Services on Demand
Journal
Article
Indicators
-
Cited by SciELO
-
Access statistics
Related links
-
Cited by Google
-
Similars in SciELO
-
Similars in Google
Share
CT&F - Ciencia, Tecnología y Futuro
Print version ISSN 0122-5383On-line version ISSN 2382-4581
C.T.F Cienc. Tecnol. Futuro vol.2 no.3 Bucaramanga Jan./Dec. 2002
1Ecopetrol - Instituto Colombiano del Petróleo, A.A. 4185 Bucaramanga, Santander, Colombia
2Universidad Nacional de Colombia - Faculty of Chemical Engineering - Bogotá, Colombia
e-mail: cberdugo@ecopetrol.com.co
ABSTRACT
This work presents a membrane bioreactor prototype used to separate emulsion phases formed in the biodesulfurization reaction. Hydrophobic membranes used for the construction of the prototype allow the separation of the organic/watery phases. The separation unit resembles a tube and carcass heat exchanger. By feeding the emulsion through the housing and due to the pressure gradient pushed on the membrane, the organic phase pass through and allow to obtain an organic phase free of cells and water. Several organic phase/watery phase ratios and many cellular concentrations were evaluated. Results indicate that is possible to separate the phases by manipulating the fluid pressure within the bioreactor. This is possible even for cellular concentrations of the order of 7 g/l. The system can also be used as a reaction unit. The biological conversion was evaluated by verifying the presence of 2-HBP, one of the metabolites of the path 4S in the biodesulfurization reaction. This bioreactor configuration has not been explored before for the biodesulfurization process and therefore it represents an innovation in this research area.
Keywords: Membrane reactor, biodesulfurization, biocatalysis.
RESUMEN
En este trabajo se presenta un prototipo de biorreactor de membranas para la separación de las fases de la emulsión formada en la reacción de biodesulfurización. Las membranas hidrofóbicas utilizadas para la construcción del prototipo permiten la separación de las fases acuosa orgánica. La unidad de separación se asemeja a un intercambiador de tubo y coraza. Alimentando la emulsión por la carcasa y gracias al gradiente de presión que se ejerce sobre la membrana, la fase orgánica permea la membrana permitiendo la obtención de la fase orgánica libre de células y agua. Se evaluaron diferentes relaciones fase orgánica/ fase acuosa y diferentes concentraciones celulares. Los resultados indican que es posible separar las fases manipulando la presión del fluido al interior del biorreactor incluso a concentraciones celulares del orden de 7 g/l . El sistema puede ser utilizado también como unidad de reacción, la conversión biológica se evaluó verificando la presencia de 2-HBP, uno de los metabolitos de la ruta 4S en la reacción de Biodesulfurización. Esta configuración de biorreactor no ha sido explorada antes para el proceso de biodesulfurización y por tanto representa una innovación en el área.
Palabras clave: prototipo de biorreactor, biodesulfurización, biocatálisis.
INTRODUCTION
Petroleum-constituent organosulfured compounds contribute to the formation of acid rain, to environmental pollution and to catalyst poisoning. Environmental regulations are increasingly demanding and conventional technologies for the removal of sulfur may be very expensive to achieve the removal ranges required.
Research groups working in this area of expertise have dedicated their efforts to the search of new technologies that may be both competitive and effective to meet legislation regulations. Among these new unconventional technologies, sulfur removal through biological means represents an alternative for the development of clean, environmentally safe technologies that may complement refinery processes.
The biodesulfurization technology involves multiple research topics. Biocatalysis may be carried out through the use of complete cells or isolated enzymes, either free or immobilized. Biocatalytic conversion and finishing may be affected by critical factors such as selectivity and specificity of the biocatalyst; stability of the biocatalyst; biocatalytic activity; and, bioreactor design, in which the volumetric ratio between the organic phase and the watery phase represents the main limiting factor for the industrial application of the bio-technological process (Setti et al., 1996). One of the bottlenecks for this technological development is the design of bioreactors at the reaction and organic-phase separation stages, where few groups have showed improvements, due to the complexity of the process. The Colombian Petroleum Institute (Instituto Colombiano del Petróleo -Ecopetrol - ICP) has been working in the implementation of the biodesulfurization technology, addressing some of the topics involved in the development of this technology, such as biocatalyst enhancement (through genetic engineering tools) and process development.
The biodesulfurization process scheme, as addressed by the ICP, involves a biocatalyst production phase. In this regard, culturing strategies have been implemented in order to bio-increase the biocatalyst, up to the levels required for the development of the process (Berdugo et al., 2001). Following the biocatalyst production phase, the contact reaction with the organic phase takes place, in order to remove sulfur. In this stage, the formation of a very stable emulsion has been evidenced. This emulsion does not allow to obtain a final product free of a watery phase. Different studies at laboratory scale were carried out at the ICP in order to separate the emulsion. They included centrifugation, chemical breakers, temperature and pH. The results showed that only by modifying the pH with acid was possible to separate the emulsion with an efficiency of around 12% for an emulsion 20/80. However, this alternative could present problems when scaling due to the problem generated by the acids handling.
To this date, there is only one biodesulfurization pilot plant developed at Monticello, by Energy Biosystems Inc. (Texas, USA) -now Enchira Biotechnology-, using stirred-tank bioreactors for desulfurization of medium distillate's light fractions. These reactors feature organic phase/watery phase volumetric ratios that do not exceed the 50% mark, to avoid microbial inhibition and formation of stable water-oil emulsions. It has been observed that this type of bioreactor features many impairing limitations for industrial applications; therefore, new bioreactor designs for reaction and process separation stages have gained enormous significance.
The bioreactor's design depends on many physical-chemical factors, such as the oil/water volumetric ratio; oxygen availability; cofactors regeneration; diffusing mechanisms between watery and organic phases; biocatalyst recovery; and, phase separation that may produce a desulfurized product, free from the biocatalyst and the watery phase. At lab and bench scales, certain reactor alternatives have been assessed for biodesulfurization reactions. (Kaufman et al.,1997; Setti et al., 1996). However, difficulties in up-scaling and enhancing of separation efficiencies still persist, thus reinforcing the need to explore new alternatives, such as the one described in this paper.
Among unconventional reactors that have been used in industrial applications, membrane bioreactors attract attention, because they can host integrated biocatalysis and separation processes. Membrane bioreactors combine selective, chemical-reaction mass transfer with selective removal of inhibitory products, in order to increase reaction conversions (Giorno and Drioli, 2000). Through the use of appropriate systems, cells or enzymes may be immobilized while simultaneously reaching bioconversion, product separation and enrichment in one single operating unit (Belfort, 1989, quoted in Cass et al., 2000). These reactors have been used in several biotechnological applications, such as waste water treatments (Pearson, 2002).
Then, it follows that the biodesulfurization reaction within a membrane system should offer the following advantages:
- Recovery of the biocatalyst after the microbial treatment
- Higher oil/water volumetric ratios, as compared to the 50% mark achieved by stirred-tank reactions
- Disposal of smaller quantities of exhausted watery media after treatment
- Easy separation of oil from the biocatalyst
During this research, a membrane bioreactor was designed and built, and an assessment was carried out in order to determine whether such bioreactor may be used in the separation of the emulsion formed in the biodesulfurization reaction. The bioreactor prototype acts as a tube and carcass heat exchanger in making possible to separate the organic phase through the use of hydrophobic membranes in the tube covering. In the selected operating scheme, the emulsion is loaded into the carcass and the organic phase is recovered through lumen. The bioreactor proposed herein is intended to carry out both the bioconversion and the separation stages in one single unit. This article presents and discusses the results for the module design and the phase separation and bioconversion-related aspects.
MATERIALS AND METHODS
Microorganism and culture medium
Assays were performed while using a native stub ICP 172 (Gordona rubroperthencus). Inoculants were obtained in nutritious medium (Merck® brand). For the production phase, the ICP4 culture medium was used, as standardized in the ICP biotechnology lab (Berdugo et al., 2001), containing glucose as carbon source and Dimethylsulfoxide (DMSO) as organic sulfur source.
In the biodesulfurization and emulsion formation reactions, a phosphate buffer 0,15 M with 2% glucose was used as the single source of carbon plus diesel as organic phase, in the proportions required for the assessed organic phase/watery phase ratios.
Cellular concentrations were determined via optical density, using a calibration curve read in a Cary spectrophotometer at 610 nm.
Membranes
For membrane selection analysis, considered about 10 different membranes were considered with hydro-philic and hydrophobic characteristics available in the market. Two types of Millipore® membranes with hydrophobic characteristics were selected.
The two (2) hydrophobic membranes are made of poly-vinylidene (PVDF) featuring 0,5 :m pore size and GVWP reference, and membranes made in ethylene poly-tetrafluoride (PTFE) featuring FHLP reference, 0,5 :m pore size and 45 mm diameter, with a polyethylene support for higher consistency, were used in the preliminary assays. For the construction of the bioreactor prototype, 262 mm diameter, PTFE membranes were used.
Chemical compatibility
To determine compatibility of the membrane and the solvent used, an integrity assay was performed, using the manufacturer's bubble point as reference. Membranes were placed in diesel and in a diesel-water blend for fifteen (15) days. Then, an assembly made up of two (2) recipients connected through a filter carrier containing the membrane was used. Using methanol, air was passed through, in order to displace the liquid from one recipient to the other, slowly increasing air pressure at the entry until a constant flow of bubbles at the exit was observed. Bubble-point pressure is reached when continuous bubbling is observed.
Rupture pressure
Assays were carried out in order to determine rupture pressure for membranes, as a factor to define the reactor's operating variables. An organic blend featuring a 3:1 organic phase/watery phase ratio was used in this assay. Pressure was increased 5 psi every 2 minutes, verifying only the organic phase path. The rupture pressure is the pressure at which the watery phase starts to pass together with the organic phase, thus indicating that the membrane has suffered a fracture.
Permeability
Assays were performed in order to determine the trans-membrane pressure at different flows of permeated organic phase. Diesel and a 1:3 diesel-water blend were used as fluids to be assessed. The system was conditioned by recycling the fluid being fed and by maintaining a constant pressure head. Pressures were varied through the manipulation of line exit valves. The flux for the permeated phase was determined (Mass flow or volumetric flow per area unit).
Construction of the bioreactor prototype
The tubular membrane's body was manufactured with a stainless steel mesh with cylinders 1/8" in diameter. It was sealed with stainless steel and tin welding, At the ends, 5 cm diameter pipe was placed to allow coupling with the bioreactor.
The PTFE membranes were sticked to the mesh with an adhesive (Loctite brand). Previously, the adhesive and the applied weld were subjected to a toxicity test.
The prototype is made up of a carcass and a membrane in its interior, both assembled as concentric tubes. The carcass has two ports, dedicated for fluid input and exit. The membrane's ends are isolated from the housing so that, the fluid of the housing only pass through membrane's interior if it moistens the Teflon® membrane. The system is equiped with gauges to the entrance and exit of the membranes's interior. In the housing a digital indicator of pressure, Druck brand is located. In the input ports, for the housing and the internal membrane, valves on-off were placed. The exit ports have needle valves to manipulate the flow and therefore to control the pressure.
Separation trials
Two (2) sets of experiments were performed. The first set assessed separation at different cellular concentrations while maintaining the organic phase/watery phase at a 25/75 ratio. The second set assessed separation at different organic phase/watery phase ratios (60/ 40, 50/50, 40/60, and 25/75), at cellular concentrations of 3 and 7 g/l .
Biocatalytic reactions
Contact reactions were made with 25% organic phase (diesel) and 75% watery phase. Cellular concentrations varied from 16 to 20 g/l . Permeated diesel was collected and forwarded for sulfur removal assessment. Reactions were simultaneously carried out in stirred reactors and abiotic controls, in order to compare results from the new prototype with those obtained via the conventional STR reactor.
Hydroxibifenile Determination (HBP)
The HBP's determination was carried out using liquid cromatography using a HP1090 Series II chromatographer. Supelcosil LC-18 Column (25 cm x 4,6 mm x 5 mm). As eluent a gradient 10:90 ACN was used: water up to 100% in 18 minutes, maintaining 100% ACN up to 20 minutes, then 10:90 ACN: water to 25 minutes, maintaining a flow of 1 ml/min. Detector DAD, 260 nm , wideband (10 nm) and injection volumen of 5 ml . HBP standards in diesel matrix were prepared to compare retention times of the HBP both in samples and standards.
RESULTS AND DISCUSSION
Membrane Assessment and Selection
For membrane selection, an initial market survey was made on available membranes and characteristics to be considered under the topic of interest. Most hollowed-fiber membrane reactors have hydrophilic features; therefore, it was necessary to assess different types of membranes to select the bioreactor's construction material. The first assays were intended to assess the membrane's characteristics and its resistance to the interest matrix, as well as to estimate the operating conditions.
Chemical compatibility assays
The membrane's integrity was assessed through the bubble point test suggested by Millipore® for the assessment of filtering systems. Any significant difference between the observed bubble point and that specified for a specific membrane indicates that the membrane is damaged. Results for this test are included in Table 1. After comparing the bubble point specified by the manufacturer and the bubble point obtained after the exposure of same to the organic phase (diesel), it is possible to state that the membrane's integrity remains unaffected.
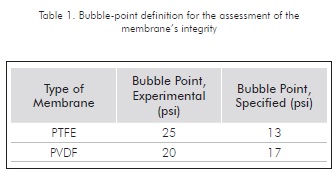
Rupture pressure
Assays on these two types of membrane provided results indicating that the rupture pressure for the Teflon® membranes was around 20 psi (see Table 2). As for the Durapore® membranes, the value at which the watery phase (that is, the phase that does not moistens the membrane) passed through was 30 psi. The rupture pressure defined for the Teflon® (PTFE) membrane coincides with the 21 psi , reported by Woodley and Schröen (1997). Although the Durapore® (or PVDF) membrane showed higher resistance and would allow for a wider range of pressures to work with, the PTFE membrane is best fitted for the experiments, since it enabled the organic phase's to pass through at lower pressure, thus indicating, from a separation perspective, less resistance and higher permeating flux.
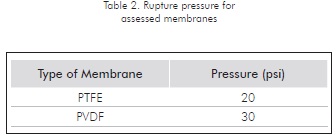
Comparisons for results included in Tables 1 and 2 show that the rupture pressure is lower than the pressure defined for the bubble point, a fact that would appear to be contradictory, since it would indicate that the membrane fractures before it reaches its bubble point. This incongruence may be justified by the addition of the tensoactive agent to the emulsion used for rupture pressure assays. According to research by Vaidya et al., (1994), the presence of bio-surfactants causes a transition in the membranes moisturizing ability. This phenomenon lowers the pressure required to force through the non-permeating phase, thus indicating that the membrane fractures at a lower pressure than if it had not been exposed to the tensoactive agent.
Prototype
Assessment of the material's toxicity
The toxicity assessment for the material used in the construction of the prototype showed that, as per the counts of microorganisms (included in Table 3), the conditions at which the assays were performed do not show any relevant toxic effects, either from the soldering or the adhesive used. This observation on bacterial feasibility is possible through the comparison between the initial recount for the cellular suspension used and follow-up recounts. The fact that the different recounts retained the same magnitude order was defined as the applicable assessment criterion.
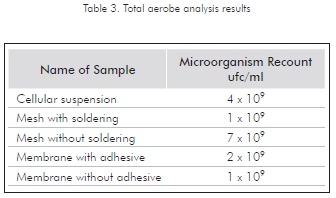
There are two (2) basic operating schemes for this type of units or reactors. The first one is the conventional, tubular bioreactor scheme. Under this scheme, tubular membranes or fibers are contained in a carcass, and cells are confined within the extra-capillary space (Figure 1). The flow profiles for this scheme have been modeled by Brunining, 1989, and Kelsey et al., 1990. Nutrients mass transfer is carried out via diffusive transportation through the membrane, between the medium flowing axially through the lumen (or membrane's interior) and the cells on the exterior (Piret and Cooney, 1990). This system, called "closed-shell", is widely used in cultures of animal cells.
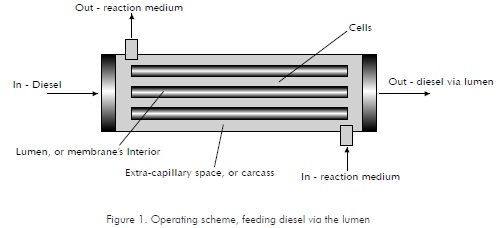
As for its application in bio-catalysis for organic phases, biomasses will be found in the exterior space of the tubular membrane and, considering that the lumen's entry pressure is higher than the extra-capillary space or carcass pressure, then the diesel flow will pass through to the cells. At the other extreme, the lumen's pressure will be lower than inside the carcass and, therefore, the diesel will return to the membrane's interior.
In the second operating scheme, as proposed by Patankar and Oolman (1990), cells are immobilized in the membrane wall's matrix. The nutrient-filled medium circulates axially through the carcass. A convective flow is induced, causing some of the circulating medium to flow from the carcass into the lumen.
In this case, both the diesel and the reaction medium with cells in suspension are fed by the carcass (Figure 2). Under this operating scheme, diesel is permeated through the membrane and collected by the lumen. The scheme could feature a variation, in which the emulsion is fed via the lumen and the diesel is collected via the carcass.
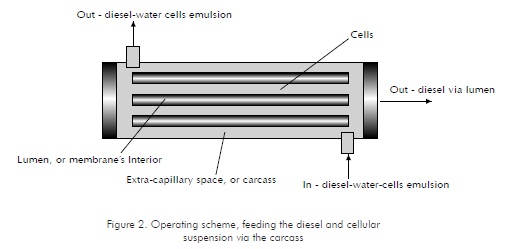
For the selection of the operating scheme, assays with no cells were performed. Results for the first operating scheme showed that a flow profile similar to that modeled by Brunining, 1989, and Kelsey et al., 1990, may not be obtained, since the required differences in pressure at the lumen and the carcass may not be achieved. In these assays, the carcass pressure was maintained at atmospheric pressure (No data shown). As for the second operating scheme, the flow characterization within the system showed that variations in the emulsion flow being fed produce an inversely proportional response from the permeated diesel flux (See Figure 3). Flux measurements were performed at time periods of approximately 22 minutes. Based on these results, it may be said that the system can be operated below 50 ml/min , since, as observed in Figure 3, higher emulsion flows do not translate into higher permeated diesel flows recovered.
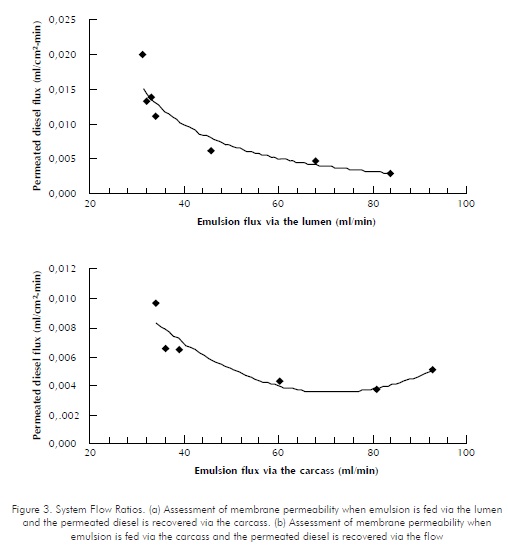
As shown in Figure 3, there are no substantial differences between emulsion feeding via the carcass and emulsion feeding via the membrane's interior. However, according to Patankar and Oolman (1990), one of the advantages provided by this configuration is that mass transfers to and from the cells are improved by convective flow transportation. Therefore, the operating scheme in which the emulsion is fed through the carcass and the permeated diesel is collected at the membrane's interior was selected.
The separation unit may act as a bioreactor under a combined scheme involving a stirred tank and a membrane unit operating on an intermittent basis, as shown in Figure 4. This operating scheme has been reported as used in several applications, such as: a fixed stratum coupled to a hollowed-fiber module, for extractive fermentation (Dai et al., 2000); a membrane module for antibodies production (Gramer et al., 1999); a system used in protein hydrolysis (Deeslie and Cheryan, 1981); a membrane bioreactor for treatment of phenol-polluted waste waters (Loh et al., 2000); and, production of nutraceutic foods (Sehanputri and Hill, 1999).
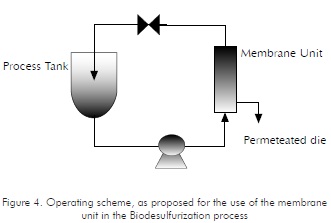
The proposal for the Biodesulfurization process calls for the System to operate on an intermittent basis. The contents of the process tank should be pumped into the membrane unit and then returned into the tank. In the stirred tank, ventilation is provided as required by the process, causing sulfur concentrations to decrease. This, in turn, provides for sufficient residence times, while the desulfurized organic phase is gradually removed.
Trans-membrane pressure
Behaviors of hexadecane, diesel and emulsion fluxes versus trans-membrane pressure variations are shown in Figures 5, 6 and 7, respectively. As expected, increments in trans-membrane pressure increase flows passing through the membrane. Assays were performed with a feeding flow of approximately 43 ml/min , at room temperature.
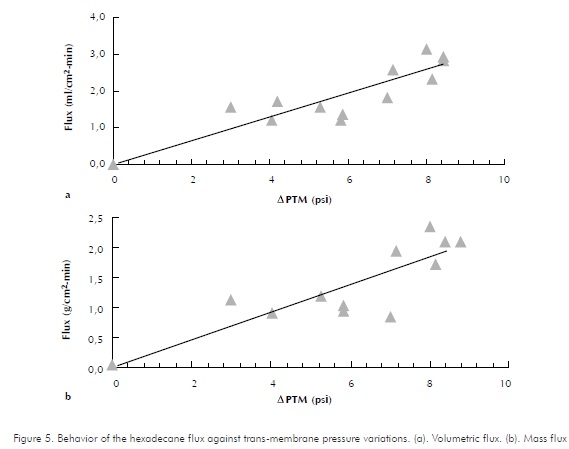
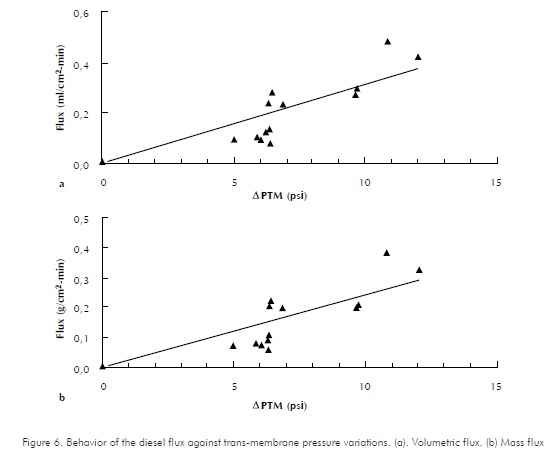
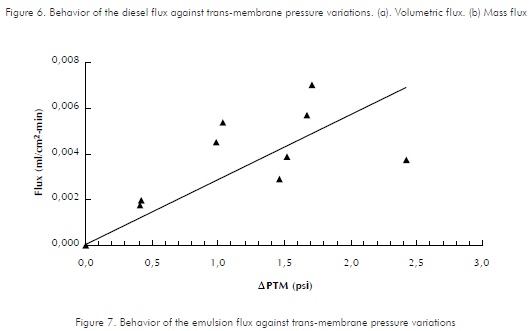
Flux variations for a permeated, cell-free substance against the trans-membrane pressure gradient within a typical membrane process operating with cross-flows, are reflected as a straight line. This behavior is analogous to that of a pure substance in a ultra-filtering system (Tejada, 1995). A straight-line trend is identified by Luque et al, (1999) and by Cass et al, (2000), while assessing hollowed-fiber membrane modules. Therefore, the lines presented as trends in the graphics are adjusted to a straight line. It is possible that data dispersion is due to an experimental error caused while trying to perform two-fold assays, due to the difficulty to obtain the same trans-membrane pressure drops. This is evident in Figures 6 and 7. In the first one, for 1,5 psi variations in APTM, pressure drops 5 to 6,5, a similar value to that of the permeated flux is obtained. Likewise, as observed for the different points of Figure 7, through small variations in APTM, the permeated flux varies around 0,001 ml/cm2-min .
The line's slope in Figures 5, 6 and 7 represents the permeability coefficient. By calculating statistical correlation coefficients for datasets, the slopes that indicate permeability coefficients are: with hexadecane, pure compound, slope value is 19,26; for diesel, it is 1,8844 and for the emulsion, it is 0,172. The statistical correlation coefficients (as calculated in excel spread-sheet, for datasets) are 0,8870, 0,8704 and 0,6765 for Hexadecane, Diesel and emulsion respectively. Therefore, membrane's permeability is different depending on the type of permeable substance and decreases as purity degree of the compound decreases. The above, explains the decrement of permeability flux observed by comparing hexadecane, diesel and emulsion. Cass et al., (2000) reported a reduction in the permeability flux along against transmembrane pressure decrease due to the components present in the enzymatic raw extract used.
Assessment of the membrane bioreactor operations
Figure 4 shows a schematic diagram for the bioreactor's operation. The operating conditions for the prototype were based on the results from independently- performed assays on membranes. Therefore, operating pressure was initially defined within the 10 to 15 psi interval. Separation tests were carried out under these conditions. The system's pressure was monitored through the installation of manometers, both at the lumen and at the carcass, and it was manipulated through flow variations and gauge valves installed at the system's in and out points.
Phase separation assays
For the process involving the biocatalyst, the cross-membrane flux is expected to be affected by the membrane's soiling. To assess this scenario, the biocatalyst used in biological desulfurization (Gordona rubroper-tinctus) was added to water/diesel blends, measuring the cross-membrane flux as a time function.
Figure 8 shows the behavior of the diesel-water-cells system, at different cellular concentrations (1 g/l , 3 g/l , and 7 g/l). The organic/watery phase ratio was 25/75, while the APTM was around 1 psi. Flux dramatically decreases as a time function during the first 3 to 4 hours, and then tends to level up at a constant value. For the 1 g/l concentration, flux decreases nearly 68%, during the first three (3) hours, as compared to the first measurement. For the 7 g/l concentration, flux reduction is also 68%. As for the 3 g/l concentration, data obtained fail to show the same tendency. This may be due to the fact that several difficulties were faced at the beginning of the assay, while stabilizing the initial pressure drops.
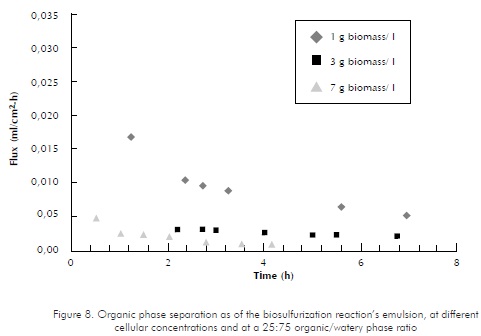
The loss of permeated flux in the bioreactor system coincides with results reported in reference literature. Schroën and Woodley (1997) assessed flux through the membrane of a Teflon® module as a time function, for a water-sunflower oil-yeast blend. Flux values decreased nearly 89% in 30 minutes, due to soiling by cells or by cell-produced components (particularly proteins).
The diesel recovery percentage at the four hour mark was of 43% for the 1 g/l concentration, of 14,6% for the 3 g/l concentration, and of 6,6% for the 7 g/l concentration.
The organic phase to emulsion ratios were varied for cellular concentrations of 3 and 7 g/l , in order to compare behaviors with higher proportions of diesel in the emulsion. Figure 9 shows replications of assays at 3 g/l concentrations, at 60/40, 50/50, and 40/60 ratios. APTM for these assays oscillated around the 2 psi mark. The figure shows that, as the organic phase proportion increases, the decrease in permeated flux is less noticeable. These results are similar to those found in reference literature, Schroën and Woodley (1995) showed how in a water-octanol-yeast emulsion at an organic/water phase ratio of 75/25, flux decreases 92% in 35 minutes (97,6% when ratio is changed to 50/50). The graph shows that for the 50/50 organic/watery phase, the permeated diesel flux is higher than that for a 60/40 ratio, and in graph b, it is close to that for a 40/60 ratio. This behavior may be attributed to sudden pressure changes occurring during the assays. As for higher permeation configurations, at the 50/50 ratio, pressure was very unstable, oscillating between 2 and 5 psi ; meanwhile, pressure for the 60/40 ratio remained fairly stable, between 1,6 and 1,98 psi readings. In reference to the replication of these assays, where pressure ratings were similar for the 50/50 and the 40/60 ratios, the assay pressure for the 40/60 ratio oscillated between 3 and 4 psi. Presumably, variations in APTM were due to biomass accumulation that generated slight system tampons, causing axial pressure drops at the carcass of the membrane unit. These results do not include data obtained for the 25/75 organic/watery phase ratio, since they are not comparable for they were obtained at different APTM values.
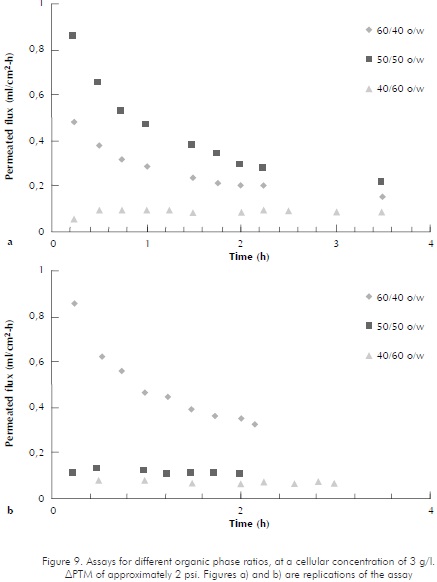
According to the results, it is important to notice the influence of the o/w ratio and the APTM, like associated parameter, and its stability in the system. However, it can be concluded that there is a tendency in which the bigger the organic phase portion the better the separation efficiency under the evaluated conditions, 3 g/l and 7 g/l .
Figure 9 also shows how the permeated diesel flux decreases against time. An analogous behavior has been described in other researches (Cass et al, 2000; Patankar and Oolman, 1990; Tompkins et al., 2000), and it is considered to be due to the membrane's pore blocking, as caused by the accumulation of cells on its surface (Patankar and Oolman, 1990; Schroën and Woodley, 1997; Luque et al, 1999) and by protein adsorption (Piret and Cooney, 1990; Patkar et al., 1993).
At cellular concentrations of 7 g/l (Figure 10), higher organic phase proportions in the emulsion generate higher separation rates for same. APTM for these assays was kept at around 2 psi . The graphic behavior of the 50/50 ratio is very similar to that of the 60/40 ratio, but, as expected, it is less evident.
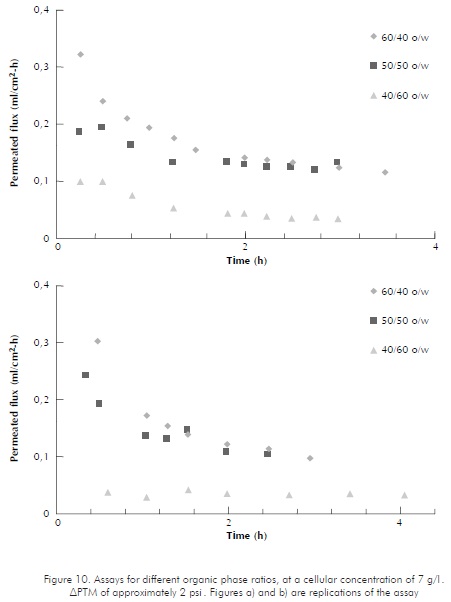
While assessing diesel recovery percentages at the two-hour mark, at 3 g/l concentration levels, an average 26,4% of recovery was observed for 60/40 ratios,
Organic phase fraction
Figure 11. Diesel flux through the membrane, in function of the organic phase's volumetric fraction, at different cellular concentrations. ∆PTM of approximately 2 psi against 12.05% for 40/60 ratios. It might be stated that organic phase separation at 60/40 ratios is twice as high as separation at 40/60 ratios. At 7 g/l concentration levels, separation percentage of 12,64% was observed for 60/40 ratios, while 5,83% was observed for 40/60 ratios, thus showing a similar trend to that for lower concentrations. Results for 50/50 ratios are not compared against the aforementioned, since they are very similar to those for 60/40 ratios, as shown in graphs of Figure 10.
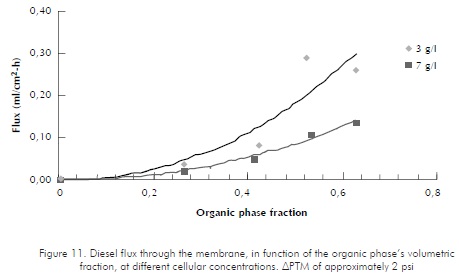
Finally, product quality specifications, as set forth by Ecopetrol, provide for a maximum water content of 500 ppm in regular diesel. Analyses on the permeated diesel provided results of 144 ppm of water content, a value well below the permitted maximum concentration level.
Contact Reactions
Contact reactions were carried out using 25% of organic phase (diesel) and 75% watery phase. Two controls, abiotic and upset, were applied. The abiotic control serves as target and the upset control as comparison parameter with the conventional system (STR). According to ICP's studies (Berdugo et al., 2001) the biggest desulfurization is reached in the first twelve hours of the process. Samplings were made at 12 and 24 h . As a variable answer to validate bioconversion reactions, the qualitative determination of 2-HBP utilizing liquid chromatography was used. 2-HBP is a metabolite produced in the biodesulfurization reaction that uses microorganisms following route known as 4S. Sulfur present in the DBT is removed as sulfate and generate 2-HBP (Ohshiro et al., 1995). Figures 12 and 13 show the chromatographic profile found in the determination of HBP in two of the reactions carried out. Figure 12 shows the production of HBP in the unit membrane for 12 and 24 h . Figure 13 shows a chromatographic profile for 12 h and compares the membrane reactor with the STR conventional system and the negative controls for an original sample (Diesel) and an abiotic reactor. The samples of diesel and abiotic reactor do not show a 2-HBP peak while the reactor of upset tank and the membrane system show production of 2-HBP, being more prominent production of HBP in the membrane system that indirectly indicates more efficiency in the reaction. This can be attributed to the combined STR system and the membrane that increase the efficiency of mass transfer by means of system blending and recirculation.
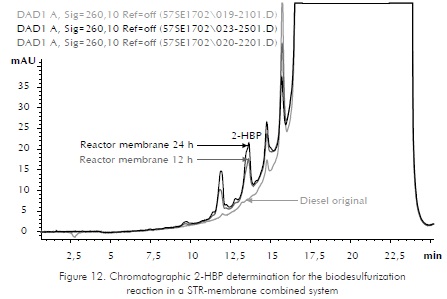
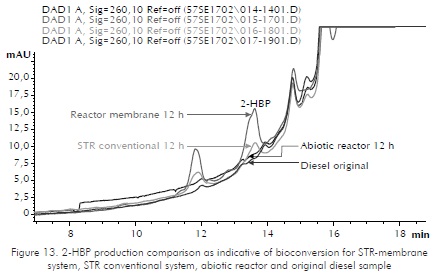
CONCLUSIONS
-
A membrane bioreactor prototype was constructed, featuring a configuration similar to that of a tube-and-carcass interchanger. At controlled pressure and flux conditions, this prototype allows for the recovery of the organic phase from an organic/watery phase emulsion.
-
Results for the watery phase/organic phase biocatalyst separation indicate that separation efficiency increases as the organic phase proportion increases. These results are aligned with the development of a biosulfurization technology, which is intended to handle higher organic-to-watery phase ratios. Additionally, the need to use a biocatalyst that tolerates higher organic phase concentrations for the desulfurization reaction is confirmed.
-
Given the cellular concentrations required for the Biodesulfurization process, and taking into account results from this research, a scheme may be considered, such that it might include more than one separation module, each with different membrane pore sizes, so that rough separation activities might be carried out in the first stages, followed by separation activities such as those discussed in this research, under the prototype's design conditions.
-
The organic phase recovery percentages varied between 5,83% and 26,4%, depending on the cellular concentrations and the organic/watery phase ratios. The separation's final product does not contain cells, and the water contents fall within applicable specification requirements.
-
This research is the basis for the development of an operating scheme for integrated or isolated reaction and separation processes. Guidelines and recommendations deriving from this survey constitute a new proposal for the construction of a bioreactor for the contact stage of the biodesulfurization process.
ACKNOWLEDGEMENTS
The authors wish to acknowledge the financial support provided by ICP - Ecopetrol during the development of this research. They also wish to state their words of gratitude to the Biotechnology research group, for its support and contributions to the study.
BIBLIOGRAPHY
Belfort, G., 1989. "Membranes and bioreactors: a technical challenge in biotechnology". Biotechnology and Bioengineering, 33: 1047-1066. [ Links ]
Berdugo, C., Mena, J. A., Acero, J. R. and Mogollón, L., 2001. "Increasing the production of desulfurizing biocatalysts by means of Fed-Batch culture". CT&F - Ciencia, Tecnología y Futuro, 2 (2): 7-15. [ Links ]
Bruining, W., 1989. "A general description of flows and pressure in hollow fiber membrane modules", 44 (6): 1441-1447. [ Links ]
Dai, Xiao-Ping, Luo, R. and Sirkar, K., 2000. "Pressure and flux in bead-filled ultrafiltration/microfiltration hollow fiber membrane modules". Biotechnology Progress, 16: 1044-1054. [ Links ]
Cass, B. J., Schade, F., Robinson, C. W., Thompson, J. E. and Legge, R. L., 2000. "Production of tomato flavor volatiles from a crude enzyme preparation using a hollow-fiber reactor". Biotechnology and Bioengineering, 67 (3): 372-377. [ Links ]
Deeslie, D. and Cheryan, M., 1981. "A CSTR- Hollow Fiber System for continuous hydrolysis of proteins. Performance and Kinetics". Biotechnology and Bioengineering, 23: 2257-2271. [ Links ]
Giorno, L. and Drioli, E., 2000. "Biocatalytic membrane reactors: applications and perspectives". Tibtech., 18: 339-349. [ Links ]
Gramer, M., Poeschl, D., Conroy, M. and Hammer, B., 1999. "Effect of harvesting protocol on performance of a hollow-fiber bioreactor". Biotechnology and Bioengineering, November 5, 65 (3): 334-340. [ Links ]
Kaufman, E., Harkins, J., Rodríguez, M., Tsouris, C., Selvaraj, P. and Murphy, S., 1997. "Development of an electro-spray bioreactor for crude oil processing". Fuel Processing Technology, 52: 127-144. [ Links ]
Kelsey, L., Pillarella, M. and Zydney, A., 1990. "Theoretical analysis of convective flow profiles in a hollow-fiber membrane bioreactor". Chemical Engineering Science, 45 (11): 3211-3220. [ Links ]
Loh, K., Chung, T. and Ang, W., 2000. "Immobilized-cell membrane bioreactor for high-strength phenol waste-water". Journal of Environmental Engineering. January. [ Links ]
Luque, S., Mallubhotla, H., Gehler, G., Kuriyel., Dzenge-leski, S., Pearl, S. and Belfort, 1999. "A new coiled hollow-fiber module design for enhanced microfiltration performance in biotechnology". Biotechnology and Bioengineering, November 5, 65 (3): 247-257. [ Links ]
Ohshiro, T., Hirata, T. and Izumi, Y., 1995. "Microbial desulfurization of dibenzothiopheno in the presence of hydrocarbons". Appl. Microbiol Biotechnol., 44: 249-252. [ Links ]
Patankar, D. and Oolman, T., 1990. "Wall-growth hollow-fiber reactor for tissue culture: I. Preliminary experiments". Biotechnology and Bioengineering, 36: 97-103. [ Links ]
Patkar, A., Bowen, B. and Piret, J., 1993. "Protein adsorption in polysulfone hollow fiber bioreactors used for serum-free mammalian cell culture". Biotechnology and Bioengineering, 42: 1099-1106. [ Links ]
Pearson, D., 2002. "Easing wastewater woes through membrane filtration". Chemical Processing, January, pp. 24-27. [ Links ]
Piret, J. and Cooney, C., 1990. "Mammalian cell and protein distributions in ultrafiltration hollow fiber bioreactors". Biotechnology and Bioengineering, 36: 902-910. [ Links ]
Setti, L., Lanzarini, G. and Pifferi, G., 1996. "Immobilized cells for applications in non-conventional systems". In: Immobilized Cells: Basics an Applications, Wiffels, R., Buitelaar, R., Bucke, C. and Tramper, J., (Eds), 777-784. [ Links ]
Schröen, C. and Woodley, J., 1997. "Membrane separation for downstream processing of aqueous-organic biocon-versions". Biotechnol. Prog., 13: 276-283. [ Links ]
Sehanputri, P. S. and Hill, C. G., 1999. "Biotechnology for the production of nutraceuticals enriched in conjugated linoleic acid: I. uniresponse kinetics of the hydrolysis of corn oil by a pseudomonas sp. lipase immobilized in a hollow fiber reactor". Biotechnology and Bioengineering, September 5, 64 (5). [ Links ]
Tejada, A., Montesinos, R., and Guzmán, R., 1995. "Bioseparaciones". Sonora, México, Editorial Unison. [ Links ]
Tompkins, K., Murphy, L., Owsenek, B., Pignataro, R. and Rodríguez, A., 2000. "Ultrafiltration membrane polishing system for ship boat treatment of oily wastewater". Naval Sea Systems Command, Crystal City. [ Links ]
Vaidya, A., Halling, P. and Bell, G., 1994. "Surfactant-induced breakthrough effects during the operation of two-phase biocatalytic membrane reactors". Biotechnology and Bioengineering. 44: 765-771. [ Links ]