1 Introduction
Monoterpenes are compounds with 10 carbon atoms, their structure consist of 2 units of 5 branched carbon atoms of isoprene type and can be cyclic or acyclic [1]. Limonene is a cyclic monoterpene, which is found in the essential oils of citrus fruit peels, such as: tangerine, lemon, orange, among others. Although it is also found in some essential oils of some herbs such as citronella and mint [2]. Apart to be the principal component in citric peels, it is founded with a high optically purity with 90%. For example, from the deterpenation of orange oil, extraction yields from 72% to 82% of R-(+)-Limonene are achieved, being this the majority optically active form of the mixture in 90% [3]. More attractive than the limonene enantiomers are their corresponding epoxides, because of this molecule can be used at industrial level in pharmaceuticals, cosmetics, eco-polymers, flavors, fragrances. In addition, due to the limonene is a labile compound, it can be oxidated either air, heat, radiation or catalysis. In the synthesis of R-(+)-limonene oxidated compounds is intended increase selectivity and decrease variety of side products (Figure 1).
Based on previous, the general scope of this article is show the methods of R-(+)-limonene epoxidation, taking into account three guiding questions. The first one: For what is it important to do epoxidation of R-(+)-limonene?, where the importance of agroindustrial wastes and its potential as precursor of high added value products are evidenced. The second guiding question is: Why to do the limonene epoxidation? where the importance of the epoxides obtained from the R-(+)-limonene oxyfuntionalization is highlighted, and which industries employ it. The last guiding question is: How to do the R-(+)-limonene epoxidation?, focusing in two different ways. The first are the types of oxidizing agents commonly used in the oxyfunctionalization of our interest substrate (with in-situ and ex-situ generation methods) and the second way involves the kind of catalysis used.
Inside of the catalytic methods, there are two ways: I) Biological catalysis, where enzymes and microorganisms are present; and II) Chemical catalysis, where all chemical nature catalyst are involved. For chemical nature catalysts, they have a wide variety of catalysts, inside of them are: organocatalysts, photocatalysts and coordination compounds. Even more, the coordination complexes catalysts can be classified in two groups; the first group are the metal-salen ligand compounds and the second group are the organometallic catalysts, which are recognized by the presence of a covalent bond between a carbon and metal atom.
2 Method
In order to reach the purpose of this minireview article, three steps were made up, which are:
2.1 Locating the primary sources of valuable information
First, a qualitative-documentary methodology was implemented from a selection of publications (original articles, review articles, proceedings, patents, postgraduate thesis and so on), where the specialized search engine Google Scholar and Google Patents was used, and exploration in databases, such as: Proquest, Redalyc, Dialnet, ScienceDirect, Scopus, Scielo, American Chemical society, Chemical Abstracts (SciFinder), Web of Science (WoS) and digital catalogs of Strem Chemicals and Sigma. -Aldrich (Merck KGaA) in the publication periods ranging from 1990 to 2020. Then, to activate these searches, syntactic combinations of keywords were used to improve the search, such as: essentials oils, citrus fruits waste, limonene transformation, limonene oxides, limonene oxidation and limonene epoxidation mediated by catalysis. Once the results were obtained, a descriptive analysis of each document was carried out, where over one hundred publications were obtained, which were classified into three groups: the first one, for what is useful to carry out the research of the subject; the second group is, why suits to deepen in these investigations; and finally, the last group is, how this type of conversion has been carried out starting from different catalytic methods.
2.2 Bibliographic references selection criteria
For the selection criteria, one the duplicate papers found within the search in the different selected databases was discarded due to giving the same information. Next, a score was assigned, where five items were considered:
Considering scores from 1 to 5, giving the value of 1 as the lowest score and 5 as the highest rating, the articles chosen by assigning this rating, give as result high-impact journals (with h5-index upper than 3). After obtaining the respective score, and consequently the highest rated articles, were taken as those that provided the most information for the fulfillment of this review article, for example:
Title: Photo-epoxidation of (R)-(+)- and (S)-(-)-Limonene by Chiral and Non-Chiral Dioxo-Mo(VI) Complexes Anchored on TiO2-Nanotubes.
Summary: Over titania nanotubes, the effect of the ligand kind (Schiff base, terpyridine and bipyridine) in the stereoselective epoxidation of limonene was studied.
Purpose: To achievebstereoselective epoxidation of the two enantiomers of limonene using photocatalysis.
Methodology and data collection: Heterogeneous photocatalysts obtained by the grafting method and catalytic performance evaluated by GC-FID.
Findings: The catalysts employed in this reaction, once are photostimulated, they allowed the selective oxidation of (R)-(+)- and (S)-(-)-limonene using O2 as the primary oxidant, affording 53% of diastereomeric excess.
Score: 5
3 Analysis and Discussion
Citrus fruits are crops that usually have high adaptability to different climatic conditions, allowing their cultivation in a large number of countries, although the producing countries are generally found in the American continent and in the west of the European continent [4]. The countries of the Northern Hemisphere are the largest producers of citrus, with 58% of world production, with the remainder coming from the countries of the Southern Hemisphere. Citrus production in 2010 was led by China, Brazil and the United States. Brazil and the United States of America remain the two largest orange processing regions in the world [5]. On the other hand, the world orange manufacture for the 2020-2021 period increased by 7% over the previous period (2019-2020), to extent 49.4 million metric tons as a result of increased production in Brazil, China, and the European Union (table 1) [6, 7]. Because to grow oranges and citrus fruits in general, hot and humid climate conditions are required because oranges and citrus fruits are susceptible to cold, therefore, Brazil is the largest producer with 40% of world production [8].
In case of Colombia, it contributes about 2% of world citrus production, where 0,9% is orange, grown in 6 different geographical zones (table 2).
The benefits of this type of fruit lie in its high content of antioxidants, substances capable of blocking the damage of free radicals (such as pollution, tobacco, among others), avoid early aging of the body and prevent chronic and degenerative like the cancer [9].
Likewise, they stand out for their high content of nutrients such as C vitamin, which is not generated by the body, thus, it is necessary to obtain it through the daily intake of this type of fruit. This water-soluble vitamin promotes tissue repair and healing, strengthens blood vessels and maintains healthy bones, gums and teeth [10]. They are excellent allies of the respiratory system, because they increase defenses, relieve the discomfort of colds, flu, sore throat and muscle, and have antiviral and antibacterial action. Fiber is another nutrient present in citrus fruits; this cleanses the digestive tract, discards fats and toxins [11].
Thanks to the high demand for citrus fruits, a large number of tons of waste (peels) are generated from the industrialization of this type of crops. Where for the 51.8 million tons reported for the 2008 period, only 40% was used for the production of orange juice, leaving 13 million tons of waste orange peel unused [12]. In the case of Colombia, near to 71,943,813 tons/year are generated, where a large part of this number is incinerated or taken to landfills, further increasing environmental problems [13]. As for waste from the citrus fruit industry, it is estimated that more than 120 million tons are produced worldwide, where 40% is used in the extraction of industrial juice, the skin or peel, seeds remain as waste, and part of pulp, which represents around 20 million tons of waste. In Colombia this type of industry presents a low development, where the highest production is found in oranges with residues of approximately 228,128 tons/year [5]. The residues from the citrus fruit industry present a high potential as raw material in the elaboration of high added value products, these raw materials are: pectin, fiber, fixed oils and essential oils [14].
These citrus wastes can be dried out and employed as raw material for pectin extraction or pelletized for animal food [15]. However, a huge portion of citrus wastes is still discarded every year. This deposition is not ideal due to both economic and environmental issues such as high transportation costs, lack of retention sites, and the land-filling material having high organic content [16].
Due to the increase in the supply of waste generated by the agribusiness of citrus fruits, it becomes feasible to implement strategies for the use of these wastes and thus increase the economic impact generated by these crops and in turn reduce the negative environmental impact caused by their waste [17].
For orange peels, orange oil has an R-(+)-limonene (Figure 2) content between 94 and 96%, depending on the climatic and geographical conditions of yours crops [18].
In recent years, limonene in its optically pure form, which nature provides us from the "Chiral Pool", has gained a singular importance due to its demand as a biodegradable solvent, apart from industrial solvent it is also considered a transfer agent of clean and environmentally innocuous heat which is used in many pharmaceutical and food processes [14].
Limonene is used as a solvent for resins, pigments and inks in the manufacture of adhesives. Also, is used by the pharmaceutical and food industries as an aromatic component and to give flavor, being used in the obtaining of artificial mint flavors and in the manufacture of sweets and chewing gum [19]. Recent studies seem to point out that limonene has anticancer effects and it increases the levels of liver enzymes involved in the detoxification of carcinogens. Glutathione S-transferase (GST) is a system that eliminates carcinogens, as it promotes the GST system of the liver and intestines, attenuating the damaging effect of carcinogens. Studies in animals show that limonene in the diet reduces tumor growth in mammals [20].
As limonene molecule has two instaurations (Cl-C2, C8-C9), generally, this substance is easily oxidizable. Optically active epoxides derived from R-(+)-limonene are key molecules with multiple applications in the fine chemical manufacture.
Even the limonene oxides are more useful than the limonene as such, for example, in [21], starting from (+)-1,2-limonene oxide, it was used for the production of cis-(Z) and trans-(E)-α-bisabolene epoxides (Figure 3), which are the main component of the male sex feromene in Nezara viridula, and they are in charge of attractivity of males or females in the green stink bug (Nezara viridula).
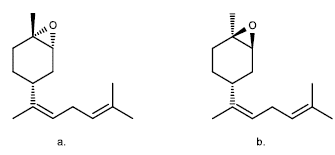
Figure 3 Isomers of bisabolene epoxide: a. trans-(E)- α-bisabolene epoxide, b. ris-(Z)-a-bisabolene epoxide [21].
In [22] was applied a fast and easy deconvolution approach to the Mainwald rearrangement of epoxides, like (+)-limonene oxide, with the aim of producing dihydrocarvone (Figure 4). The best performance was achieved by using bismuth triflate as a catalyst, reaching up to 57.6% yield, to obtain valuable carbonyl structures, which are interesting molecules for the synthesis of renewable polymers.
Other high value product of the industry is the di- and polyfunctional carbonates and specifically derívate from limonene oxides, because of these materials are the intermediates to attain polycarbonates (Figure 5), and non-isocyanate polyurethane (NIPU) (Figure 6), which contribute with the solar economy [23] and they represent a challenge for the auspicious of the green chemistry industry and the manufacture of products environmentally benign [24].
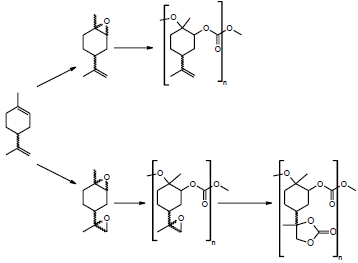
Figure 5 Synthesis of poly (limonene carbonate) (PLC), poly (limonene-8,9-oxide carbonate) (PLCO) and poly(limonene) dicarbonate starting from limonene oxides [27].
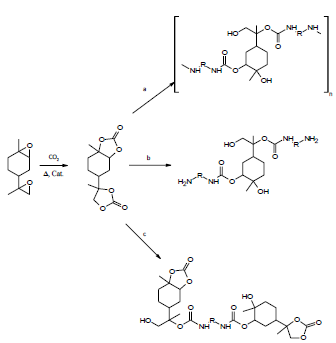
Figure 6 Synthesis of isocyanate-free poly (hydroxyurethanes) by different routes employing a molar ratio: a) Amine/carbonate of limonene = 1; b) Amine/carbonate of limonene > 1 and c) Amine/carbonate of limonene < 1 [29].
The limonene poly carbonate proceeds by a method where a co-polymerization is given by the interaction between two renewable reagents, such as racemic limonene oxide and carbon dioxide, where the addition of CO2 is catalyzed by diethylzinc ((CH3CH2)2Zn) [25]. Also, the access to optically active limonene polymers was achieved, using a β-diiminate zinc acetate complex as catalyst, at room temperature and 6.8 atm. The catalyst induces the reaction only with the trans diastereomer of limonene oxide, and the eis diastereomer stays unchanged [26].
Another case is the carbonation of limonene dioxide with carbon dioxide (CO2). It is also used to obtain limonene carbonates, which are intermediates that serve to prepare a great diversity of green polyurethanes with hydroxy, linear, branched and cross-linked functionalities. They do not require the use of phosgene or toxic isocyanate monomers due to its high carbonate content and rigid structure [28].
Generation of remediation of limonene carbonate with polyfunctional amines produces rigid isocyanate-free poly (hydroxyurethanes). Using diamines, different types of linear isocyanate-free poly (hydroxyurethanes) are generated with terminal groups and molar mass controlled by the molar ratio amine/carbonate (Figure 6). The amine- and carbonate-terminated isocyanate-free oligopoly (hydroxyurethanes) are exciting new additives and remediation agents for epoxy resins and the production of segmented polyurethanes [29].
All materials derived from the fixation to limonene oxides have good properties, such as: hardness, high molecular weight (> 1000 KDa), quality thermal resistance and good optical properties (high transmission and low haze) [30]. Even potentiates them as promising resins, coatings, thermoplastics, among others [31-33].
The epoxides from R-(+)-limonene have even been precursors of several aminoalkylalcohols, where the nature of the substituents located in the amino group change (Figure 7) [34]. The compounds obtained are used as chiral auxiliaries for the addition of diethylzinc to benzaldehyde, yielding an enantiomeric excess between 78% and 85% for (R) -1-phenyl-1-propanol.
Optically active epoxides from limonene not only implies an increase in the added value for the substance, but it is also a precursor of several synthetic compounds in pharmacy. The racemic mixture of endocyclic epoxide has been used to obtain numerous organic structures, one of them being (+)-cymbodiacetal [36]. This is a natural product, with a complex structure, isolated from the extracts of Cymbipogon Martinii [21], and whose configuration comes from the racemate (+)-1,2-limonene oxide (Figure 8).
Another transformation method is through the use of biocatalysis, using enzymes. In [37] the transformation of R-(+)-limonene was carried out using a chloroperoxidase belonging to the fungus Caldariomyces fumago. During this process, different products with low yields were obtained, such as: (E)-carveol (0.041%), carvone (1.099%) and an oxide with high added value (> 1%). This indicates that the enzymatic reaction conditions are not very efficient when implemented on an industrial scale.
The limonene epoxidation can be made considering the use of the oxidizing agent, and into this, the active oxygen content, because off this issue includes the environmental and economic issues.
In comparison with molecular oxygen, the use of other oxidizing agents generates stoichiomet-ric amounts of secondary products which can decrease the efficiency of the epoxidation reaction; furthermore, the active oxygen content is lower in comparison with the use of molecular oxygen or with the molecular oxygen/reducing agent combination. The use of sodium hypochlorite (NaOCl), peracetic acid (CH3CO3H), potassium per-oxymonosulfate (KHSO5), bis(trimethylsilyl) peroxide (((CH3)3SiO)2)) or iodosobenzene (PhIO), led to acids or bases that cause the epoxide ring-opening [51]. In some cases, the generated acids and bases do not intervene in the opening of the oxirane ring.
It is the case of in situ generated dimethyldioxirane (DMD) from a mixture of acetone and Oxone® (Figure 9), yielding an oxidizing agent with higher selectivity and yield in asymmetric epoxidation reactions [52].
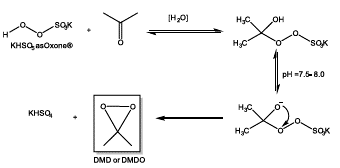
Figure 9 Generation of in-situ dimethyldioxirane starting from Oxone® (2KHSO5KHSO4K2SO4) and acetone.
On the other hand, the type of catalyst affects the asymmetric induction [53]. There are many types of catalyst involved in the oxyfuncionalization of limonene and even for its isomers specifically. For the biocatalysts, several examples exist. One of the most important techniques is the epoxidation by Prileschajew method [54], which consists in generating and electrophilic addition of one step, by the production of a species called peroxyacid. The peroxyacid is who transfers the oxygen atom to the double bond of the olefins. Bjsrkling et al [55], found that the use of lipases is an useful and efficient catalyst for the generation of peroxy-compound starting from carboxylic acids found in fats and oils. In addition, they observed that between different lipases, such as: Mucor mihei, Humicola sp., Candida antartica, Pseudomonas sp., and Candida cylindracea; the best one was Candida antartica, in terms of yield for peroxy compounds. For the case of substrates derivate from essential oils, and more specific for limonene, Oliveira. et al [56] performed the R-(+)-limonene epoxidation using fraction B of a lipase from a yeast (Candida antartica) and peroxyoctanoic acid (as oxidizing agent), to obtain 1,2-Limonene oxide (Figure 10). In this case, an enzyme amount of 5%, 70 mM of octanoic acid, 250 mM of hydrogen peroxide (as one of the precursors to generate the specie responsible for carrying out the substrate oxidation), and 40 nM of R-(+)-limonene; accomplished a yield of 74.92% with a recycle capability of 3 cycles, without a remarkable loss of its catalytic activity.
Ganapati et al [57], employed a similar system shown in Figure 10, but in this work, they immobilized the enzyme in a silica derived from the rice husk ash for a cross-linked method using 3-aminopropyl triethoxysilane (APTS) as silanization agent and glutaraldehyde as an cross-linking agent. Also, they obtained the limonene oxide using microwave radiation to improve the process yield, where the best conditions were 2 hours of reaction time, temperature of 50 °C, and a power of 50 W, to achieve a 75.35% of limonene conversion to 1,2-limonene oxide. One other work, attempted to achieve the oxidation of limonene but employing a chloroperoxidase from a fungus called Caldariomyces fu mago [58], where the enzy me influence a stereo and regioselective oxidation to the endocyclic oxide, and then consecutive oxidation mediated by the addition of hydrogen peroxide to obtain endo-cyclic diols. The reaction conditions used were: pH = 3 or 6, controlled by a buffer solution in the reaction mixture, and the presence and absence of chloride ions, achieving 99% of diastereomeric excess for (1S,2S,4R)-4-(prop-1-en-2-yl)cyclohexane-1,2-diol. Castellanos et al [59], made a study of the biotransformation of limonene and essential oils of orange and tangerine with high content of limonene (>70%). The reactions were carried out using the filamentous fungus Aspergillus niger DSM 821 as biocatalyst. Different reaction parameters were studied: culture medium, substrate concentration and oxygen availability. The main conversion products obtained were a-terpineol, p-menthane-1,8-diol and perillyl alcohol with the highest relative percent of 55,1%, 46,5% y 14,7%, respectively. Despite the good results both in regio and stereoselective presented by the biological type catalysts, their limited operating conditions prevent to be scaled to an industrial level, opening the opportunity to the chemical catalyst, due to this type of catalyst can support more severe conditions temperature, pressure, pH, among others; reaching similar results to the biocatalysts.
For the organocatalysts, in 1998, Armstrong et al [60] proposed to employ exocyclic iminium salts derived from pyrrolidine as catalysts for the oxidation of alkenes, along with the use of Oxone® as an oxidizing agent, where, the limonene conversion reached 98%, and a 93% of isolated yield with a 40% of diastereomeric excess. Years later, it was used a series of polyfluoroalkyl ketones as green catalysts for the cheap epoxidation of olefins, where the best catalytic activity was for 2,2,2-trifluoroacetophenone, bearing for limonene a yield to endocyclic limonene oxide of 81%. The improving of reaction is due to the activation of ketone by the oxidizing agent, where could be formed a perhydrate or dihydroperoxide specie (Figure 11), and one of both causes the oxo-transference to the substrate [61].
The limonene oxidation has been even made with photocatalysis, Ciriminna et al [63], took sunlight and titania P25 silylated to induce the R-(+)-limonene epoxidation, attaining a 79.3% substrate conversion and 54% of selectivity to endocyclic limonene oxide (cis/trans mixture). It is believed that the oxidation mechanism is generated by the singlet oxygen through energy promoted from the excited TiO2 to molecular oxygen adsorbed. Martínez et al [40], like Ciriminna employed sunlight to promote the epoxidation of limonene (both enantiomers), but this time used catalysts based on organometallics complexes of dioxomolybdenum(vi) anchored to titanium oxide nanotubes through ligands, such as: terpyridine, Schiff Bases and bipyridine. The best catalyst was the complex MoCl2O2(terpyridine)/TiO2-Nanotubes (Figure 12), regarding an 80% of conversion and 75% of selectivity to endocyclic epox-ides. Even, an analogous complex (MoCl2O2(Schiff base)/TiO2-Nanotubes) improves the selectivity to 86%, but with minor conversion (48%). The best diastereomeric excess for R-(+)-limonene and S-(-)-limonene were 36% and 34% respectively, both of them using the catalyst with the ligand Bipyridine. Usually, sunlight and this type of catalysts are employed to treat polluted water [64, 65] or involved in green oxidation for organic synthesis [66, 67]. This type of works evidences a new way to tackle the generation of these high added value compounds as the limonene epoxides are.
The heterogeneous catalysis has proved to give an alternative to this topic in different ways. Quiroz et al [68], used zeolites type NaMY, (where M = Fe2+, Co2+, Mn2+, and Mo2+) and NaCoMoY. The reaction was made employing molecular oxygen (O2) and iodosylbenzene (PhIO) as oxygen donors. The better catalytic performance was obtained with the bimetallic zeolite (NaCoMoY) yielding a conversion and selectivity to 1,2-limonene oxides (cis/trans mixture ratio equal to 2) of 57% and 53%, respectively. These good results are attributed to a synergistic effect into the metals interchange inside the zeolite [69], thanks to a spillover process that lets control the selectivity. Other attempts have involved the use of hydrotalcites synthesized by a co-precipitation method and the use of hydrogen peroxide as oxidant, reaching conversions over 63% and selectivities for endocyclic epoxides between 35 and 66% [70]. In addition to the solid supports used in the previous work, Villa et al. [71] explored the solvent effect on the hydrotalcite, ion exchange resins (Amberlite IRA 900,400 and 93) and poly-4-vinylpyridine doped with wolfram and molybdenum. These materials were assessed in the limonene epoxidation using aqueous hydrogen peroxide and the catalytic activity expressed in terms of limonene conversion and epoxides selectivity. Acetonitrile was found as the best solvent when the molybdenum catalyst supported on Amberlite IRA 900 reached a conversion of 84%, epoxide selectivity of 93%, and 1,2-limonene epoxides:8,9-limonene epoxides ratio of 30. In [72], reported the same oxidation system as the previous work, but using Ti-MCM-41 and Ti-BETA as catalysts, achieving the best catalytic activity for Ti-MCM-41 in conversion (25%), while the selectivity for both catalysts were very similar (60%).
In [73], reported the use of hydrogen peroxide and Ti-MCM-41 as catalyst, but in this work reached a maximum conversion of 52%. Moreover, it was found similar selectivities for both epoxides (endocyclic and exocyclic) as reported by [72]. According on the reported by [71], it is believed that the catalyst of wolfram supported on resin (PW-Amberlite) was deactivated after the reaction, therefore, it was proposed to wash the used catalyst with acetone (Table 4) to recover the initial catalytic activity [74].
An effort to enhance the asymmetric induction in the diastereoselective epoxidation of limonene was using the Jacobsen's catalyst, which has mainly been employed to cis-monosubstituted prochiral aromatic olefins; thanks to high electron density containing in the substrates like isoprene derivates, which ensures an efficient route to obtain desired products [75]. Cubillos and colleagues heterogenized manganese(III)-Salen complexes in its achiral and R,R-chiral version by using a mesoporous solid like Al-MCM-41 (Figure 13) [41]. In this work was found that homogeneous catalysts exhibited complete selectivity towards the endocyclic epoxide with conversions over 50%. On the other hand, their heterogeneous counterparts increased the conversion, but the selectivity decreased 32 and 19% for the achiral and chiral form, respectively. Also, these catalysts could be recycled until three times without significative loss of the initial catalytic activity.
In other work, it was reported the immobilizing of Jacobsen's catalyst on modified silica by a multi-step grafting method [76]. With the heterogenized R,R-Jacobsen catalyst (R,R-NH2-SiO2) was achieved the best catalytic activity results with a total conversion of R-(+)-limoneno, a selectivity to 1,2-limonene oxide of 72% and diastereomeric excess to cis-1,2-limonene oxide of 20%.
In addition to that, more inorganic microporous supports such as TS-1 and Ti-SBA-15 were tested in [72]. This work highlights a remarkable loss of selectivity with the raise of temperature, obtaining the best selectivity to 1,2-limonene oxide at 0 °C. Malko et al [77], used montmorillonite as catalyst for the oxidation and isomerization reactions of R-(+)-limonene. Once, the catalyst was obtained by a purification process of bentonite using a sedimentation method, it was followed to evaluate the catalytic activity. For the oxidation reaction were detected different products, such as: carveol, carvone, perillyl alcohol and the epoxides (endocyclic epoxide, exocyclic epoxide and diepoxide). Despite afford 80% of conversion, the selectivity was low due to the different products obtained. The isomerization reaction showed high conversions too, but in this reaction, the selectivity was towards p-Cymene. In contrast, [44] employed a tungsten-based polyoxometalate and aqueous hydrogen peroxide. The reaction parameters studied were temperature, oxidant, solvent, acid, and sodium sulphate (this compound was to prevent the limonene oxide hydrolysis). They founded a complete selectivity to the 1,2-limonene oxide at 50 °C with limonene/hydrogen peroxide molar ratio equal to 4, catalytic load of 1% and without solvent. Another work operating with titania supported with mesoporous materials as catalyst was reported by [78]. They dopped KIT-6 with titanium oxide and tested it in the limonene epoxidation with tert-butyl hydroperoxide (TBHP) in aqueous phase as oxidant. The catalytic activity results obtained were 23% of conversion and 60% selectivity to 1,2-oxide limonene. Gawareka et al [79], used Ti-MCM41 and Ti-MWW as catalysts with TBHP for limonene oxidation. The best reaction conditions to obtain a 52% of limonene conversion and 39% of yield to perillyl alcohol (main product), were: 130 °C, TBHP/R-(+)-limonene molar ratio of 1, 80% wt of amount solvent, 3% of catalyst load, 6 hours of time reaction and the catalyst Ti-MWW. Another important contribution to the limonene epoxidation was by using a system included: alumina (Al2O3) as catalyst, aqueous hydrogen peroxide (H2O2, 30% wt) as oxidant and ethyl acetate (CH3-COO-CH2-CH3) as solvent [80]. This work shows an efficient green method to fully access limonene diepoxide. Also, this method shows an alternative to use cheap starting material and no toxic for the obtention of this valuable epoxide.
Up to here, some of the most relevant works in heterogeneous catalysis were showed. By the other side, the homogeneous catalysis also is involved in the limonene oxidation reactions. From this point, we will see some of the most outstanding works in this area.
Some coordination compounds like metal-porphyrins have been used as catalysts. Tayebbe et al [81], reported the electronic and steric effects employing the system Mn(porphyrin)OAc-NaI04 as catalyst in the R-(+)-limonene and stilbene (cis/trans mixture) epoxidation; they evaluated two different catalysts Mn(TPP)OAc (TPP: meso-tetraphenyl-porphyrin dianion) and Mn(TMP)OAc (TMP: meso-tetramesityl-porphyrin dianion) (Figure 14). The best catalytic activity was reached for the catalyst with the meso-tetraphenyl-porphyrin as ligand, affording a total conversion of the substrate and 68% of selectivity to 1,2-limonene oxide.
Moreover, Trytek et al. [82], used a tetraphenylporphyrin photoexcited to promote the R-(+)-limonene oxidation. The best yield (814.6 mg/L) was obtained for a different product from the conventional oxidation products. By mass spectrometry, the authors proposed that its product could be 6,6-dimethylcyclo-octa-2,4-dienon or verbenone (Figure 15). The second majority yield was reported for carvone (172 mg/L) [83].
Lima et al [45] used metal-Salen complexes (metal: Mn, Ni or Co) (Figure 16) as catalyst for the limonene oxidation, moreover evaluated the influence of two different oxidizing agents (sodium hypochlorite NaCIO and iodosylbenzene PhIO) and four different solvents ( acetone, acetonitrile, dicloromethane or ethyl acetate). This work reported the best selectivity to carveol and carvone with the manganese coordinated complexe. Thus, this metallic center resulted as the most efficient for limonene allylic oxidation.
Another Salen ligand used as chelating agent to coordinate metallic centers is the ligand type N,AT-Bis(3,5-di-im-butylsalicylidene)-l,2-cyclohexanediamine (Figure 17), which coordinated to a manganese atom is known as the Jacobsen's catalyst. This catalyst was designed by Erick Jacobsen and Jay Larrow, to promote high enantios-elective epoxidation of non-functionalized aromatic conjugated olefins and hydrolytic kinetic resolution of racemic epoxides [85, 86].
Other homogeneous catalysts kinds are the Salen-metal complexes. These types of catalysts show an remarked improvement due to the Salen chelate ligand, who is compose by a salicylaldehyde (Sal) and a diamine (en). The enhance is thanks to the ligand design, because it has various advantageous, such as: ease in synthesis, coordination capability with a wide range of metals, different geometry acquisition and let the metallic center exhibit various oxidation states [84].
Pinto et al. [87], used ionic liquids in the epoxidation of limonene, using the Jacobsen's catalyst supported on l-n-butyl-3-methylimidazolium tetrafluoroborate (BMIBF4) (Figure 18). They found that the combination of (R,R)-Mn(Salen) with (R)-limonene or (S,S)-Mn(Salen) with (S)-limonene generated diastereomeric excesses between 57% and 74%; and that the combination of (S,S)-Mn(Salen) with (R)-limonene or (R,R)-Mn(Salen) with (S)-limonene decreased diastereomeric excesses (between 7% and 22%). In this way, they showing that the asymmetric induction was controlled by the chiral center of the olefin.
Other attempts with the Jacobsen's catalyst were made evaluating the effect of chirality present in it. In studies carried out by Cubillos et al [41, 52, 88], was explored the effect of the substrate chirality of the Jacobsen's catalyst (racemic, achiral and optically active)(Figure 19) and the oxidizing agent in the diastereoselective epoxidation of R-(+)-limonene with in-situ generated DMD in both homogeneous and heterogeneous phase. For both phases, similar catalytic activity was found in the chiral (R,R and S,S) and achiral catalysts, which suggested that the preferential formation of the main product does not depend on the chirality of the catalyst but on the chiral center of R-(+)-limonene. This result represents a clear economic advantage, since R-(+)-limonene is much cheaper than Jacobsen's catalyst. They also found that using a monophasic water-acetone mixture as solvent and in-situ generated DMD as oxidant (Figure 9), monoepoxide and the diepoxide were the main products, again indicating that there is no effect of catalyst chirality on the catalytic activity; instead of this the catalytic activity is attributed to the high degree of coordination of the manganese atom more than the chiral centers of the Shiff base ligand. The oxidation of olefins using the Jacobsen's catalyst is normally carried out using a catalyst concentration between 1% and 10% molar with respect to the amount of olefin.
Notwithstanding, the scarce use of the Jacobsen catalyst at the industrial level due to its low reuse and loss of asymmetric induction when it is immobilized on solid supports, are aspects that are open to investigation, specifically in reactions with substrates from agroindustrial wastes. In this sense, R-(+)-limonene has high potential in the production of fine chemical products. The above considerations are enough to concentrate on future research and thus widen the knowledge of this type of catalyst in their different stereochemical forms so that they are considered potential candidates for the development of highly efficient and environmentally compatible catalytic processes.
4 Conclusions
Next, we point out the main conclusions derived from this minireview article:
R-(+)-limonene is an attractive alternative to take advantage from the valorization agroindustrial wastes into fine chemical products. In this sense, the optically pure limonene oxides are very important intermediates for the total synthesis of biologically activity compounds.
In situ generated DMD showed the possibility to reuse the Jacobsen's catalyst without the need of an immobilizing procedure on a solid support. Also, this allowed the easy purification of the reaction products.
The diastereoselective epoxidation of R-(+)-limonene has been achieved through the use of different catalytic systems, such as: biocatalysis, photocatalysis, heterogeneous catalysis (supported mesopore materials), homogeneous catalysis (organocatalysis, coordination compounds). Even the use of catalytic hybrids has been the new trend, such as light-activated coordination complexes, where it is expected to increase the yield to a desired eutomer and decrease the formation of distomers.
Inside different catalysts, for the biocatalysts, the best yield was 75.5% with a total selectivity to the endocyclic epoxide, using the Prilescha-jew method epoxidation and a fraction B of a lipase from yeast (Candida antartica) supported on silica as an enzyme. The problem presented by these biocatalysts are the narrow operation conditions, which reduce its possible use at industrial level.
In the case of chemical catalysts, the best catalytic performance in the R-(+)-limonene epoxidation is presented by the Jacobsen catalyst employing different oxygen sources from inorganic or organic types, such as: H2O2, PhIO, m-CPBA, among others. But, the use of in-situ generated DMD as oxidizing from KHSO5 and acetone as solvent, allow the useful reuse of the catalyst due to the segregation of a solid phase insoluble with the reaction liquid mixture without remarkable loss of initial catalytic activity.