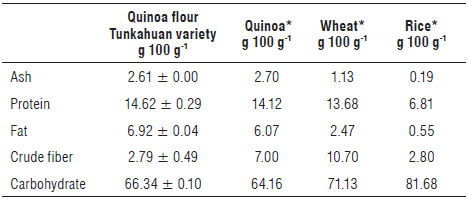
±a; n = 2.
The results for ash, protein, fat and carbohydrate obtained for the quinoa flour used in this study are in a similar range of the values reported by Navruz-Varli and Sanlier for quinoa grain (2016). When comparing quinoa with the most consumed staples in developing countries, its protein content (14.62%) is higher than that of wheat (13.68%) and rice (6.81%) (Navruz-Varli & Sanlier, 2016), and also than the protein of corn (8.50%), manioc (0.50%) and buckwheat (12.00%) (Chemache et al., 2019). The amount of fiber in the quinoa flour sample is the lowest because the grain already underwent a saponin elimination process that removed its outer layer. According to Li and Zhu (2018), starch is the main carbohydrate present in quinoa (30 to 70 %) and is composed of amylose (27.7%) and amylopectin (7.1%).
Conditions for the agglomeration process
The results of the agglomeration yield for the different treatments are shown in Table 2. The ANOVA test showed a statistically significant effect (P<0.01) for the variables of moisture of the mixture, polysorbate concentration, and their interaction. In general, a higher moisture content had a significant positive effect on the agglomeration yield. Using polysorbate in the solution to wet the flour had a significant negative effect. The ratio of fine/coarse flour and the temperature of the solution did not have any effect on the outcome variable.
Higher agglomeration yields are observed in the quadrants H2-S1 and H2-S2 and under the condition P1 (Tab. 2: 50.61°% ± 0.31 and 48.62% ± 1.25, and 48.18% ± 3.13 and 47.52% ± 0.94, respectively). On average, the use of polysor-bate in the solution slightly decreased the yields for the P1 condition treatments. Therefore, polysorbate was not used in the following stages. Although the variable temperature of the polysorbate solution had no significant effect, the level T2 (e.g., 70°C) was selected. A higher temperature promotes the glass transition of the flour particles, and the resulting partial fusion could strengthen the inner structure of the agglomerates (Cuq et al., 2013). Arzapalo Quinto et al. (2015) showed that the starch gelatinization temperature for three quinoa varieties was between 66 and 69°C; therefore, we expect that the use of a warm solution will allow for a partial gelatinization process to take place. With these considerations, the selected conditions for the quinoa agglomeration process were: a) 70/30 ratio of fine/ coarse flour, b) 40% moisture of the mixture, c) no polysor-bate added to the solution, and d) a temperature of 70°C for the solution used to wet the flour. Under these conditions, the yield of the agglomeration process was 48.62% ± 1.25%. A similar result was reported by Silva Castro (2014) for couscous prepared with durum wheat flour using moisture of 40% in the mixture.
TABLE 2 Agglomeration yield (%) obtained under different treatments.

±σ, n=2.
Different letters among all treatments show significantly different yields. Letter a corresponds to the lowest yield, and letter e to the highest yield.
H1: Moisture of the mixture 35%, H2: Moisture of the mixture 40%.
T1: Polysorbate solution at room temperature (~20°C), T2: Polysorbate solution at 70°C.
P1: Fine/coarse flour: 70/30, P2: Fine/coarse flour 75/25.
S1: Polysorbate solution: 0%, S2: Polysorbate solution: 3%.
ANOVA-test results: """ significant at the 1% level for Moisture of the mixture (H); + + + significant at the 1% level for Polysorbate solution (S).
Conditions for the couscous steam-cooking and drying processes
The results for the output variables for the second and third stages are shown in Table 3. The ANOVA test for WAI showed a statistically significant effect (P<0.05) for the variable drying temperature and the interaction drying time-drying temperature.
According to Debbouz and Donelly (1996) and Giovanelli et al. (2023), a high WAI is a good quality factor for raw couscous, because it is an indicator of its ability to absorb water during cooking and to maintain its firmness, while WSI is a negative attribute because it indicates the amount of soluble materials leached out during cooking, affecting its stickiness. Water absorption is the result of the interaction of water with protein, due to its hydrophilic properties, therefore these indexes are affected by the couscous protein concentration and its structural characteristics (Butt & Batool, 2010). Laya et al. (2022) indicate that processing methods, such as crushing, grinding and sieving carried out during flour production, have an effect on the flour protein content.
The SP was also significantly influenced by the variable drying temperature (P<0.05). A higher drying temperature (70°C) caused a slight increase in the WAI and SP. Cerón-Fernández et al. (2016) also reported higher WAI for higher extrusion temperatures in a quinoa product. The WSI was significantly influenced by the variable drying time and the interaction drying time-drying temperature (0.05). Based on the WSI data, a longer drying time and a higher drying temperature resulted in a lower WSI. However, when the couscous underwent a shorter drying period at a lower temperature, the WSI decreased.
In summary, the variables that had a significant influence on the functional properties of the quinoa couscous were drying temperature and drying time. The supply of energy to the agglomerates improves their stability through starch gelatinization and the cross-linking of the proteins, which in turn reinforce the product's structure (Cuq et al., 2011).
The multiple response optimization methodology (e.g., maximize the WAI and SP and at the same time minimize the WSI) was further used to determine the range of conditions to be selected for the cooking and drying processes. The surface graph for the multiple response optimization, when the drying time is 70°C, is presented in Figure 1. The possible combinations of the best conditions (cooking time-drying time) that can be used to obtain the highest desirability are in the blue surface area of the graph. With the drying temperature fixed at 70°C, the highest desirability is observed for a cooking time of at least 27 min (if the drying time is 120 min) or for a drying time of at least 106 min (if the cooking time is 30 min). The overall highest desirability is observed for a cooking time of 30 min and a drying time of 120 min.
TABLE 3 Water Absorption Index (WAI), Swelling Power (SP), and Water Solubility Index (WSI) for different steam-cooking and drying conditions of quinoa agglomerates.
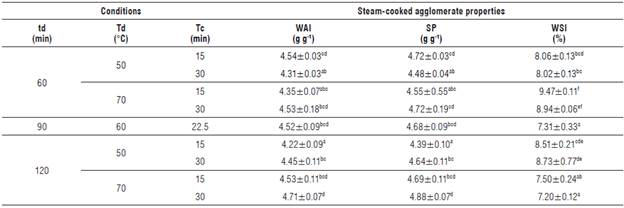
x±a, n=2.
Different letters in each column show a significant difference for the analyzed property. Letter a corresponds to the lowest index observed, and letter d (WAI and SP) or de (WSI) to the highest index.
Tc: cooking time (min); td: drying time (min); Td: drying temperature (0C).
Functional, chemical and sensorial properties of quinoa vs. wheat couscous
Functional and physical properties
A comparison between the results for the functional properties (e.g., WAI, SP, and WSI) for the quinoa and commercial wheat couscous is shown in Table 4. A high WAI is considered a desired quality attribute because high-water absorption improves the firmness of the couscous grain (Debbouz & Donnelly, 1996). The values of WAI for the quinoa (4.72 ± 0.06) and wheat couscous (5.04 ± 0.17) are not significantly different, and both are higher than the minimum WAI required reported by Coskun (2013). The SP of wheat couscous (5.23 ± 0.16) is 7% higher than that of quinoa couscous (4.88 ± 0.07). This result is in line with the existing literature, which reports that products with a higher gluten content have a higher SP than those with lower gluten content (Debbouz & Donnelly, 1996).
Regarding the WSI, the value for the quinoa couscous (7.20% ± 0.12) is twice as high as that for the wheat couscous (3.53% ± 0.28), which implies that the structure of the first sample is less stable. According to Hafsa et al. (2015), partial leaching of starch grains out of the amy-lose chains can happen during the gelatinization process. These starch grains are dissolved in water which increases the WSI. Aboubacar and Hamaker (1999) report a WSI of 6.2% for sorghum (Sorghum bicolor L. Moench) couscous, which is also significantly higher than the WSI reported for wheat couscous. WSI was acceptable, as it normally ranges between 4 and 16% (Giovanelli et al., 2023). Finally, Cankurtaran and Bilgiçli (2021) report that the use of pseu-docereal flour (e.g., amaranth, buckwheat and quinoa) in pasta-like products, causes an increase in their WSI because of the weak protein-starch matrix.
TABLE 4 Functional and physical properties and chemical characterlzation of quinoa couscous and wheat commercial couscous.
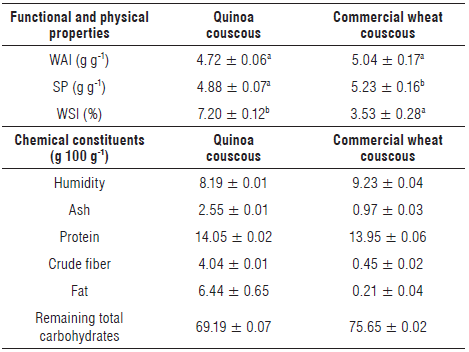
x±σ, n=2.
Figure 2 shows the micrograph from our quinoa couscous sample. The granule is spherical and as seen in the graph, flour particles appear to be strongly joined together and show irregularities and pores on their surface. Additionally, smooth areas also appear on the surface, which are a product of partial fusion of flour particles that have been gelatinized. Partial starch gelatinization occurs during agglomeration and cooking processes (Hafsa et al., 2015). The quinoa granule structure is similar to the one found by Hafsa et al. (2015) for wheat couscous, but in their study the wheat granule appeared slightly more elongated and formed by smaller flour particles.
Chemical properties
The results for the proximal analysis of both samples are presented in Table 4. The moisture in both samples is lower than the maximum value of 13.5% set in the Couscous Codex for this product type (FAO & OMS, 2007). The ash content in the quinoa couscous (2.55% ± 0.01) is 2.5 times higher than in the wheat product (0.97% ± 0.03). According to the Couscous Codex, the maximum ash content in wheat couscous should be 1.1% (FAO & OMS, 2007). This value was set to avoid any adulteration of the product, given that the wheat's maximum ash content is 1.3% (FAO & OMS, 2007). However, the ash content in the quinoa flour used as raw material (2.62%) is higher than the ash in wheat flour, thus, the higher amount of ash in quinoa couscous. The ash content is related to the minerals in the sample. Koziol (1992) mentions that quinoa has 2.9 times more calcium than wheat, which increases its nutritional value.
There is no significant difference in the amount of protein in both samples when a t-student test for mean differences was applied (P value=0.258). However, in terms of protein quality, quinoa flour has more lysine (7.4%) and histi-dine (3.9%) than reported in wheat (e.g., lysine 3.6% and histidine 2.4%) (Abugoch James, 2009). Regarding crude fiber and fat, the amount found in quinoa couscous is 9 and 30 times higher respectively than that in the wheat couscous sample. The higher percentage of fiber in quinoa couscous could bring health benefits by improving bowel movements (Sánchez Almaraz et al., 2015). According to Repo-Carrasco et al. (2003), 87% of the fatty acids found in quinoa are unsaturated, with a high amount of Omega 3, 6, and 9, which play an essential role in maintaining cellular membrane functions and are, therefore, desirable in human nutrition.
Additionally, the carbohydrate content of quinoa couscous is lower than that of wheat couscous. According to Hernández Rodríguez (2015), quinoa carbohydrates have a low glycemic index and are therefore suitable for people suffering from diabetes mellitus.
Sensory properties
Fewer panelists chose quinoa couscous as their favorite when evaluating the following parameters: color, flavor, aroma, and texture. In detail, only 25% of the panelists preferred the color and texture of the quinoa couscous and 33.3% preferred its flavor over the wheat couscous. Some panelists even mentioned the presence of off-flavors and aromas in the quinoa product. This points in the direction of the possible need of additional ingredients or additives to improve the flavor and aroma of the product. Nevertheless, 42% of the panelists did choose quinoa couscous as their overallfavorite product (42% for quinoa vs. 58% for wheat), probably because they related it with a higher nutritional quality. Care has to be taken when looking at these results, given that the difference in the panelist choices is not statistically significant. In a future study, more panelists should be added to the pair comparison test. Cankurtaran and Bilgiçli (2021) report that a highly acceptable couscous sample was obtained when 25% of the wheat flour was replaced with quinoa flour and that the use of an increasing quantity of quinoa decreased the overall acceptability and appearance of the product.
Conclusions
When using quinoa (Chenopodium quinoa Willd.) for the preparation of couscous, the highest yield in the agglomeration process was achieved under the following conditions: ratio of fine/coarse quinoa flour of70/30, a moisture of the mixture of 40%, no bidding agent (polysorbate), and a 70°C water temperature. For the second and third process stages, the best conditions to obtain a stable agglomerated sample were: a steam-cooking time of 30 min, a drying time of 120 min and a temperature in the drying process of 70°C.
The obtained quinoa couscous showed acceptable values for WAI, SP, and WSI. Compared to a commercial wheat couscous sample, the WAI and SP were similar, but the WSI was approximately double. Regarding the chemical properties, the protein amount of the quinoa and wheat couscous samples were similar. However, quinoa does not contain gluten; therefore, the new product could be consumed by gluten-intolerant people. Additionally, the amount of ash, fat, and crude fiber reported for the quinoa product was higher. Finally, quinoa couscous scored lower on the stated preferences of a sensory panel, yet 42% of the panelists did choose quinoa couscous as their favorite product. This result could improve if, in a future study, additional ingredients were added to improve the flavor of the product.