I. Introduction
Manganese oxides type perovskite are of scientific interest due to their potential applications in fuel cells and information storage, and to their diverse advantages, such as miniaturization, long life, and low costs [1-7]. La0.7Sr0.3MnO3 (LSMO) is a compound that, given its characteristics, is a good candidate to be used in field magnetic sensors, non-volatile memories, oxygen sensors, and catalysts in the oxidation of light hydrocarbons [7]. LSMO belongs to the family called perovskites, with formula ABO3, where the cation A occupies the cube's central position; the cations B, the vertices; and the oxygens, the edges' middle position. Regarding the oxygen, cation A presents dodecahedral coordination, whereas cation B presents octahedral coordination [8,9].
Two of the most common methods for synthesizing manganese oxides type LMSO are the ceramic method, and the polymeric complex (Pechini) method, which was developed as a variation of the sol-gel process. The ceramic method, also known as solid state reaction, involves high temperatures, long reaction times, and the synthesis of heterogeneous products. In contrast, the Pechini method allows to prepare highly complex and homogeneous pure materials, and to control the stoichiometry and the possibility of obtaining fibers, powders, and thin films of nanometric size [10] that are the focus of this research.
The most used methods to obtain thin films of nanometric size are physical vapor deposition and chemical deposition. Physical vapor deposition comprises conventional evaporation, reactive evaporation, electron beam evaporation, and sputter deposition. Many of these methods required specialized equipment and high consumption of materials and energy, consequently increasing production costs. On the other hand, among the chemical methods, the spin coating technique has been widely used for manganites (e.g., LSMO) thin film deposition; this technique, in contrast to the physical deposition methods, allows to obtain films with simple and low cost equipment [11].
Spin coating deposition involves four stages: deposition, spin-up, spin-off, and evaporation. The three first stages take place sequentially, while the fourth stage occurs during the entire preparation process. Initially, during the deposition stage, liquid in excess is poured over the surface of a rotating substrate. During the spin-up stage, the liquid flows radially driven by the centrifugal force that is caused by the acceleration and angular speeds applied to the substrate. During the spin-off stage, the liquid that reaches the substrate edge is eliminated in drops as the film thins down. The spin coating deposition ends with the solvent evaporation, that, as previously mentioned, occurs at all times during the film formation process, caused by the spin coater high spinning speeds; however, the final evaporation is carried out, usually, by means of thermic treatment [12].
This paper studies the applicability of the spin coating technique in obtaining manganite La0.7Sr0.3MnO3 thin films, previously synthesized using the Pechini method. Multilayers of this system were deposited over strontium titanate substrates. Structural characterization was achieved by means of X-ray diffraction (XRD); and morphological characterization by means of electron microscopy (SEM). SEM allowed to confirm the formation of uniform and unbroken films, with thickness in the nanoscale. Film thickness was calculated using the envelope method from the transmission spectrum according to [13], in which the envelops constructed around the transmission maxima (TM) and transmission minima (Tm) are used to calculate the refractive index (n) for each layer, using equation (1):
Where S is the substrate's refractive index (strontium titanate: 2.37) [14]. If at two adjacent interference extremes of wavelength h and k the refractive indexes are n 1 and n2, from the conditions of maxima and minima interference, it is possible to find that film thickness is:
With M=1 for two adjacent minima or maxima, and M=1/2 for one adjacent minimum and maximum.
II. Materials and methods
Perovskite in the system La0.7Sr0.3MnO3 was synthesized by means of the Pechini method. Initially, citric acid (Carlo Erba, 99.5 %) and ethylene glycol (Carlo Erba, 99.5 %) were mixed, at 80 °C in reflux for 30 minutes. Subsequently, the precursors La(NO3)3.6H2O (Alfa Aesar, 99 %), Sr(NO3)2 (Sigma Aldrich, 99 %), and Mn(NO3)2.4H2O (Merck, 98.5 %) were added in stoichiometric amounts, constantly stirring, and keeping the temperature at 80 °C for 20 more minutes; after completing this time, the mixture was heated to 150 °C in a muffle furnace until a viscous gel was obtained [7].
Deposition of precursor solution was achieved by means of the spin coating technique, using the spin coater equipment [15]. Previously, the MTI strontium titanate substrates (5 x 5 x 0.5 mm), with orientation 110, and lattice constant a = 3.905 Ǻ [13], were cleaned following four stages: a) 5-minute immersion in NaOH (Carlo Erba, 20 %) followed by a rinsed out with water; b) 4-minute immersion in acetone (Mallinckrodt, 99 %); c) 3-minute immersion in isopropyl alcohol (Merck, 99,8 %), and d) 30-minute drying at 60 °C in a muffle furnace [16].
Over the clean substrate, which was fixed to a rotating plate, 0.2 µL of the LSMO viscous gel were added. Then, the system was rotated at 3000 rpm for 60 seconds, evaporating the excess of liquid, and thus, the film gelification was achieved. Subsequently, to partially eliminate volatile compounds (i.e., solvent and organic species), the layer was dried in a muffle furnace at 80 °C for 30 hours, and then, at 350 °C for 5 minutes. A last calcination, at 650 °C for 6 hours, assured the perovskite formation. The same procedure was followed for multilayers (2, 4, and 6 layers)
The crystal structure of the LSMO films was studied with X-ray diffraction (XRD), in a double circle diffractometer multipurpose X-Pert-Pro PANanalytical, with the following characteristics: tube with copper anode (wavelength K(=1.54060 □), standard resolution goniometer that has the geometry ((-() to measure symmetric and asymmetric configurations, as well as thin films with minimum step width of 0.001° and maximum of 1.27°. The La0.7Sr0.3MnO3 multilayers' surface morphology was analyzed with scanning electron microscopy (SEM), using a JEOL JSM-5910LV microscope operating a 7 kV. Chemical composition of the monolayer was determined by means of Energy Dispersive X-Ray Spectroscopy (EDS). Finally, to measure thickness with the envelop method, thin film transmission spectra for 1, 2, 4, and 6 layers in the 400 nm to 1100 nm region were recorded using a Genesys 10S UV-VIS spectrometer.
III. Results and discussion
The diffraction pattern of the LSMO sample covering the strontium titanate substrate (Fig. 1) was qualitatively analyzed by comparing the observed profile with the diffraction profiles reported in the PDF2.ICDD (International Centre for Diffraction Data) database, following [17]. To treat the data, the following steps were completed: smoothing, background noise removal, K(2 elimination, and peak location through the POWDERX software. Indexing was carried out with the software Dicvo104.
The Rietveld method was used to refine the data, using as models the cubic structure of La0.4Sr0.6MnO2.6 (structure type Ag3SI), with card N° 419753, and the rhombohedral structure of La0.7Sr0.3MnO3, with card N° 153631 from the ICSD (Inorganic Crystal Structure Database), with adjustments to the Gaussian and Lorentzian functions. The refining parameters were X2=2.14, Rwp=11.31, and Rexp=3.93. Using the commercial software RIQAS, and the structure of La0.4Sr0.6MnO2.6 (structure type Ag3SI), with card N° 419753 from the ICSD, as model, a better adjustment between observed/calculated peaks for the cubic system, was achieved.
Positions of the diffraction maxima allowed to assign the Miller's indexes, applying the dichotomy criterion in the specialized software Dicvol04. Only one solution was obtained in the cubic system, with a lattice constant a=3.8653 ± 0.066 Ǻ.
The diffractograms of thin films, which are shown according to the number of layers, show that there is only one phase with cubic crystal structure, and that lines' intensity increase with the number of layers (2, 4, and 6) (Fig. 2); this is due to a larger quantity of deposited material, and therefore, larger number of planes to diffract.
Figure 3(a) shows a SEM image of an area of a LSMO bilayer deposited over strontium titanate substrate using the spin coating technique. The LSMO bilayer shows good morphological characteristics that are comparable with those reported for films deposited using more sophisticated techniques, such as pulse laser deposition [18]. The analyzed film surface does not show a strong granular character, which indicates the formation of a dense surface. This is due to the influence of the inorganic precursors on the LSMO film structure, and to the evaporation and condensation relative speeds during deposition because they control the pore volume and size, and the final film superficial area [19]. In addition, figure 3(a) shows small nodules heterogeneously distributed, whose possible porosity resulted from the shear effect during the centrifugation process [19,20]. Such surfaces are desired in multi-layer systems because they can be used as bases to deposit other functional materials, and thus form heterostructures that are, ultimately, the basic components of many electronic devices.
Figure 3(b) shows the EDS spectrum measured over a LSMO monolayer. Some carbon traces were detected in the spectrum, which are, probably, organic reactant residues, or residues of the tape that was used to fix the sample to the microscope stage. It is noteworthy that the carbon content, yet small, could affect some film properties, especially the resistivity [20]. Chemical composition of the studied material (regarding the elements present) obtained by EDS was 24.12 % La, 6.85 % Sr, 14.24 % Mn, and 47.32 % O; from this composition, it was possible to estimate, following the methodology used in [21], the possible composition formula for the synthesized perovskite (La0.72Sr0.32Mn1.05O3,2), which is consistent with the LSMO molar relation.
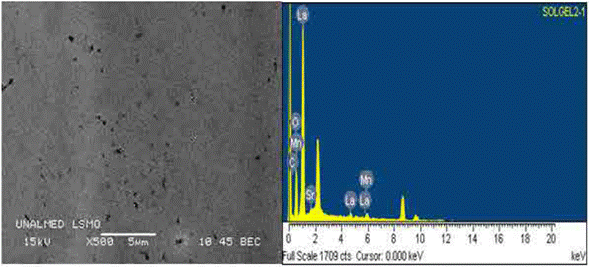
Fig. 3 a) SEM image of a LSMO bilayer over the strontium titanate substrate; b) EDS spectrum of a LSMO bilayer over the strontium titanate substrate.
Figure 4 shows the transmission spectrum of LSMO multilayers, at 3000 rpm, deposited over strontium titanate substrate. Spectra of 4 and 6 layers show a well-defined maxima and minima, while the monolayer's spectrum is less defined.
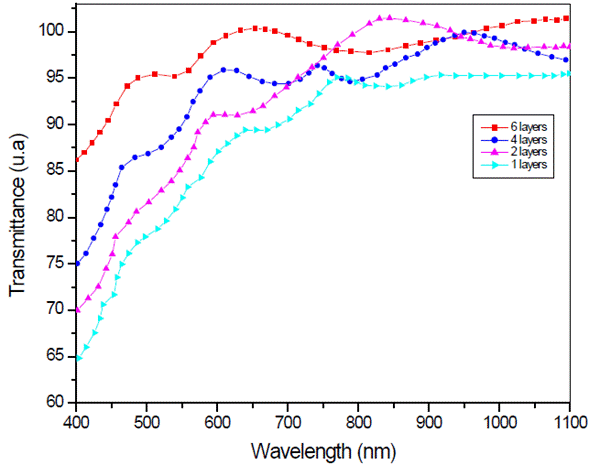
Fig. 4 Optical transmission spectrum of La0.7Sr0.3MnO3 multilayers over strontium titanate substrate.
With the TM and Tm envelopes resulted from the LSMO transmission spectrum, refraction indexes for each multilayer were calculated, using equation (1) in four distinct points of the transmission spectrum (Table 1). Subsequently, the average value was calculated, estimating an error percentage lower than 1 %, and the thickness was estimated by the envelop method, using equation (3) (Table 1).
Table. 1 Thickness by the envelop method for 1, 2, 4, and 6 layers of LSMO over strontium titanate substrate
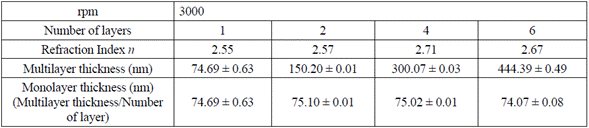
Variations in LSMO monolayer thickness are due to factors such as solution concentration, temperature, and substrate spinning speed (rpm) that varies according to the resin own nature (volatility of the used solvents) and the air surrounding the substrate during the spinning process [11,22,23].
Table 1 also shows that the refraction index (n) for the LSMO 6-layer thin film decreases to 2.67 due to the possible humidity absorption during the spinning and film drying processes.
IV. Conclusions
Using the Pechini polymeric precursor method, and the spin coating technique, it was possible to obtain multilayers in the La0.7Sr0.3MnO3 system with good adherence and homogeneity, and with no fractures. From the obtained Miller's indexes in the DRX analysis, it was estimated that the perovskite La0.7Sr0.3MnO3 presents a simple cubic structure with a lattice constant a = 3.8653 ± 0.066 Ǻ. The SEM images of a LSMO bilayer indicates that its surface is not granular, favoring deposition of other materials. Finally, through the envelop method, the thickness for 2, 4, and 6 LSMO layers was calculated, obtaining thickness lower than 100 nm; this indicates that the Pechini method and the spin coating technique, like other more sophisticated and expensive methods, are useful to obtain films of nanometric size.