Services on Demand
Journal
Article
Indicators
-
Cited by SciELO
-
Access statistics
Related links
-
Cited by Google
-
Similars in SciELO
-
Similars in Google
Share
CT&F - Ciencia, Tecnología y Futuro
Print version ISSN 0122-5383On-line version ISSN 2382-4581
C.T.F Cienc. Tecnol. Futuro vol.2 no.3 Bucaramanga Jan./Dec. 2002
1Asphalt Research Group (GIAS), Universidad Industrial de Santander, Chemical Engineering School
Carrera 27 calle 9. Ciudad Universitaria. Bucaramanga, Santander, Colombia
e-mail: gias@uis.edu.co
ABSTRACT
The present work shows the rheological properties of the three Colombian asphalts produced in the refineries at Barrancabermeja, Cartagena and Apiay, exposed to the open air during 18 months using a specially designed testing bank. Rheological behavior was evaluated using the new specifications of SHRP technology in the Brookfield and DSR rheometers to determine viscosity, Shear Stress, Shear Rate, Dynamic Share Modulus and other related variables. The measurements were made using different temperatures and load times.
Keywords: Asphalts, climate, aging, rheological properties.
RESUMENT
En el presente trabajo se estudian las propiedades reológicas de los tres tipos de asfaltos industriales producidos en Colombia, en las refinerías de Barrancabermeja, Cartagena y Apiay, después del envejecimiento durante 18 meses en un banco de pruebas al aire libre especialmente diseñado. La evaluación del comportamiento reológico se realizó a través de ensayos basados en las nuevas especificaciones de la tecnología SHRP en los reómetros Brookfield y DSR para determinar características tales como: viscosidad, esfuerzo de corte, susceptibilidad térmica, velocidad de deformación y módulo de corte dinámico, a diferentes temperaturas y tiempos de carga.
Palabras clave: Asfaltos, clima, edad, propiedades reológicas.
INTRODUCTION
Asphalt binder for roads, as any material obtained from petroleum, has its limitations in its response to transport loads that increase day after day in frequency and intensity, without mentioning the aging caused by the climate, constantly generating changes in its physical and chemical properties and as a result, premature failures and cracks. As a consequence, the rheological behavior of asphalts occupies a fundamental level of importance for its appropriate application on the construction of roads, also in handling during the mixing process, and in service and quality control. Another reason for rheological research is the durability and preservation of asphaltic coatings and the sinthesys of new modified asphaltic materials. To obtain durable material, projects should seek optimization of binder rheology, which studies strain and fluence of those materials in which strain depends not only on magnitude but also on application rate, loading time and environmental conditions.
Traditional asphalts have a very complex rheological behavior that depends mainly on temperature, loads and application rate. At low temperatures and low load application rates, asphalt acts as an elastic solid, while at high temperatures and longer load rates, it acts as a viscous liquid; at intermediate temperatures it is characterized as a viscous-elastic fluid.
The importance of rheological characteristic refers to viscosity as a function of the temperature. However, a series of assays and techniques have been standardized in order to study it in detail using rheometers that give broader information about the fundamental properties of asphalts by means of dynamic assays, studies of cracks and the type of rheological flow based on the climate and the type of traffic that is typical in the region (Bolzan, 1995). These assays correspond to the new specifications for Superpave asphalt binders proposed in the "Strategic Highway Research Program" (SHRP). These specifications are closely linked to the main problems in flexible pavements such as permanent strain, cracking due to stress and thermal fracture (Chaney, 1997).
The chemical composition, rheology and physical-chemical properties of asphalt are affected by increases in temperature. Therefore, one of the basic characteristic rheological curves for asphalts is viscosity as a function of the temperature (Tia, 1985). The relation between viscosity and temperature is a critical property of the asphalt binder, because it establishes the temperature required while handling the binders during production and application of asphalt mixtures (Kafarov et al, 1996): service temperature in warm and hot temperatures (0-60°C), hot mixing (>130°C) and placement and spreading of mixtures (Agnusdei et al, 1973; Agnusdei and Iosco, 1998).
For Newtonian fluids, viscosity η as the relation between shearing stress τ and strain rate γ is constant and the flow curve is represented by a straight line in logarithmic coordinates, whose slope characterizes the index of complex flow, which is equal to 1,0 (Tuffour and Ishai, 1990).
For non-Newtonian fluids presenting a complex fluidity phenomenon, such as asphalts at relatively high temperatures, the flow curve is not linear. That is, viscosity depends on other variables, such as fluence rate and shearing stress, as well as the geometry of the assay, the previous history of the material, and others. In this case, the non-Newtonian behavior of fluids is described by means of an empirical equation denominated the law of power, based on Oswald's law that relates fluence rate with shearing stress, where k and c are constants for a particular fluid (Agnusdei and Iosco, 1998), as follows:
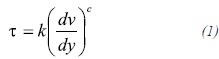
The measurement of k is a characterization of the consistency of a material, also called apparent viscosity, c is denominated the index of complex flow and represents a measurement of the degree of complexity of the material or, in other words, the degree of remoteness of simple or Newtonian fluence, and (dν/dy) is the strain rate for non-Newtonian flows. According to some researchers, (Arenas and Sanabria, 1991), a straight line is obtained in logarithmic coordinates, for the shearing stress and fluence rate, determined for asphalts, being the slope of this line the index of complex flow c, which is less than 1,0 for non-Newtonian fluids, such as the Apiay asphalt.
METHODS AND PROCEDURES
This study evaluates the rheological properties of three types of industrial asphalts produced in Colombia, under the influence of the climate after 18 months of aging in a specially designed outdoor testing bank, located in Guatiguará, Santander (Afanasieva et al., 1998), to determine total climatic influence and each factor under conditions of different types of protection from climatic factors, such as: ambient temperature, relative humidity, wind velocity, volume of precipitations, global sol radiation and ultraviolet radiation in the regions UVA, UVB and UVC. Results are shown in Table 1.
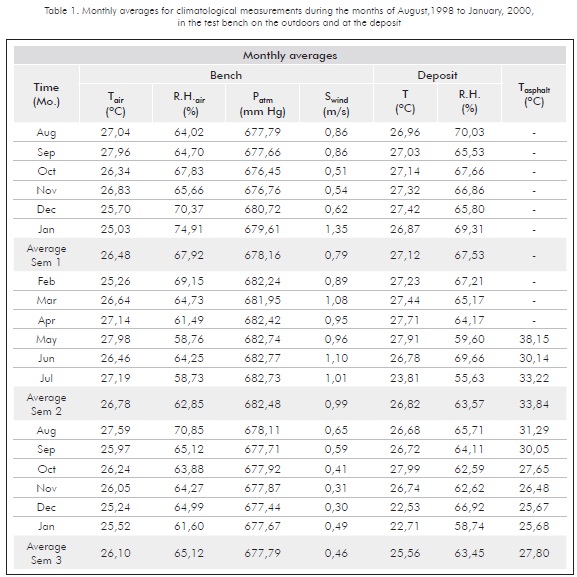
For purposes of the research, three types of asphalts were chosen:
CIB, CIB + Aggregate and CAR Asphalt
These asphalts are produced at the Industrial Complex in Barrancabermeja (CIB) and at the Cartagena Refinery (CAR), respectively. They come from mixing the vacuum residues with a naphtenic base and the intermediate vacuum residues, adjusting penetration (70-90), softening point and flash point by adding different amounts of gas-oil to the same crude oils. An asphalt extracted from the mixture of the same asphalt with the calcareous type aggregate (limestone), called CIB + Aggregate, will also be studied. Complete physical-chemical characterization is shown in pre vious works (Afanasieva et.al., 1996, 1998, 1999).
APIAY Asphalt
The asphalt produced at the Apiay plant (Departamento del Meta) is obtained by means of the mixing of a heavy crude oil extracted from Campo Castilla (65%) and Apiay (35%). The physical-chemical characterization of this asphalt has been previously published (Afanasieva, 1996, 1998, 1999; Arenas and Sanabria, 1991).
Physical-chemical characterization was carried out monthly on the asphalts in the test bank in order to evaluate their changes during the aging process. Properties such as penetration, absolute viscosity, density, softening point, ductility, flash point and chemical composition were obtained using methods from the ASTM standards. The physical chemical properties of the three initial asphalts and those aged during 18 months are shown in Table 2.
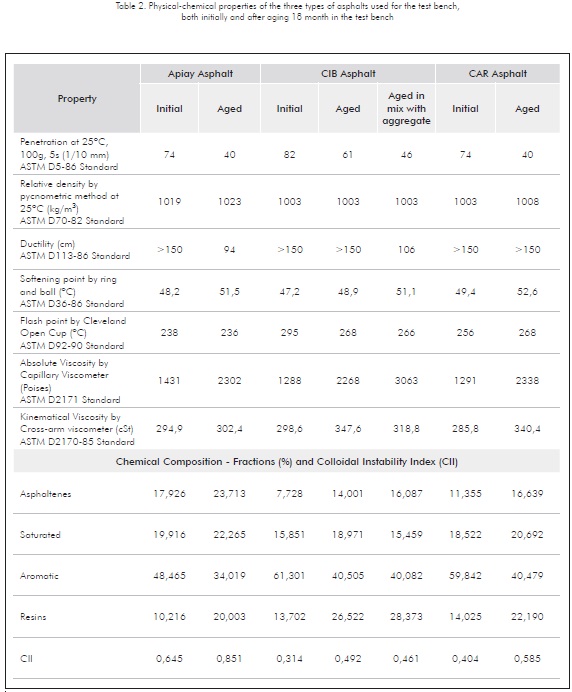
The SHRP specifications of the new Superpave technology (Bolzan, 1995; Chaney, 1997) introduced, new rheological characterization by means of the Dynamic Shear Rheometer (DSR). In this work properties such as resistance to permanent strain, cracking temperature and temperature of vitreous transition were determined using the DSR tests. Other rheological curves of the initial and aged CIB asphalts and the CIB extracted from the mixture with the aggregate, and Apiay asphalt were calculated after measurements in a Brookfield Thermosel Rotational Viscometer, evaluating properties such as viscosity, shearing stress and strain rate in a wide range of testing temperatures and loading times.
An apparatus Thermosel DVII Rotational Viscometer, Model LV in accordance with ASTM standard 4402 was used for the evaluation of the original and aged materials. During the test, the asphalt was initially fluidized at 110°C for homogenization and placed in the sample holder. It was later brougth to 90°C using the Thermosel system to begin measurements. The equipment has a temperature regulator with a RTD sensor that regulates temperature fluctuations at a range of ±1°C . The needle has been previously submerged in the sample because the sample holder and the needle should have a constant and even temperature.
The equipment has a software to specify the size of the needle, rotational speed, the number of readings per temperature and the time between each reading. The data of rotational speed, torque required to rotate the needle (%), viscosity (cps), shearing stress (dinas/cm2) and strain rate (seg-1) are also reported. The parameters mentioned above are measured using the same needle and gradually elevating the temperature (Figures 1-3) for each sample of the original asphalt (Apiay, CIB and CAR) and the aged asphalt (Apiay, CIB, CAR and CIB + Aggregate).
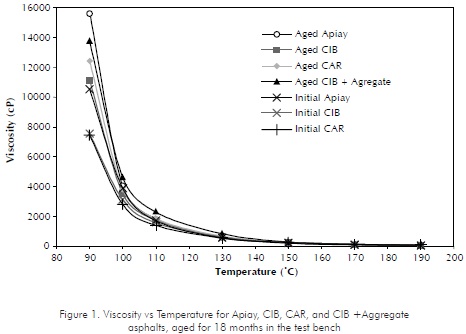
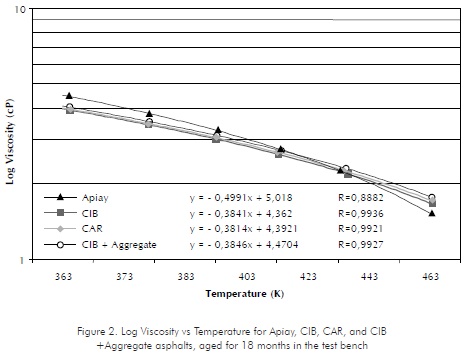
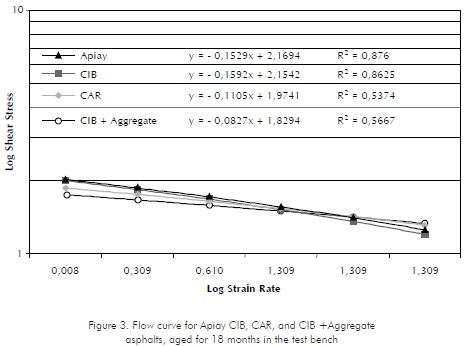
Tests were done to establish the ratios of rotational viscosity and complex shearing modulus based on the specifications of the SHRP technology for evaluating the properties of resistance to climate and strains.
The study of rheology also implies the study and evaluation of the influence of the duration of loads or the shearing force. It's required to apply high forces at given ranges to obtain measurements of viscosity and strain.
The rheological characterization of asphalt binders includes the study of strain and flow of solid or liquid materials, and by analogy, elastic and viscous categories are established. This characterization, as mentioned before, is made using a dynamic shear rheometer (DSR) applying an oscillatory strain on a sample and the consequent measurement of the resulting strain. The apparatus used in this study was a DSR Rheometrics with a special geometry (similar to cone-needle geometry).
The primary response in this kind of dynamic testing at the DSR is the complex dynamic modulus, usually expresed by the term G* and shows the relation between the absolute value of oscillatory shearing stress and applied angular strain. Just as described by Bolzan (1995), Tia and Ruth (1985), this parameter evaluates the total asphalt resistance to strain when it is repeatedly submitted to constant stress in the zone of linear viscoelasticity.
The strain test consists of an increase in the angle of oscillation with variations in applied strain, maintaining temperature and frequency constant. The temperature selected for this test was 40°C , because this is the approximate average climatic temperature of service for a pavement in this region of Santander (Afanasieva et al., 1999).
The test was performed on original asphalt, whose linear viscoelasticity zone was determined, and it is characteristic of the region where the strain and stress relation is function of only frequency (or time) and temperature.
The elastic modulus or modulus of stiffness (G*/sen L) is a measurement of resistance to permanent strain caused by the consolidation of asphalt layers or base layers, and/or plastic fluence of the mixture. These values should be low for the asphalt to have good resistance to strain. To reduce the problem of permanent strain due to the binder at high temperatures, a minimum value for the parameter G*/sen L is established at 1 kPa in the original asphalt and 2,2 kPa in aged asphalt for the laboratory test on fine rotating film or RTFOT (Bolzan, 1995; Tia and Ruth, 1985).
The viscous modulus or the modulus of dissipated energy in the form of heat per strain cycle (G*sen δ), also called dynamic loss modulus, is a parameter used to consider fracturing due to binder fatigue caused by the action of repeated loads applied on the structural layers and manifests itself on the surface of the pavement as a network of interconnected cracks. The cracking generally begins at the upper part of the asphaltic layer or at the base where the tensions of traction are critical under the action of traffic. Just as in the elastic modulus, this other modulus was measured at a testing frequency of 10 rad/s, as in Bolzan's work, 1995.
The specifications calculated by the DSR require control of stiffness given by G*/sen δ at high temperatures and thus ensuring that the asphalt provides clear action on the shearing stress composed in terms of viscosity temperature. According to Chaney (1997), showing some procedures with automatic tests, a diagram of G*/sen δ versus temperature is made to determine the cracking temperature of the original sample.
The effect of the load is based on the strain rate given by the DSR at 10 rad/s. This is equal to a traffic speed of about 80 km/h. The asphalt samples will have to show very high stiffness to support load rates imposed by heavy traffic or trucks with a permanent load.
ANALYSIS AND DISCUSSION OF RESULTS
When comparing the viscoelastic behavior of each aged asphalt with its corresponding initial asphalt, it is observed for the Apiay aged asphalt (Figure 1) that its viscosity at low temperatures is much greater than the corresponding to the initial asphalt, but with a progressive increase in temperature, viscosities on both curves do not show significant differences. This proves again that asphalt is a material that behaves like a viscous fluid at high temperatures. Its viscosity decreases significantly when temperature increases (Tuffour and Ishai, 1999).
The viscosities for asphalt extracted from the equally aged CIB + Aggregate mixtures are very far from the viscosities presented by the initial pure CIB asphalt. The aged CIB asphalt shows intermediate rheological behavior. This is how the CIB + Aggregate asphalt has shown great changes in structure due to environmental aging, to the same action of direct contact with the aggregate and its highest resulting thermal susceptibility regarding the pure CIB asphalt.
The aged CAR asphalt is more viscous at testing temperatures than the initial CAR asphalt. There is a very notorious difference in viscosities at original testing temperatures. However, as temperature increases, there are small differences in these values. It is worth mentioning that initially, the CAR asphalt is more susceptible to temperature than the CIB asphalt.
Figure 1 also shows that initially at low temperatures (90-100°C), the values of cinematic viscosity behave in agreement with the following decreasing order: Apiay > CIB + Aggregate > CAR > CIB. That is, the effect of increasing the temperature is greater for the Apiay asphalt based on a mixture of heavy crude oils. The opposite occurs with the refined CIB asphalt (vacuum residues), which has low viscosity values at relatively low temperatures. By increasing temperature, the influence on viscosity is greater for CIB asphalt after contact with the aggregate. The fluctuation of viscosity at high temperatures is small for the rest of the asphalts.
Thermal susceptibility is related to the rate at which asphalt consistency (in relation to its colloidal nature, and particle size) decreases due to an increase in temperature. In rheological terms, this viscosity - temperature ratio varies in agreement with each asphalt (Tia and Ruth, 1985; Crawford, 1992). The logarithmic coefficient of temperature (Viscosity Temperature Susceptibility), or thermal susceptibility, represents the slope obtained in a graph of the logarithm of the viscosity in function of temperature. Figure 2 illustrates this behavior for the four types of asphalts that are studied in this work. The figure show that the consistency (average viscosity) of each asphalt changes considerably as temperature increases. This figure is based on an analysis of linear regression to evaluate the value of the slope in terms of thermal susceptibility.
In Figure 2, the Apiay asphalt has a steeper slope. That is, the changes in its consistency due to temperature are more significant and, it is more susceptible to thermal changes and less resistant to hot climates. In general, this behavior will make the Apiay asphalt more fluid than the other asphalts and therefore, lower stability at temperatures above 90°C , which makes pavements "crack" more easily due to abrupt changes in temperature. The slightest change in consistency is obtained for the CAR asphalt being that it presents a reduced slope. This asphalt is not as viscous and hard at high temperatures, and it is much more fluid than the previous asphalt. It can provide pavements with great stability. The values of consistency for CIB and CIB + Aggregate asphalts can be found among the extreme values represented by the Apiay and CAR asphalts. Therefore, they have an average or intermediate thermal susceptibility. The following is the order of thermal susceptibility for asphalts aged for 18 months under the tropical climate of Santander: Apiay > CIB + Aggregate > CIB > CAR.
Figure 3 shows the fluence rate under the dynamic shear stress for the different types of aged Colombian asphalts. It can be concluded from these diagrams that the complex flow index is lower than 1,0 for all of the aged asphalts that were characterized and therefore, are described as non-Newtonian flow at testing temperatures. As the complex flow index decreases, complexity and similarity to the Newtonian flow of each asphalt increases. The following decreasing order indicates this rheological behavior in asphalts: Apiay > CIB > CAR > CIB+ Aggregate. That is, the asphalt that is farthest from Newtonian behavior is the Apiay asphalt, and the closest is the CIB + Aggregate asphalt.
Thermo-oxidative climatic aging leads to changes in chemical composition and increases the breaking of asphaltenes, which affects colloidal structure and causes rheological changes, reflected in the fact that asphalt flow becomes more complex. This is why the complex flow index is closely linked with asphalt composition. Asphalts with SOL type colloidal behavior have a Newtonian behavior at ambient temperature. As this index decreases, asphalt complexity increases, presenting a high asphaltene content and an imperfect peptization phenomenon causing a GEL type asphalt. Therefore, a complex flow is generated by the resulting non-Newtonian behavior and an increase in the degree of asphalt gelification (Agnusdei and Iosco, 1998).
Figure 4 shows the behavior of viscosity in all of the asphalts that were analyzed (initial and aged) during the measured ranges, indicating the dependence of viscosity on applied force time. It shows that asphalt viscosity decreases with the increase in time. This behavior is known as thixotropy (Usmani, 1997) and is a non-Newtonian form of flow because, as previously observed in the flow curves, asphalts have a non-Newtonian flow at testing temperatures. It can also be seen in this diagram that viscosity at 90°C has the expected behavior tendency and it has the following decreasing order for aged asphalts: Apiay > CIB + Aggregate > CAR > CIB.
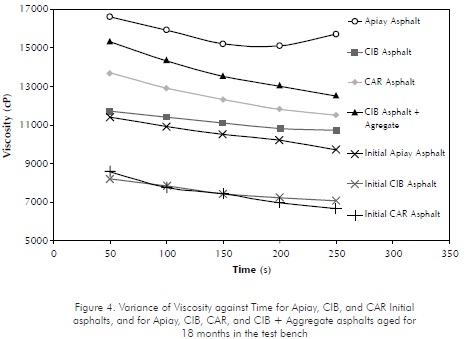
The change of viscosity in time is smaller for the CIB asphalt. This asphalt has not only the lowest viscosity values at low temperatures, but also tends to remain constant after short ranges, as shown in the curves of viscosity versus temperature.
Figures 5a and 5b show the behavior of the complex shearing modulus G* of the initial CIB, CAR and Apiay asphalts, versus the applied angular strain (%). The linear viscoelasticity zone for the initial Apiay asphalt goes from 1 to 15% applied strain applied; 20 to 35% for the initial CIB asphalt and 1 to 20% for the initial CAR asphalt. These results indicate that in each of these intervals, the complex modulus or any other component that is selected, the asphalt does not depend on the level of applied strain. Figure 5b shows that the initial CIB asphalt had one of the highest dynamic modulus for every interval, characterizing it as the most resistant of all of the asphalts under study to total applied angular strain G*.
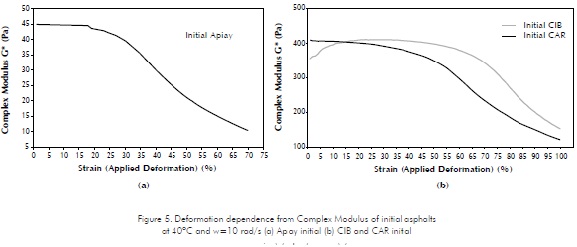
Based on the above figures, the conditions to carry out the temperature sweeping test were chosen for original as well as aged asphalts in the test bank. Figure 6 shows the behavior of the elastic modulus (which indicates how much the asphalt recovers after strain) and the viscous component (global measurement of asphalt resistance to fluence) versus temperature for the Apiay asphalt. The other asphalts had similar behaviors and the figures turned to be similar, so this asphalt was chosen to represent the behavior of the elastic modulus or recovery, and viscosity or resistance.
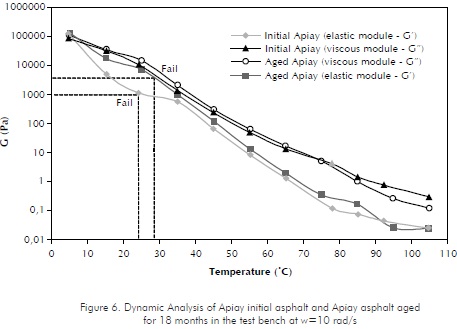
The results corresponding to the other asphalts (Figure 6), with the curves of the elastic component of the dynamic modulus versus temperature of the initial as well as the aged asphalts, were used to determine cracking temperature. Table 3 shows the cracking temperatures that were calculated for the different asphalts considering that G*/sen δ > 1 kPa for original asphalts, and G*/sen δ > 2,2 kPa for aged asphalts.
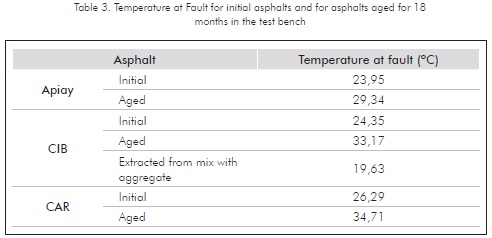
Figure 7 shows the behavior of the dynamic viscosity ETA*(G*/w) versus temperature, determined in the same dynamic shear rheometer (DSR) with a frequency of 10 rad/s. The viscosity curve shows that there is an elastic fluid behavior at low temperatures, followed by a region of pseudoplasticity (c < 1,0), and then an exponential curve reaching a region of high temperatures where viscosity tends to zero.
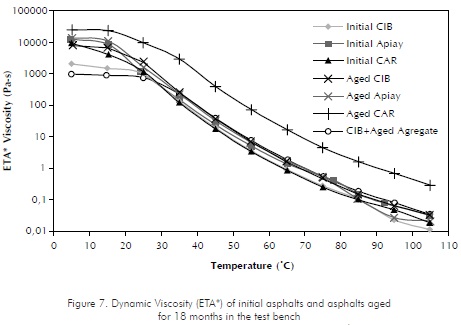
Initial Apiay, CIB and CAR asphalts and the corresponding aged samples report very similar results, so the curves tend to overlap and it is difficult to see significant differences. Nevertheless, the aged CAR asphalt curve is a little distant from the rest, because this asphalt has been affected with a increase in viscosity due to the effects of natural aging.
The aged CIB asphalt has a curve where the variation in viscosity in function of temperature is lower than the other aged asphalts and thus, tends to support less strain and routting during the whole range of testing temperature. The following is the order by dynamic viscosity : Aged CIB < CIB + Aggregate < Apiay < CAR.
The complex shear modulus has been defined as the relation between the shear stress in oscillation applied versus the angular strain, measured as a consequence to the load. Since it is a sinusoidal load, there is a value δ, which is the angle of phase lag between applied torque and angular strain. This parameter reaches a value of 90° when both curves are in phase and the material has a pure viscous behavior. At the other hand the phase angle will be 0°when the material shows a pure elastic behavior. The tangent of the phase angle, called tan δ, is the G"/G'ratio and represents the balance between the energy lost through heat and the energy elastically recovered by strain cycle (Bolzan, 1995).
According to the colloidal model of the asphalt the large micelles of the oil binder structure coagulate or form cells when they are heated. The wax crystals go through changes that affect the colloidal structure, the movement among the molecules increases and there is a change in phase from the vitreous state to a semi-liquid state. It is considered that there is no friction between the molecules and the material goes from a solid to a liquid state at this temperature, known as vitreous transition temperature, which represents change from a state where elastic behavior predominates to a predominantly viscous state. The behavior of the asphalt between these two extremes is viscoelastic (Chaney, 1997).
In order to complement the rheological analysis of the asphalts, a relation is determined for the tangent of the phase angle δ regarding testing temperature, thus determining the temperature of vitreous transition (TTV) for the asphaltic binders by the maximum curve at high temperatures. The results obtained for the asphalts under study are shown in Figure 8. According to this information, the value of tan δ at low temperatures is lower, and the lower this value, the higher the elasticity and strain resistance of the asphalt to permanent strain.
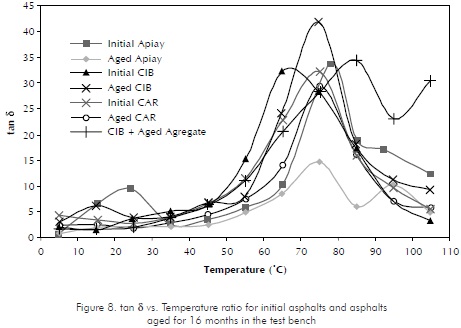
As for initial asphalts, the CIB asphalt has a lower vitreous transition temperature (+ 65°C) compared to the Apiay and CAR asphalts (+74,87°C and +78°C respectively). The aged asphalts behave the same with the following order of vitreous transition temperature (TTV): CIB < Apiay < CAR < CIB+Aggregate, although the differences between the first three are insignificant. This indicates that the CIB asphalt flows faster despite the aging suffered during exposure over a period of 18 months. On the other hand, the CIB + Aggregate asphalt has a complex flow which is the most viscous of all reaching the highest temperatures of vitreous transition (+83,92°C), confirming the significant effect on its chemical and colloidal structure caused by contact with the mineral.
CONCLUSIONS
-
The rheological behavior for the asphalts studied in this paper can be related to the change in viscosity at a broad range of testing temperatures (90-190°C) by implementing the new SHRP technology. It was determined that the lower the handling temperature, the higher the value of the change in aged Apiay asphalt viscosity compared to the aged CIB asphalt and viscosity at 90°C tends toward expected behavior. The following order was established for the change in viscosity for aged asphalts: Apiay > CIB + Aggregate > CAR > CIB. The aged CIB asphalt showed less resistance to flow and lower viscosities in regions near the state of viscoelasticity at temperatures from 60 to 80°C. The sensitivity of the different asphalts when flowing has exposed the adjusted thermal susceptibility that is equivalent to the resistance of the CIB asphalt with or without aggregate at an average temperature, resulting in better rheological characteristics, to be selected as an appropriate binder for pavement in Colombia's tropical climates.
-
Due to the non-linear behavior of flow of all of the asphalts that were studied and at the low values of complex flow index, it was found that at testing temperatures, the type of flow is completely non-Newtonian and the viscosity of asphaltic binders depends on temperature as well as load magnitude and rate. As the flow index decreases in time, the structural complexity of the asphalt increases, which results in a complete change of colloidal state (SOL: SOL-GEL: GEL) or gelification as occurs in CIB asphalt. All of the asphalts studied (CIB, CAR, Apiay and CIB + Aggregate) had a non-Newtonian behavior and a slightly observable thixotropic behavior at testing temperatures at decreased viscosity and shearing stress in relation to time, which is characteristic of aged asphalts.
-
By determining the complex modulus of dynamic shear in function of applied strain, there was a zone of viscoelasticity for the initial asphalts. The modulus of rigidity G'or the elastic component and the modulus of wasted energy or the viscous component G" were determined for all of the asphalts. This determined the resistance to permanent strain and to cracking due to fatigue, respectively. The initial Apiay asphalt has a linear viscoelasticity strain between 1 and 15%; the initial CIB asphalt between 20 and 35%; the initial CAR asphalt between 1 and 20%. The initial CIB asphalt has the highest dynamic modulus, characterizing it as the most resistant to total strain G* of all the materials evaluated in this work.
-
By means of the parameters established for the elastic component of the original and aged asphalts, a cracking temperature was obtained for each one. Any temperature above the cracking temperature will cause the asphalt to suffer permanent strain and to be governed by the viscous component, remaining above the elastic component as temperature rises. Low cracking temperatures such as those undergone by the CIB + Aggregate asphalt (19,63°C) and the Apiay asphalt (23,95°C) mean high thermal susceptibility and sensitivity to premature strain due to high load magnitudes and times. On the other hand, the CIB asphalt with a high cracking temperature (33,17°C) and low susceptibility to temperature is characterized as the most resistant and durable binder. The following is the order of thermal susceptibility for aged asphalts under the tropical climates of Santander: Apiay > CIB + Aggregate > CIB > CAR.
-
The relation between dynamic viscosity and temperature indicated the range of low temperatures (5-25°C) at which asphalts behave as elastic fluids, an intermediate zone of pseudoplasticity and an range of high temperatures (> 80°C) where viscosity tends to reach zero. The descending order of dynamic viscosity after the CIB asphalt is CIB + Aggregate, Apiay and CAR. It was determined that at low vitreous transition temperatures, the asphalt begins to flow faster. This is the case of CIB at 65°C and at high values such as the CIB + Aggregate at 83,92°C , where pour point is more delayed. The aged asphalts show the same behavior with the following order in values for vitreous transition temperature: CIB < Apiay < CAR < CIB + Aggregate, although the first three have insignificant differences among them.
-
According to these results, the CIB asphalt is the most resistant to tropical temperatures and the Apiay asphalt has the highest thermal susceptibility, thus causing that its range of application for different climates is restricted in Colombian conditions.
ACKNOWLEDGEMENTS
The authors would like to thank the "Instituto Colombiano para el Desarrollo de la Ciencia y la Tecnología Francisco José de Caldas - Colciencias" for its support in financing this project.
BIBLIOGRAPHY
Afanasieva, N., 1996. "Envejecimiento de los asfaltos colombianos". XXIX Reunión del Asfalto. Mar del Plata, Argentina, Noviembre 7-14, Memorias, Tomo 1, 111-118. [ Links ]
Afanasieva, N., Álvarez, M. and Puello, J., 1999. "Envejecimiento de los asfaltos colombianos bajo la influencia de los diferentes factores climáticos". Memorias XI Congreso Ibero-Latinoamericano del Asfalto. Sevilla, España, Noviembre 1-6, Tomo I, 83-98. [ Links ]
Afanasieva, N., Álvarez, M. and Serrano, S., 1998. "Diseño de un banco de pruebas para el estudio del proceso de envejecimiento de los asfaltos colombianos". Memorias Primeras Jornadas Internacionales del Asfalto. Piedecuesta, Colombia. Junio 25-27, No. 23, 1-15. [ Links ]
Agnusdei, J., Frezzini, P. and Rueda, E., 1973. "Comportamiento reológico de asfaltos argentinos y sus componentes". XVIII Reunión del Asfalto, Argentina, 1-17. [ Links ]
Agnusdei, J. and Iosco, O., 1998. "Comportamiento reológico de asfaltos a temperaturas elevadas". Memorias Primeras Jornadas Internacionales del Asfalto. Piedecuesta, Colombia, Junio 25-27, No. 10, 1-11. [ Links ]
Arenas, H. and Sanabria, L., 1991. "Análisis físico-químico y reológico del asfalto de Apiay". Memorias VI Congreso Ibero-Latinoamericano del Asfalto. Santiago de Chile, Chile, Noviembre 17-22, Tomo I, No. 2, 1-20. [ Links ]
Bolzan, P., 1995. "Selección del ligante asfáltico más apropiado según las condiciones locales de clima y tránsito de acuerdo con el SHRP". Memorias VIII Congreso Ibero-Latinoamericano del Asfalto. Santa Cruz de la Sierra, Bolivia, Noviembre 12-17, Tomo 4, No. 21, 1-18. [ Links ]
Crawford, W., 1992. "Characterization of asphalt in paving materials". Asphalt Seminar, Petrocanada-Ecopetrol - ICP, pp. a1-f1, d1-d8, e1-e8, g8. [ Links ]
Chaney, R., 1997. "Understanding how dynamic shear rheometry is used to predict performance of paving-grade asphalt cements". A basic Review. Memorias XI Simposio sobre Ingeniería de Pavimentos, Cartagena, Junio 1-5, 184-192. [ Links ]
Kafarov, V., Afanasieva, N. and Álvarez, M., 1996. "Complex system analysis of asphalt pavements". Journal of Polymer Materials, 13: 34-47. [ Links ]
Tia, M. and Ruth, B., 1985. "Asphalt Rheology". Nashville, Ed. Oliver, E., Briscoe, 118-145. [ Links ]
Tuffour, Y. and Ishai, I., 1990. "The diffusion model and asphalt age hardening". Asphalt Paving Technologists, Part 59: 73-92. [ Links ]
Usmani, A., 1997. "Asphalt science and technology". New York, Ed. Marcel Dekker, 11-58, 119-132, 235-247. [ Links ]