INTRODUCTION
Caries is a complex process of multifactorial origin affecting dental tissues. This condition causes the destruction of dentine when the dynamic process of demineralization and constant remineralization is altered by the excess production of acids, in combination with other virulence factors of cariogenic microorganisms.1-3) The first visual manifestation of this disease is an opacity or discoloration of the enamel that, if left untreated, produces cavities and affects other dental tissues.4 Untreated caries can make eating and sleeping difficult, have an impact on children’s growth, and is the main cause of school and work absenteeism.4
Tooth decay is the most widespread chronic disease worldwide, representing a major public health challenge. Current data show that untreated caries in permanent teeth has a global prevalence (averaging all ages) of 40%.5 in Colombia, caries has a prevalence of 55.82% of the population with permanent dentition; however, if early cases are included, this figure increases to 78.97%.6 In consequence, the Ministry of Health and Social Protection of the Government of Colombia recommends developing and promoting different lines of research in oral health based on the country’s realities.6
Despite technological advances, conventional clinical examination remains the most widely used and reliable diagnostic method for dental caries.7,8 The oral evaluation procedure is based on tactile exploration with tools and the dentist’s visual perception.3 These highly subjective methods can potentially lead to faults in caries detection, including false positives, and cause patient discomfort. Science and engineering have provided dentistry with new diagnostic tools, such as X-rays, the dye method, the electrical conductance method, and a wide range of optical methods: fiber optic transillumination (FOTI), digital imaging fiber optic transillumination (DIFOTI), and light- or laser induced fluorescence. X-rays alone allow the diagnosis of proximal caries but are not a reliable method for occlusal caries detection.8 Caries-detector dyes help differentiate healthy dentin from decalcified dentin, although several studies have questioned their effectiveness in recent years.9 Electrical conductance methods are based on the concept that measurements in areas with caries are significantly more sensitive than in caries-free zones, mainly in the differentiation of healthy enamel and carious dentin. They have proven to be more effective than radiographic or transillumination methods in the detection of occlusal caries lesions.10,11 But due to the diversity of depths, the mineral content of enamel and the morphology of occlusal surfaces, adequate data interpretations are generally hindered, mistaking areas of healthy enamel with demineralized areas.12,13) The optical methods claim that when a tooth is illuminated, the light can be absorbed by the dental organ, or get dispersed. These two processes can be altered by changes in the external/internal structure of the tooth.8 Transillumination with fiber optics in all its forms is based on the fact that light propagates from a light source to the tooth through optical fibers and must be intense enough to traverse tooth structure. The transillumination method has been recommended to detect proximal and occlusal cavities.14-16 Acquiring and processing images through fiber optic transillumination to increase sensitivity using software is known as digital imaging fiber optic transillumination.8
Light- or laser induced fluorescence is based on the principle that changes induced in the dental structure by the caries process lead to an increase in fluorescence when specific wavelengths are applied.17 This method has been successfully used in the detection of lesions on flat teeth surfaces but its use in occlusal sides has not been sufficiently demonstrated. However, it seems to be very promising.18
Taking into account the state of the art and the recommendation by the Colombian Government, this study sought to examine the behavior of carious and non-carious areas of vital teeth by submitting them to infrared light of 830 nm. It also assesses the effectiveness of this wavelength to diagnose dental caries, with the objective of exploring new perspectives in the creation of more effective detection devices. In finding a behavior to differentiate carious lesions from healthy areas, this study characterized the system used to detect tooth decay. In the suggested system, the infrared light is conducted through a trifurcated optic fiber to reach the teeth, and, thanks to the derived branches, the reflected signal is received and brought to an infrared light sensor.
METHODS
The study sample included 167 vital dental units from human beings aged 18 to 60 years who admitted to a dental examination and had at least one dental caries. Underage individuals and patients in medical treatment were excluded. The test population was the administrative staff of Universidad Popular del Cesar.
This study sought to examine the behavior of carious and non-carious areas by submitting them to infrared light of 830 nm. After finding a behavior that helped differentiate carious lesions from healthy areas, the system was characterized to detect tooth decay. The procedure used an experimental assembly provided with an optic fiber sensor and infrared light with which optical power measurements were acquired from each dental unit. The sampling process was performed on teeth from patients who had previously been diagnosed with cavities by a professional dentist. Each sample was cleaned and dried, trying to maintain that state during measurements, which were performed by a person calibrated in the identification of dental caries and in the handling of optical fibers. The occlusal and buccal areas of the subjects’ complete denture were preliminarily characterized in order to determine the levels of reflected power of the assembly made for a healthy tooth and a decayed tooth, according to their classification and shape. Once these levels of power were established, the hypothesis was tested in five patients. This study complies with the Declaration of Helsinki19 for research on humans and with the terms set forth in Resolution 8430 of the Ministry of Social Protection.20
The experimental assembly is composed of a fiber optic sensor using a Newport/Oriel model 77536 trifurcated fiber which is flexible enough to easily lead infrared light to the teeth. It also consists of a Newport optical table provided with supporting tools to connect an infrared LED (IR LED) with wavelength 830 nm to one of the three branches of the trifurcated fiber. The infrared LED belongs to a Newport Laser Diode-Led Driver Model Nº FK-DRV.21,22 Its two branches are connected by the same method to a Newport Model 1815 Power Meter-C. An 818-SL silicon photodetector was used with a dimmer. The free, unbranched end is used to bring infrared radiation to the teeth as a probe. Figure 1 shows the implemented configuration.
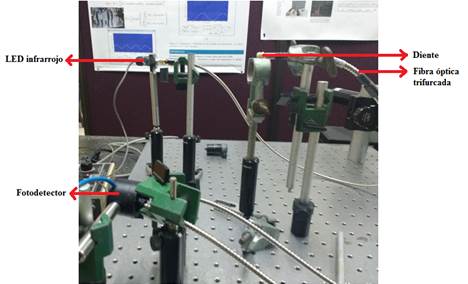
Figure 1 Experimental assembly [Traducción de la figura. De izquierda a derecha: Infrared LED - Photodetector - Tooth - trifurcated optic fiber]
Once in operation, the assembly brings infrared light through the fiber’s assigned branch to the unbranched end impacting the tooth. At the same time, the radiation reflected by the dental unit is received and transported to the branched ends. The branched ends lead the reflected light to the power meter to establish intensity. In order to obtain results with very significant magnitudes, all the tests and detections were performed with the Newport Laser Diode-Led Driver at maximum power. In this article, averaged values were taken for all variables measurements.
Optical and electrical characteristics of the implemented optical fiber sensor
As a fiber optic sensor was implemented, it was necessary to know its operating ranges. The values of the optical power parameters emitted by the IR LED, the power transmitted by the optical fiber, and the current intensity were obtained after selecting the maximum power of the Newport Laser Diode-Led Driver Model Nº FK-DRV as operation current for all tests. Obtaining these variables’ values will help reproduce this experimental system in future studies or verify the achieved results. The fiber optic sensor operation characteristics can be seen in Table 1. Figure 2a shows the process of measuring the power emitted by the IR LED and Figure 2b shows the process of acquiring the magnitude of the infrared power transmitted by the sensor.
Table 1 Optical fiber sensor operation features
Operating optical and electrical features of the fiber optic sensor | |||
---|---|---|---|
Parameter | Value | Unit | |
Operating current | 100.3 | Ma | |
Optical power emitted by the IR LED | 1.814 | Mw | |
Infrared power transmitted by the sensor | 1.386 | Mw | |
Transmitted optical power efficiency | 76.41% | ||
Maximum reflected power | 228 | ΜW | |
Reflected infrared light conduction efficiency | 81.72% |
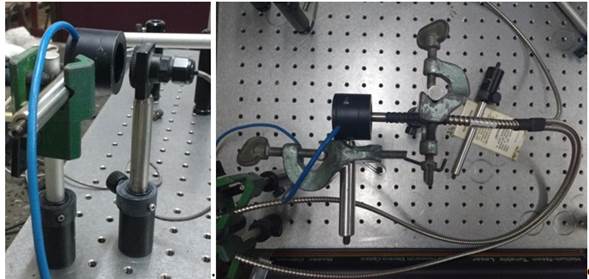
Figure 2 a) Process for measuring the optical power emitted by the IR LED, b) Process for measuring the infrared power transmitted by the optical fiber sensor
In order to determine infrared light conduction efficiency, measurements of power reflected by a mirror with and without the sensor were carried out. The reflected power without the sensor between the IR LED and the power meter was 279 µW (this measurement is shown in Figure 3a). When the sensor was added, in the same operating current a reflected power of 228 µW was obtained (the process is illustrated in Figure 3b). Based on these measurements, the reflected infrared light conduction efficiency, which is also referred to in Table 1, was calculated.
Finally, after measuring the operation characteristics of the fiber optic sensor, the system was tested in live teeth samples to determine any type of behavior that would help establish the presence of caries.
RESULTS AND DISCUSSION
Preliminary test on live teeth and characterization of sensor performance
Sampling was performed only in the vestibular, incisal, and occlusal areas of the teeth of a volunteer patient who previously received a dental caries diagnosis by an oral health professional. In attempting to sample the lingual area, the fiber was at risk of suffering a superflexion that could harm the tongue. Therefore, the lingual area was not sampled. The sampling process is shown in Figure 4a. In addition, full denture sampling helped determine eigenvalues for common elements in the treatment of oral diseases such as amalgams and resins. Bearing in mind that teeth shape would influence the values of powers to be measured, these were stored by the FDI dental notation,23 which would allow the analysis per tooth type. To facilitate understanding of this article, the FDI notation is shown in Figure 4b. In comparing the measurements obtained with the oral diagnosis, caries detection thresholds were established on the occlusal, incisal and vestibular surfaces. The reflected power measurements are shown in Tables 2 and 3.
Table 2 Power in the vestibular area
Vestibular surface | ||||||||||||||||
Upper maxillary | Type of tooth | Molars | Premolars | Canine | Incisors | Canine | Premolars | Molars | ||||||||
Tooth | 18 | 17 | 16 | 15 | 14 | 13 | 12 | 11 | 21 | 22 | 23 | 24 | 25 | 26 | 27 | 28 |
Power (μW) | 20.8 | 21.3 | 18.3 | 17.8 | 19.5 | 22 | 22 | 18.5 | 20.8 | 19.8 | 18.9 | 19.5 | 20.5 | 19.7 | ||
Mandible | Type of tooth | Molars | Premolars | Canine | Incisors | Canine | Premolars | Molars | ||||||||
Tooth | 48 | 47 | 46 | 45 | 44 | 43 | 42 | 41 | 31 | 32 | 33 | 34 | 35 | 36 | 37 | 38 |
Power(μW) | 19.4 | 20 | 19.1 | 19 | 21.4 | 22.4 | 21.4 | 21.3 | 21.8 | 21.4 | 19.9 | 18 | 18 | 19 |
Table 3 Power in the incisal and occlusal areas
Incisal and occlusal surfaces | ||||||||||||||||
Upper maxillary | Type of tooth | Molars | Premolars | Canine | Incisors | Canine | Premolars | Molars | ||||||||
Tooth | 18 | 17 | 16 | 15 | 14 | 13 | 12 | 11 | 21 | 22 | 23 | 24 | 25 | 26 | 27 | 28 |
Power (μW)) | 11.4 | 7.6 | 11.5 | 12 | 10.5 | 8.7 | 8.4 | 9.0 | 8.2 | 10.1 | 12.7 | 12.8 | 11.9 | 8.9 | ||
Mandible | Type of tooth | Molars | Premolars | Canine | Incisors | Canine | Premolars | Molars | ||||||||
Tooth | 48 | 47 | 46 | 45 | 44 | 43 | 42 | 41 | 31 | 32 | 33 | 34 | 35 | 36 | 37 | 38 |
Power (μW)) | 10.7 | 12.1 | 11 | 11.8 | 6.1 | 6.5 | 7.1 | 6.8 | 7.0 | 7.7 | 13 | 11.5 | 10.2 | 10.2 |
The dental diagnosis of the patient yielded the following results: caries in the left upper molar 27, an amalgam in the right upper molar 16, a white resin in the lower right molars 36 and 37, and a reconstruction of the left upper incisor 21. Molars 18, 28, 38 and 48 were not present in the patient. Based on this information, the measurements made with the sensor were analyzed.
The obtained measurements showed that the levels of reflected power vary according to the geometry of the dental unit and the existing area for infrared light exposure. Therefore, the analysis of the results was performed per dental areas and according to teeth typology. The vestibular areas, regardless of tooth type, reflect more uniform levels of infrared power. It should be noted that, because of their size, incisor teeth (dental unit 12, 11, 22, 42, 41, 31 and 32 in Table 2) reflect higher power levels. The same situation was observed in measuring incisal and occlusal areas: the power reflected by the premolars and molars was higher than that reflected by the incisors and canines (see differences in Table 3).
The power levels reflected in healthy incisors of incisal areas are lower than those in occlusal areas of premolars and molars. Similarly, in the incisal area, the upper canines reflect higher levels than the lower canines (see teeth 13, 23, 43 and 33 in Table 3).
It can be seen (Table 3) that dental unit 27, with caries in its occlusal area, reflected 8.9 µW, while the other healthy bicuspids and molars reflected more than 10 µW. This observation demonstrates that the behavior shown by extracted dental units is also shown in living dental units. This behavior can be used to determine the presence of cavities. In short, caries-related areas reflect less infrared power than healthy areas.
Amalgams, because of their physical properties (color), reflect much lower levels than healthy teeth, to the point of coinciding with caries-specific values (see tooth 16 in Table 3). Resins reflect power levels very close to the levels of healthy areas (see molars 36 and 37 in Table 2 with respect to adjacent healthy molars).
Quantitatively, it is evident that, on the vestibular surface, healthy teeth reflect power levels above 17 µW. On the other hand, the levels measured in healthy incisal surface segments show differences per tooth type: the incisors present powers greater than or equal to 6.5 μW, while the canines reflect powers greater than 6 µW. On the occlusal surface, the bicuspids and caries of the molars reflect powers greater than or equal to 11 μW. Therefore, caries thresholds, as shown in Table 4, were determined for the assembled system and used in the additional tests with patients.
Caries detection in volunteers
Given the low number of caries samples in lingual, buccal and interproximal areas, the tests in patients were mostly focused on occlusal areas. The verification of the proposed caries diagnostic thresholdswas performed in 5 additional patients who had been diagnosed with dental caries by their dentists. The 6 patients, including the volunteer of the preliminary test, offered 167 experimental dental units, of which 11 corresponded to carious teeth diagnosed by the visual-tactile method. The fiber optic sensor detected 100% of caries previously diagnosed by dentists. The detections in all samples made in decayed units with their respective measurements are displayed in Table 5. Healthy teeth reflected power levels higher than the suggested thresholds.
Table 5 Caries detection in volunteers.
Sample | Location in dental area | Caries type | Sensed optic power (µW) | Upper threshold of dental caries (µW) | Presence of dental caries |
1 | Buccal | Moderate | 14 | 17 | Positive |
2 | Buccal | Moderate | 11 | 17 | Positive |
3 | Occlusal | Incipient | 10 | 10.9 | Positive |
4 | Occlusal | Incipient | 10.5 | 10.9 | Positive |
5 | Occlusal | Incipient | 10.2 | 10.9 | Positive |
6 | Occlusal | Moderate | 7.6 | 10.9 | Positive |
7 | Occlusal | Moderate | 8 | 10.9 | Positive |
8 | Occlusal | Moderate | 8 | 10.9 | Positive |
9 | Occlusal | Moderate | 9 | 10.9 | Positive |
10 | Occlusal | Moderate | 6 | 10.9 | Positive |
11 | Occlusal | Moderate | 8 | 10.9 | Positive |
During the tests, patients expressed discomfort in their teeth under resins in occlusal areas, which were not detected by the dentist or the system. This raises questions-which must be addressed in further studies-regarding the penetration depth of infrared radiation in teeth.
CONCLUSIONS
This study showed differences between the power levels reflected by carious and non-carious areas, helping establish the presence of dental caries. Although the oral conditions to which teeth are subjected vary from one individual to another, the system and the suggested diagnostic thresholds of caries meet the desired expectations. The approach used proved to be effective and practical, and might help minimize costs. The implemented sensor detected 100% of caries in the occlusal areas where it was tested. Of course, tests for caries diagnostic thresholds that were not tested because they were not present in patients should be the subject of further studies. In addition, this system needs further studies to find methods to differentiate the reflected powers of amalgams, resins and decayed areas. This will lead to accurate detections, avoiding false positives. In the same way, there are pending questions about the depth of penetration of infrared radiation in teeth and the possible induced infrared fluorescence caused by radiating a carious zone with infrared light with wavelength of 830 nm. These phenomena were not analyzed in this work.