1. INTRODUCTION
Emerging and re- emerging diseases pose a continuing challenge for global public health. Within these, respiratory diseases or syndromes caused by coronavirus have recently acquired relevance given the several outbreaks caused by this family of viruses since 2003 (1-3). However, currently the most important is the contagious disease COVID-19 (Corona Virus Infectious Disease 2019), first detected in December of 2019 in the province of Wuhan, China, and declared a pandemic by the World Health Organization (WHO) in March 2020 (4-7). This illness is caused by the infection with the new beta-coronavirus named Severe Acute Respiratory Syndrome Coronavirus 2 (SARS-CoV-2). A positive- sense single-stranded RNA virus with a genome of approximately 30 kb with an untranslated structured region, which appears to be of zoonotic origin since it shares about 96% genetic homology with bat coronavirus and also high genomic homology, close to 80%, with SARS-CoV, another beta-coronavirus described in 2002-2003 also in Asia (2,8).
Symptoms caused by COVID-19 are unspecific, and the clinical presentation encompasses a wide range of manifestations, ranging from asymptomatic infections to severe pneumonia and death. The main symptoms of COVID-19 are fever, tiredness, and dry cough. Some patients may have shortness of breath, chills, muscle pain, loss of taste or smell, vomiting, diarrhea, and/or sore throat (4). Older people and people with pre-existing comorbidities such as high blood pressure (30.7%), heart disease (11.9%), lung disease (6%), cancer, or diabetes (12-14%) are more likely to develop a severe presentation (4). So far, the available vaccines to prevent infection do not reach 100% efficacy, and there is no specific antiviral treatment for SARS-CoV-2 infection; therefore, patient management focuses on symptomatic treatment (9).
This scenario makes clear the need to find new specific pharmacological and therapeutic alternatives against SARS-CoV-2 infection and opens a way to the exploration of a practice historically carried out by communities of Asia, Africa, and Latin America, such as the use of medicinal plants for diseases treatment (10,11).
A medicinal plant is any plant that contains substances that can be used for therapeutic purposes; they can be prepared in different ways, including teas, infusions, vinegar extracts, or alcohol tinctures (12). Medicinal plants are used to treat a wide range of diseases and, in developing countries, represent an alternative to primary health care. It has been reported that approximately 80% of the world population uses medicinal plants for health purposes; It is also estimated that at least 50% of modern medicines contain one or more active ingredients originating from a plant (12).
Medicinal plants historically used in traditional Chinese medicine were utilized in about 91.5% of COVID-19 cases in China, showing encouraging results in improving the symptoms and reducing deterioration, mortality, and recurrence rates (13). In Colombia, medicinal plants play an important role in Afro-Colombian, mestizo, and indigenous territories treating different conditions, including COVID -19 (14,15). Plants as Gliricidia sepium, Piper tuberculatum, Solanum arboreum, Moringa oleifera, and Bocconia frutescens have been used within indigenous people given their multiple properties. The antimicrobial properties of G. sepium has been reported previously (16,17). More recently, Cordoba-Tovar et al. reported that this plant is one of the species with the highest use against COVID-19 by western Colombian people (18). P. tuberculatum and, S. arboreum are recognized and used mainly in the Colombian Pacific region for their antimicrobial, analgesic, and antipyretic properties against several diseases (14,19-22), including COVID-19 despite the absence of scientific evidence. The potential of M. oleifera against SARS-CoV-2 has been explored recently by in silico approaches, finding flavonoid present on this plant showed good binding affinity, stable protein-ligand complexes, high binding energy, and similar binding poses in comparison with known SARS-CoV-2 Mpro inhibitor (23-25); its effect on COVID-19 associated coinfections has been explored as well (26). S. magellanicum is one of the most globally widespread mosses, traditionally used in Europe as an antiseptic and wound sterilization and was widely used during the first world war. Nowadays, traditional medicine uses this moss-like antibacterial and antiviral (27).
In this work, we set out to evaluate the antiviral activity against SARS-CoV-2 of six extracts produced from medicinal plants frequently used within the traditional medicine of the Pacific and other regions of Colombia to find possible therapeutic alternatives to face COVID-19.
2. MATERIALS AND METHODS
2.1 Cells and virus
Cercopithecus aethiops kidney cell line, Vero E6 was grown in Dulbecco’s Modified Eagle Medium (DMEM, Sigma-Aldrich) supplemented with 2 % heat-inactivated fetal bovine serum (FBS) (Gibco), 1 % penicillin-streptomycin (Sigma-Aldrich), and 2 mM L-glutamine (Sigma-Aldrich) at 37 °C with 5 % CO2. Vero E6 cell line was donated by Instituto Nacional de Salud (INS) (Bogotá, Colombia). Infections were carried out with a SARS-CoV-2 viral stock produced from a Colombian isolate an ancestral D614G strain (ID accession: EPI_ISL_536399), (hCoV-19/Colombia/ ANT-UdeA-200325-01/2020) (28).
2.2 Colombian plants identification and registration
Plant material and botanical samples were collected from some species used in traditional medicine by afro, indigenous, and farmers from departments of Chocó and Antioquia, to treat COVID-19 symptoms. The vouchers were deposited in the herbarium of the Universidad de Antioquia (HUA) with the collection numbers described in Table 1, Figure 1.
Table 1 List of plant species included in this study
Species | Common name | Family | Voucher |
---|---|---|---|
Gliricidia sepium (Jacq.) Kunth ex Walp. | Matarratón | Fabaceae | Alzate 5404 |
Piper tuberculatum Jacq. | Pipilongo or Santamaria | Piperaceae | Alzate 5405 |
Solanum arboreum Dunal | Sauco de monte or Sauco macho | Solanaceae | Alzate 5406 |
Moringa oleifera Lam. | Moringa or Ben | Moringaceae | Alzate 5464 |
Bocconia frutescens L. | Trompeto | Papaveraceae | Idarraga 3789 |
Sphagnum magellanicum Brid. | Esfagno | Sphagnaceae | Alzate 5463a |
The herbal drugs were selected, and the extracts were prepared following the instructions used by the communities to obtain the effect against COVID-19 symptoms.
2.3 Plant extracts preparation
Each fresh plant was extracted with 50 mL solvent (reagent grade) or distilled water at room temperature (20 °C). The samples were macerated by 10 minutes and filtered using 595 Whatman® cellulose filters, exhibiting particle retention levels of 7-12 µm. Then, the filtrates were transferred into glass bottles. The concentrations of the extracts were around 120 mg/mL. All extracts were stored at 4 °C until use. More detailed information about extract preparation can be found in Table 2.
Table 2 Plant species, herbal drugs and solvents used for the extract preparations.
Species | Herbal drug | Weight (g) | Solvent | Volume (mL) |
---|---|---|---|---|
Moringa oleifera | Leaf | 6.00662 | Water | 50.0 |
Bocconia frutescens | Leaft and stem | 6.04500 | HCl 5% | 50.0 |
Piper tuberculatum | Inflorescence | 6.10450 | Water | 50.0 |
Gliricidia sepium | Leaf | 6.27841 | Water | 50.0 |
Solanum arboreum | Leaf | 6.22018 | Water | 50.0 |
Sphagnum magellanicum | Whole plant | 6.00213 | HCl 5% | 50.0 |
2.4 Cytotoxicity assay
The cytotoxicity of plant extracts was evaluated on Vero E6 cells by an MTT (3-[4,5-dimethylthiazol-2- yl]-2,5 diphenyl tetrazolium bromide) assay. Briefly, Vero E6 cells were seeded in 96-well plates at a cell density of 1.0x104 cells/well in DMEM supplemented with 2 % FBS. Then, the plates were incubated for 24 h at 37 ºC with 5 % CO2. After incubation, 150 µL/well of plant extracts dilutions were added to each well in quadruplicate and incubated for 48 h, at 37 ºC with 5 % CO2. After incubation, the supernatants were removed, and cells were washed twice with Phosphate Buffered Saline (PBS) (Lonza) before adding 30 µL/well of MTT (2 mg/mL). Following MTT addition, plates were incubated for 2 h at 37 °C, with 5 % CO2, protected from light. Finally, formazan crystals were dissolved by adding 100 µL/well of pure DMSO (29).
Plates were read at 570 nm using a Multiskan GO spectrophotometer (Thermo-Scientific). The average absorbance of untreated cells was used as a viability control. The cell viability of each treated well was calculated based on the viability control. Concentrations with cell viability >85 % after treatment was considered non-cytotoxic. For the MTT assay, two independent experiments were performed (n=8).
2.5 Evaluation of the antiviral activity against SARS-CoV-2
The antiviral activity of plant extracts was evaluated by a pre-post-infection treatment strategy, where the cells are treated after and before 1 h of virus adsorption. Briefly, Vero E6 cells were seeded in 96-well plates (1.0 × 104 cells/well) and incubated for 24 h, at 37 ºC with 5 % CO2. Then, plants extract dilutions selected based on cytotoxic assay were prepared and added to the cell monolayers (50 µL/ well) for 1 h. After pre-treatment, the extract was removed, and the virus inoculum was added at a multiplicity of infection (MOI) of 0.01 in 50 µL/well of DMEM supplemented with 2 % FBS. After 1 h of virus adsorption at 37 °C, the inoculum was removed and replaced by 150 µL/well of the same pre-treatment dilutions. Finally, the plates were incubated for 48 h at 37 °C with a 5 % CO2 atmosphere. After 48h of incubation, the supernatants were harvested and stored at −80 °C for viral titration by plaque assay. Chloroquine at 100 µg/mL was included as positive inhibition control. The supernatant of infected cells without treatment was used as uninfected control. Two independent experiments with four replicate each were performed (n=8).
2.6 SARS-CoV-2 quantification by Plaque assay
The antiviral activity of Colombian plant extracts was determined by reducing the infectious particles of SARS-CoV-2 in supernatants of treated cells by plaque assay. Briefly, 1.2 x 105 Vero E6 cells/well were seeded in 24-well plates for 24 h, at 37 °C, with 5 % CO2. Subsequently, tenfold serial dilutions of the supernatants obtained from the antiviral assay (200µL/well) were added to cell monolayers and incubated for 1h at 37 °C with 5 % CO2. Then, the viral inoculum was removed and replaced by 1 mL of semi-solid medium (1.5 % Carboxymethyl- cellulose in DMEM 1X with 2 % FBS and 1 % Penicillin-Streptomycin). Cells were incubated for 3 days at 37 °C, with 5 % CO2. Finally, monolayers were washed twice with PBS, fixed/stained with 4% Formaldehyde/ 1 % Crystal violet solution, and viral plaques were counted.
The difference between the viral titer after treatment and the untreated control was expressed as inhibition percentage. Two independent experiments were performed (n=4) with two replicates each.
2.7 Statistical analysis
All data were analyzed with GraphPad Prism (La Jolla, CA, USA). Data were presented as mean ± SEM. Statistical differences were evaluated by Student’s t-test or Mann-Whitney U test based on Shapiro Wilk normality test. A p-value ≤ 0.05 was considered significant, with * p≤ 0.05 and ** p≤ 0.01.
3. RESULTS
3.1 Cytotoxicity of plant extracts on VeroE6.
For the cytotoxic assay, S. arboreum, G. sepium and P. tuberculatum extracts were evaluated from 10 mg/mL to 2 mg/mL using double serial dilutions. After 48 h treatment, Vero E6 monolayers exhibited viabilities lower than 85 % for S. arboreum and P. tuberculatum extracts at 10 mg/mL and 8 mg/mL; while at 6 mg/mL, these plant extracts showed viabilities of 89.1 % and 88.1 %, respectively. An IC50 of 28.8 mg/mL and 12.23 mg/mL were calculated for these extracts, respectively. G. sepium extract treatment showed viabilities higher than 85 % at all evaluated concentrations (IC50 was not calculated).
Sphagnum magellanicum, B. frutescens and M. oleifera extracts were cytotoxic at 2 mg/mL (data not shown). For this reason, these extracts were evaluated at lower concentrations. Vero E6 monolayers exhibit a viability of 84.86 % at 1 mg/mL with S. magellanicum treatment while 93.1 % at 0.8 mg/mL, S. magellanicum extract shown an IC50 of 1.2 mg/mL. For B. frutescens extract, viabilities of 28.6 % and 80.7 % were observed at 0.8 mg/mL and 0.6 mg/mL, respectively, while 88.4 % at 0.4 mg/mL to an IC50 of 0.71 mg/mL. Finally, M. oleifera extract exhibited viabilities around 99.2 % at 0.6 mg/mL, while 75.2 % and 48.7 % at 0.8 mg/mL and 1 mg/ mL respectively for an IC50 of 0.95 mg/mL.
Based on the results of the cytotoxic assay, S. arboreum and P. tuberculatum extracts were evaluated from 6 mg/mL and G. sepium from 10 mg/mL for antiviral assay. On the other hand, S. magellanicum, M. oleifera and B. frutescens extracts were evaluated from 0.8 mg/mL, 0.6 mg/mL, and 0.4 mg/mL, respectively.
3.2 G. sepium and P. tuberculatum extracts exhibit antiviral activity against SARS-CoV-2.
After 48 h of pre-post treatment strategy, G. sepium and P. tuberculatum extracts treatment resulted in a reduction of SARS-CoV-2 viral titer on Vero E6 supernatants compared to untreated control. G. sepium treatment exhibited a reduction of 75.6 % (p=0.009), 56.88 % (p=0.009), 62.50 % (p=0.009), and 40.0 % (p=0.009) at 10 mg/mL, 8 mg/mL, 6 mg/ mL, and 4 mg/mL (Figure 3). The supernatant of P. tuberculatum treated cells exhibited a reduction of 33.3 % (p=0.004) in SARS-CoV-2 titer compared to the supernatant of untreated cells at 6 mg/mL.
Solanum arboreum, S. magellanicum and B. frutescens extracts treatment showed no antiviral activity at any evaluated concentration with a pre- post treatment strategy; instead an increase in viral titer was observed at 6 mg/mL for S. arboreum, 0.6 and 0.4 mg/mL for S. magellanicum, and from 0.2 to 0.05 mg/mL for B. frutescens. No differences in viral titer were observed after M. oleifera treatment after 48 h at any evaluated concentration (Figure 3).
Chloroquine at 100 µM was used as inhibition control showing a reduction of 100 % in SARS-CoV-2 viral titer (Figure 3).
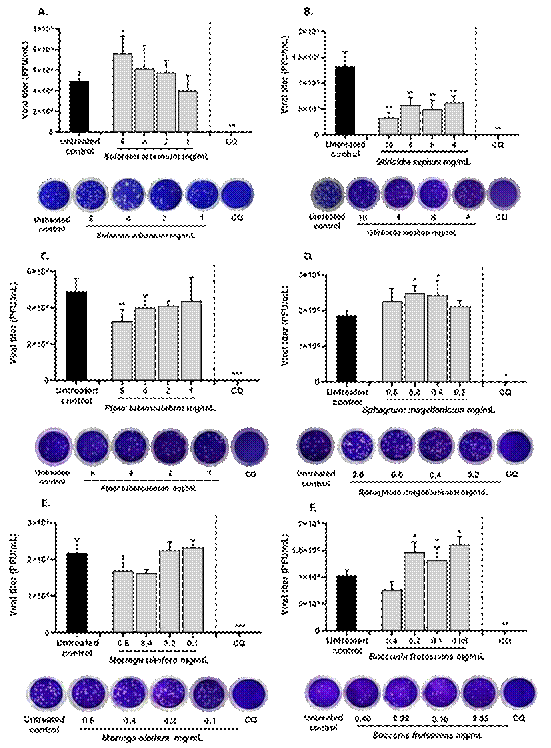
Figure 3 Antiviral activity of plant extracts against SARS-CoV-2 on Vero E6. The graph shows the titers of SARS-CoV-2 in supernatants of VeroE6 monolayers after 48 h treatment with plant extracts together with a representative figure of the plaque assay on Vero E6 after five days of incubation. Data are shown as mean±SEM. Two different experiments were done with two replicas each (n=4).
4. DISCUSSION
This article evaluates the antiviral activity of six extracts prepared from six plants used for indigenous people from the Colombian pacific and Andean regions to treat SARS-CoV-2 infection and COVID-19 related symptoms to bring light about the efficacy of these traditional treatments widely used in our territory.
Our results showed that G. sepium, has antiviral effect against SARS-CoV-2 in vitro. The biological activity of G. sepium against several organisms has been widely studied. Leaf extracts of this plant showed larvicidal activities against Anopheles stephansi, Aedes aegypti, and Culex quinquefasciatus (30). Leaves of Gliricidia sepium also have antimicrobial activity against bacteria and nematodes (31). On the other hand, G. sepium antiviral activity has been slightly explored. In 2010 Hernández Ramírez et al. reported 100 % remission of symptoms and normal leukocyte and platelet number in a small group of patients with dengue virus after administration of the leaf extract (32). However, there are no other reports of G. sepium activity against viruses. Based on our results, G. sepium extract reduces SARS- CoV-2 viral titer at all evaluated concentrations after 48 h treatment.
Given the wide range of G. sepium biological activity studies against different microorganisms, the presence of compounds with biological activity has been previously reported. The glycosides, alkaloids, saponins, and flavonoids present in G. sepium extracts have been proposed as responsible for the antibacterial, antioxidant and anti-inflammatory properties (31). Among those compounds, saponins and flavonoids have inhibitory potential against SARS-CoV-2 demonstrated by in silico analysis (33,34).
In 2021, Fakhar et al. analyzed in silico the interaction of several anthocyanin derivatives with SARS-CoV-2 Mpro (Main protein), obtaining better docking score (DS) values (from -9.58 kcal/mol to -12.37 kcal/mol) than N3-I, a native inhibitor of Mpro (-9.47 kcal/mol) (33). Similar results were reported by Falade et al. in which saponins obtained DS around -8.0 kcal/mol improving the values for N3-I (−7.3 kcal/mol) and with better pharmacokinetics and bioavailability compared to Remdesivir, the only approved drug to COVID-19 treatment (34,35).
The potential of plants belonging to the Piper genus as a source of new natural and bioactive compounds has been reviewed by several authors (36, 37). The occurrence of alkamides, piperamides, kavalactones, and benzoic acid derivatives has been reported for plants of the Piper genus (36,38). Likewise, several biological activities, including biocidal activity against bacteria like Mycobacterium tuberculosis, activities against various protozoa like Leishmania, Plasmodium, and Trypanosoma (39,40), and antiviral activity against West Nile Virus and Human immuno deficienty virus resistant strains (41,42). Among those compounds, alkamides and piperamides containing amides and aromatic groups similar to remdesivir, an approved drug for COVID-19, can exert a potential inhibition of SARS- CoV-2, given the interaction of these structures with Mpro, RdRp (RNA dependent RNA polymerase), and ACE2 (angiotensin converting enzyme) (43-45).
The interaction of Piper compounds with SARS- CoV-2 viral proteins has been evaluated in silico (35). Gutierrez-Villagomez et al. reported that alkamides and dimeric piperamides from Piper species exhibited a high binding affinity for two SARS- CoV-2 proteins (Mpro and RdRp). The complex formed between pipercyclobutanamide B and Mpro showed a DS of −7.83 kcal/mol, like the values obtained by N3-I (−7.35 kcal/mol) (35). Together with these docking results, the ADME profile indicated that dimeric piperamides have drug-likeness potential (35). This evidence and the fact that P. tuberculatum treatment exhibited a reduction of 33.33 % in SARS-CoV-2 viral titer in vitro suggest that P. tuberculatum and other Piper species can represent a target for anti-SARS-CoV-2 drug research.
The results obtained for S. arboreum, S. magellanicum, and B. frutescens treatment indicates an increase of viral titer; this result could be due to compounds present in the extract that at those concentrations can promote cell proliferation or regulation of metabolic pathways affecting the expression of viral receptors or proteins synthesis, that is the case of S. magellanicum extract which has been reported to be rich in amino acids affecting the protein synthesis; however more studies are needed to identify particular molecules involved in this response. (46,47)
The evidence presented in this article shows the potential of the ethnobotanical approach in the search for natural compounds with biological activities, optimizing the search of natural candidates for antiviral screening. We also previous evidence of its potential as anti-SARS-CoV-2 alternatives, exhibiting Mpro as an important target to consider for further research. However, further studies are necessary to identify the specific molecules with antiviral activity.
CONCLUSION
Gliricidia sepium and P. tuberculatum have been widely used by Colombian indigenous and afro people to treat different diseases. Previous studies have shown their antiviral effect against other RNA viruses; besides, recently in silico approaches have shown their potential as antiviral agents against SARS-CoV-2. This article presents evidence of the antiviral effect of G. sepium and P. tuberculatum extracts reducing in vitro the titer of SARS-CoV-2 after 48 h treatment. This evidence demonstrates the potential of these plants as a therapeutic alternative against SARS-CoV-2 infection and the importance of future studies to elucidate molecules with antiviral activity, mechanisms of action, and their potential use for the treatment of COVID-19.