Services on Demand
Journal
Article
Indicators
-
Cited by SciELO
-
Access statistics
Related links
-
Cited by Google
-
Similars in SciELO
-
Similars in Google
Share
CT&F - Ciencia, Tecnología y Futuro
Print version ISSN 0122-5383On-line version ISSN 2382-4581
C.T.F Cienc. Tecnol. Futuro vol.3 no.3 Bucaramanga Jan./Dec. 2007
BASEMENT CONFIGURATION OF THE NORTHWESTERN SOUTH AMERICA - CARIBBEAN MARGIN FROM RECENT GEOPHYSICAL DATA
Configuración del basamento de la margen Sur America noroccidental - el Caribe, a partir de datos geofísicos recientes
John F. Cerón1, James N. Kellogg2, and Germán Y. Ojeda3
1Ecopetrol S.A. – Calle 37 No 8-43, Bogotá, Colombia e-mail: jceron@ecopetrol.com.co
2Department of Geological Sciences, University of South Carolina, Columbia, S.C., USA
3Ecopetrol S.A.- Instituto Colombiano del Petróleo, A.A. 4185 Bucaramanga, Santander, Colombia
The oceanic nature of the crust in northern Colombia (underlying the Lower Magdalena Basins) has been postulated by different authors as a northern extension of the Cretaceous, mafic and ultramafic rocks accreted to the western margin of northwest Colombia (in the Western Cordillera and Baudo range). Localized, small outcrops of oceanic affinity rocks seem to support this hypothesis. However, geophysical data do not support this northern extension, but clearly mark the boundary between the collisional Panamá terrane with northern South America and the over thrusting of the latter on top of the obliquely convergent Caribbean plate.
We produced maps to basement and Moho topography by integrated modeling of gravity, magnetics, seismic reflection surveys and well data from northwest Colombia and the southwestern Caribbean. In areas with good seismic coverage, the basement under the Lower Magdalena Basins (LMB) is represented by a clear reflector. In areas where seismic data shows poor imaging or is absent, we use a back stripping methodology to model first the sedimentary section, with known densities, composition and geometry controlled by oil wells and high quality seismic data, and then the deeper section. 2,5D gravity and magnetics modeling results in an initial Moho that can be extended to the entire region based on the control of available seismic refraction points. This controlled Moho provides the basis for basement modeling for the whole area and this sequence is iterated for several sections across the region.
Our results indicate that the crust under northern Colombia is continental to thinned continental (transitional) in nature, with densities between 2,6 and 2,7 g/cm3. Our model also requires a dense wedge of sediments (density 2,5 g/cm3) at the base of the modern fold belt, which may represent a fossil sedimentary wedge attached to the continental margin. This wedge may have served as a backstop for the modern fold belt. The gravity modeling does not require oceanic crust to form the basement in the Sinú and San Jacinto fold belts as previously suggested. Discrete layers and thin slivers of oceanic sediments and basement could have been scrapped off the incoming plate and thrusted into an accretionary mélange, and eventually exposed at the surface, as seen in the Mulatos, Chalan and Cansona locations.
The shape of the continental wedge / oceanic crust boundary resembles that of a very low angle/flat subduction zone (ß angle between 2º to 3º), and is interpreted here as a low angle over thrusting of northern South America riding in a highly oblique direction over the underlying Caribbean plate. The map to basement depth obtained during this study forms the basis for basin analysis, oil maturation and evolutionary studies of the region. As an example, we apply our map to a flexural analysis of the LMB.
Keywords: Colombia, caribbean area, Lower Magdalena Basin, crust, moho geometry, basement, tectonics, geophysical, gravity model, magnetic model, reflection (seismic), flexure.
La naturaleza oceánica de la corteza en el norte de Colombia (subyaciendo las cuencas del Valle Inferior del Magdalena) ha sido postulada por varios autores como una extensión al norte de las rocas Cretácicas, máficas y ultra máficas adosadas al margen Occidental del Nor Oeste de Colombia (en la Cordilleras Occidental y la Serranía del Baudó). Pequeños afloramientos, localizados, de rocas de afinidad oceánica aparentemente confirman esta hipótesis. Sin embargo, los datos geofísicos parecen no soportar esta extensión hacia el Norte, sino que claramente marcan el limite entre el terreno colisional de Panamá con el Norte de Sur América y los sobre cabalgamientos de esta ultima por encima de la placa oblicuamente convergente del Caribe.
Hemos obtenido mapas del basamento y el Moho mediante el modelamiento integrado de gravimetría, magnetometria, sísmica de reflexión y datos de pozo del Norte de Colombia y el Sur Oeste del Caribe. En donde existe sísmica de reflexión la respuesta del basamento es muy clara. En áreas en donde los datos sísmicos son inexistentes o presentan pobre resolución, utilizamos una técnica de reconstrucción para modelar primero la sección sedimentaria, cuyas densidades, composición y geometría son conocidas, y se encuentra controlada por datos de pozos petroleros y sísmica de alta resolución. El modelamiento gravimetrico y magnetometrico en 2,5 D resulta en un Moho inicial que puede ser extendido a toda el área gracias al control de los puntos de sísmica de refracción disponibles. Este Moho controlado provee las bases para el modelamiento del basamento en toda el área de estudio, y esta secuencia es iterada para distintas secciones a través del área de estudio.
Nuestros resultados indican que la corteza bajo el Norte de Colombia es de naturaleza continental a transicional (continental adelgazada). Nuestro modelo también requiere de una cuña densa de sedimentos (densidad de 2,5 g/cm3) a la base del cinturón deformado moderno, el cual podría representar una cuña sedimentaria fosilizada adosada al margen continental. Esta cuña puede haber servido de bloque rígido (backstop) para el cinturón deformado. El modelamiento gravimetrico no requiere que una corteza oceánica forme el basamento en los cinturones plegados de Sinú y San Jacinto como se ha sugerido previamente. Capas aisladas y tajadas delgadas de sedimentos oceánicos pudieron haber sido rasgadas de la placa entrante y cabalgadas dentro de una melange acrecionaria, y eventualmente expuesta en superficie.
La forma de la cuña continental / limite de la corteza oceánica se asemeja a una zona de subducción de bajo ángulo o plana (ángulo ß entre 2º a 3º), y se interpreta aquí como un sobre corrimiento de bajo ángulo del norte de Sur América cabalgando en una dirección altamente oblicua sobre la placa Caribe subyacente. El mapa de basamento en profundidad obtenido durante nuestro estudio forma las bases para estudios de análisis de cuencas, maduración de hidrocarburos y estudios de evolución en la región. A manera de ejemplo, hemos aplicado dicho mapa al análisis flexural del LMB.
Palabras clave: Colombia, área caribe, cuenca del valle inferior del Magdalena, corteza, basamento, tectónica, geofisica, modelamiento gravimétrico, modelamiento magnético, reflexión (sísmica), geometría del moho, flexura.
INTRODUCTION
The present shape of the northwestern corner of South America is the result of the interaction between major plates (Nazca and South America) with the smaller Caribbean, North Andes and Panamá plates and microplates. The Lower Magdalena geologic province in Colombia (LMP) is a geographical denomination to design the sedimentary province north of the northernmost exposures of the Western and Central Cordillera rocks, which serves as the southern limit of the area (Schenk, Viger, & Anderson, 1999). Several sub-basins and folded belts comprise the LMP sedimentary province west of the Bucaramanga and Santa Marta faults (see Figure 1 for location) and East of the Uramita fault, near the Panamá/South America suture zone. The northern limit of the sedimentary province can be extended north to the frontal thrusts of the South Caribbean deformed belt (SCDF). The purpose of this study is to propose a definition of the type of crust underlying the LMP and the implications of the basement configuration, using a geophysical integration approach.
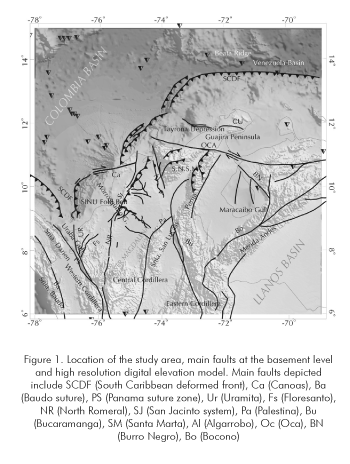
At the western side of the LMP we find the Uraba basin, a flexural basin underlain by the oceanic crust of the Panamá arc (Flinch, 2003). It is genetically and stratigraphically different from the LMP and does not form part of the same. The Uramita fault (interpreted here as a wrench fault with evidence of transpression) forms the geologic limit of the LMP on the west. This LMP comprise (from West to East): the Sinú basin (underlain by thinned continental crust), the San Jorge and Plato basins (underlain by continental crust) and the transtensional Ariguaní basin at the western flank of the Sierra Nevada de Santa Marta massif (See Figure 4b for general location). The Lower Magdalena Basins (LMB) comprise the Plato, San Jorge and Ariguani basins, while the term Caribbean basins has been traditionally used to describe only the Sinu and San Jacinto fold belts (Laverde 2000; Cediel, Caceres, & Shaw, 2003). Basement highs delineate the boundaries of these basins: the North Romeral fault (Cediel et al., 2003) marks the contact between the Sinú and the San Jorge Basin; this last one is separated from the Plato basin by the granitic Magangue high and finally, the Algarrobo strike slip fault created the El Difícil high that separates the Plato from the Ariguaní basin.
The above mentioned basins have been previously described as forearc basins (Ladd, 1984), a foreland basin formed as the result of over thrusting by Caribbean-Arc rocks (Macellari, 1995) or back-arc basins (Flinch, 2003). More recently, some authors; (Hernández and Guerrero, 2006; Reyes, 2000; Reyes, Rueda, Mantilla, & Ardila, 2002) have proposed that these basins are the result of the rotation of crustal blocks that create accommodation space behind them, triggered by the W-E relative movement of the Caribbean plate with respect to South America. The different explanations try to honor the available geologic, seismic, potential fields and well data with the interpretation of the interaction between the Caribbean Crust (CAR) and the northwest corner of the South American Plate (SAM). It must be noticed that there is no present volcanic arc in the LMP and the most recent igneous activity in Northern Colombia is Eocene in age, in the Mande batholith in the northern Central Cordillera and in the Sierra Nevada de Santa Marta (Cediel, Cáceres, & Shaw, 2003).
The north west corner of the South American plate rifted away from adjacent North America starting in the Lower Jurassic, and the opening of the paleo Caribbean ocean created a passive margin that lasted until the Cretaceous (Cediel et al., 2003; Pindell, 1993). Volcanic activity in the Caribbean plate was particularly intense during the 97 Ma and 89 Ma basalt food events (Kerr, Tarney, Nivia, Marriner, & Saunders, 1998; Mauffret, & Leroy, 1997; Mauffret et al., 2001).
The continental crust in North Colombia was stretched, broken and later on subjected to transpressive stresses that deformed the plate and created the LMP and the South Caribbean deformed belts (Audemard, 2002; Larue, 2000). The collision of Cuba with the Bahamas and other global events changed the relative movement of the Caribbean plate with respect to northwestern South America (NW SAM), to near the present ~ 110º convergence direction (Kellogg, Vega, Stallings, & Aiken, 1995; Weber et al., 2001). This convergence has produced the Cenozoic deformed belt in northern Colombia, which contains all the necessary elements (source rock, reservoir, traps and seal) to become an important target for oil exploration.
Because of over thrusting, NW SAM resembles a convergent margin throughout the Cenozoic, and most basins are of the foreland type. We will show that the geometric configuration of the basement in northern Colombia is continental to transitional in nature. We use geophysical tools with control by the available geologic and well information, to derive a crustal model for the NW corner of Colombia, map the configuration of the Moho interface and the geometry of the upper crust (i.e., basement) and then propose a model for the formation of the LMP basins.
The methodological approach is as follows: seismic reflection data provides the basic framework for the mapping of the basement, with control of oil well data. In areas where seismic imaging is not clear or we lack seismic control, we use a integrated approach to invert the gravity signal to obtain maps to basement depth. We start with a discussion of the compilation of the gravity maps for north Colombia using all new and historical data, including a discussion of the processing sequence. This forms the basis for 2D gravity modeling using new seismic reflection data as the control for the geometric modeling of the upper crust. The results of these 2D models are used to extend our knowledge of the Moho configuration, with the aid of seismic refraction surveys. Seismic data provides the basis for basement mapping, with control by surface geology and oil well data. Once we have a good control of the Moho in 3D, we can use this signal as a "regional" to model the gravity field over regions where we don't have good seismic or geological control, to infer the geometry of the basement. Finally, we use the interpreted geometry of the basement to start a discussion about the genesis of the Lower Magdalena geologic province (Sinú, San Jorge, Plato and Ariguaní).
THE COMPOSITION OF THE CRUST
The composition of the crust across the northern margin of South America (SAM) changes northward from continental to oceanic (Caro, 2003; Flinch, 2003; Mantilla-Pimiento, Alfonso-Pava, Jentzsch, & Kley, 2005) (Russo, & Speed, 1994). In the early Jurassic, the Pangea continental crust broke up, and SAM rifted away from North America. The stretching of the continental crust must have created zones of weakness, similar to the present day West African and Brazilian margins. In those rifted margins, there is a continuity from continental to oceanic crust, with a "transitional" or "continentalized" crust in between. Alternatively, a fossil spreading center may have existed in the Proto-Caribbean until the cessation of the separation, around the end of the Early Cretaceous. From that epoch to the present the northwestern margin of South America has been characterized by compression, divergence, and transpression.
The most accepted model for the origin of the Caribbean plate is as an allocthonous provenance drifting from Pacific sources (Levander et al., 2006; Pindell, 1993; Pindell, 1994; Rogers, 2003). This model leaves open the possibility that fragments of Proto-Caribbean crust may be preserved along the ocean-continent boundary.
The western Cordillera in Colombia consists of several belts of Cretaceous, oceanic affinity rocks accreted to the SAM margin during the Upper Paleocene to Lower Eocene (Kerr & Tarney, 2005; Nivia & Gómez, 2005). Mafic and ultramafic rocks were accreted to the west of the paleosuture zone (Kerr, Tarney, Kempton, Pringle, & Nivia, 2004; Nivia, 1996). Similar composition rocks are found in Panamá (Case, Moore, Durán, & López, 1971), in the Azuero peninsula, and other locations of Central America, around what has been called the Cretaceous Caribbean Igneous province (Donnelly et al., 1990; Kerr, White, Thompson, Tarney, & Saunders, 2003; Lewis, Draper, Proenza, Espaillat, Jiménez, 2006). Irving (Irving, 1975) suggested a separation between oceanic and continental crust in the Lower Magdalena basin and after that it is a common theme to read about the "oceanic crust in northern Colombia". This seems to be confirmed by surface exposures in localized outcrops of ultramafic rocks south of Montería (Cerro Matoso, El Almendro, Santa Rosa, Ure) located at the northern alignment of the North Romeral fault, small outcrops of basalts (in the Chalan anticline and the Ojo seco volcanics in Cerro Cansona) and basaltic fragments found at the well Tolú-6 (near the coast at Morrosquillo embayment).
In addition, seismic imaging west of this alignment (like in the Puerta Negra-1 well in southwest Sinú), displays very poor to null resolution of the subsurface layers. Then, it seemed natural to trace the oceanic-continental boundary North of the western cordillera in Colombia, following the alignment of the Romeral wrench-thrust fault system. Based on the qualitative interpretation of the magnetic intensity and gravity maps, we propose that the basement (upper crust) on this area is of continental composition in nature, and oceanic basement rocks have been emplaced tectonically by NW verging thrusts that have been scrapped off the over thrusted Caribbean plate or are part of fragments of obducted blocks. The crustal lithosphere is thinner than normal continental crust, and for that reason we prefer the term "attenuated crust" to describe the wedge shaped continental crust at the northern boundary of SAM.
The nature of the oceanic crust
The Colombia Caribbean Basin comprises the oceanic basin bound by the Hess escarpment on the west and the Venezuela Basin on the East (connected across the Aruba Gap, south of the Beata Ridge). One goal of this work is to clarify the nature of the crust at the ocean continent boundary, since it has been the origin of debate in many publications.
The crust of the Colombia Caribbean basin has been directly investigated by the Deep Sea Drilling Program (DSDP). Site 153, drilled at the Beata ridge in waters of 4046 meters depth, encountered a Cretaceous (Coniacian/Campanian) to Pliocene sedimentary section overlying Cretaceous age crystalline basement (basalts, dolerites, diabases). Seismic reflection data (Driscoll & Diebold, 1998; Mauffret et al., 2001) tied this site to the continental margin of Colombia, providing the opportunity to understand the nature of the crust near the continental shelf.
In the Venezuela Basin, the crust is three-layered (Diebold, Stoffa, Buhl, & Truchan, 1981) with a 6,0/6,3 km/s layer sandwiched between a 3,2 to 5,0 km/s and a 7,0/7,3 km/s layer, similar to the Colombian Basin crust. James (2005) points to the similarity with the Grenada Basin, which is also three-layered with velocities of 5,3, 6,2 and 7,4 km/s.
Seismic line Conrad-132 in the vicinity of DSDP 153 depicted reflectors A" and B" (Baquero, 1983). The reflector A" was identified from the JOIDES drilling as a sequence of early Eocene sediments composed of fine chalk or limestones interbedded with cherts (Edgar, Ewing, & Hennion, 1971). Overlying it are unconsolidated oozes and other sedimentary lithologies. The smooth reflector B" is near the contact between a chert or siliceous limestone of Late Cretaceous age (Coniacian to Campanian) and a sequence of basalts, dolerite or diabase sills (Edgar et al.,1973). Ladd et al., (1984) proposed that basalts of the B" reflector are exposed in Curacao, where Venezuela Basin crust was uplifted and exposed. In Colombia, the A" reflector outcrops in the San Jacinto fold belt, where Duque-Caro has interpreted it at the upper San Cayetano Formation (Duque-Caro, 1997b).
Sub-B" reflections were noted by Diebold et al., (1981) in the Aruba Gap near DSDP 153. They described it as a "diffuse" discontinuous relatively subhorizontal event, with an interval P-velocity above 5 km/s. Based on this high velocity and its seismic character, they proposed an igneous origin. From refraction data Edgar (in Ludwig, Houtz, & Ewing, 1975) reports values from 3,2 km/s to 4,2 km/s for the material above B" and values as high as 5,2 km/s for B". At DSDP 146/149 in the Western Venezuela Basin, Diebold et al., (1981) correlated the "smooth" reflector B" with Coniacian to Campanian tholeiitic basalt flows and dolerite sills interbedded with pelagic limestones. Bowland, & Rosencrantz (1988) suggested that the Upper Cretaceous basement below seismic horizon B" in the Venezuela Basin is similar to the smooth-surfaced basement in the Colombian Basin, and calculated velocities of 4,5 – 5,5 km/s for this layer, indicating an igneous composition. Leg 15 of the DSDP reported a P wave velocity of 4,9 km/s for these basalts. These values agree with the refraction values tabulated in Table 3.
The basaltic Aruba Lava Formation is part of the Cretaceous (88 - 91 Ma) Caribbean oceanic plateau, interpreted as originating in the Pacific. The thick succession of submarine-to-emergent volcanic and volcaniclastic rocks on Aruba suggest an intra-oceanic origin. Petrographic, geochemical and isotopic data are consistent with derivation of the Aruba Lava Formation from a mantle plume (Kerr et al., 2003). In the Aruba gap, a prominent reflector denominated "Hopkins reflector 5' (Hopkins et al., 1973) was identified as middle Miocene. Marine seismic data shows that the Middle Miocene is a prominent unconformity on the Pacific side of continental Colombia. Duque-Caro (1990b) noted that in the Sinú Basin, the 15,5 Ma (Middle Miocene) unconformity has a regional character. Laverde (2002) named this event the 'Sinú accretionary event". It has been proposed that this surface represents the maximum flooding surface (m.f.s) in the Lower Magdalena Basin. The continuity on a regional basis of this reflector can not be overlooked, but we postulate here that it represents uplifts in the northern block of South America, possibly caused by the collision of the Panamá Arc with the South American plate, which started in the Miocene and continues to the Present (Kellogg et al., 1995).
The composition of the continental basement
Continental crust-type basement has been drilled in wells of the Cicuco Field on the Magangué High. Granodiorites with K-Ar ages from 102,6 – 117,8 Ma indicate an Early Cretaceous intrusive event (Maya, 1992). During the Late Cretaceous several intrusive granitic bodies including the Antioquia Batholith (González, 2001)) were emplaced in the Central Cordillera. Large granitic boulders are found in Eocene-age conglomerates near Ovejas (Henao, 1961), in the Tolú-6 well interstratified with Lower Tertiary sediments (Reyes et al., 2001).
Numerous oil wells drilled in northern Colombia have penetrated crystalline basement. Figure 2 presents a synopsis classified by the lithology encountered in the basement. Triangles depict wells drilling low grade metamorphic rocks (schists, phyllites), circles show wells penetrating granitic rocks, while stars indicate unique lithologies. Of these, three deserve special attention. The Medialuna-1 well encountered granites, metamorphic, and gabbroic basement described by Petrochac (2001) as ophiolites. The Remolino Grande well near Luruaco also penetrated mafic rocks and the Manantiales well in the Guajira Area (northernmost star on the map) drilled spilites. Reyes et al., (2001) described samples from the Tolú-6 well as "lithologies with oceanic basement affinity". South of the San Jacinto fault (Figure 1) all the wells penetrating basement encountered felsic or metamorphic rocks.
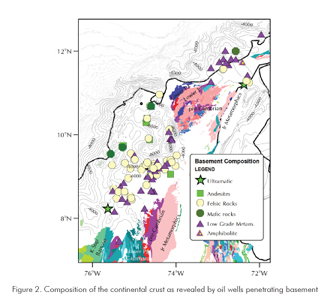
North of this fault, in the so-called San Jacinto fold belt (Duque-Caro, 1979; Duque-Caro, 1984; Guzmán, 2003) mafic and volcanic rocks of Cretaceous age outcrop in the Cansona uplift, the Chalan Anticline and several quarries, such as San Carlos and San Sebastian. An accretionary wedge has been proposed to explain the Sinú-San Jacinto sedimentary sequence in northern Colombia (Duque-Caro, 1979), and the Lara Nappes in Venezuela (Escalona, 2006) are a Paleogene example of oceanic crust over thrusting continental crust.
Repeated episodes of arc magmatism can produce continental-type felsic to intermediate upper crust while arc magmatism produces a thicker mafic lower crust than the average continent. In the Aleutian island arc, the excess mafic material in the island-arc crust can also be attributed to preexisting oceanic crust. (Fliedner, & Klemperer, 2000).
Important unique lithologies in the LMP are the peridotitic outcrops in Paraiso, Montelíbano, Planeta Rica and El Almendro, all along a narrow ridge south of Montería. These mantle-derived ophiolites are dated as Early Cretaceous by Guzmán (2003). Peridotites are known in several locations around the Caribbean, and in Colombia we interpret those discrete outcrops as slivers of mantle rocks obducted to the continent during collisions of previously detached continental blocks separated from the autochthon during the rifting periods. Gravity modeling of these bodies demonstrated their localized effect, and they appear as rootless bodies emplaced on top of continental to transitional crust.
In summary, where outcrops or well control exist the crust of the LMP can be described as continental in nature, and the few exceptions need to be explained in the regional tectonic framework.
The sedimentary column
Late Cretaceous (Cansona cherts and pelagic sediments) to Neogene sediments fill the depocenters in the LMP. Based on published works (Caro, 2003; Cediel & Caceres, 2000; Cediel et al., 2003; Diaz, & Ramos, 2003; Duque-Caro, 1984, 1990a, 1990b; Duque-Caro, 1997b; ESRI-ILEX, 1995; Laverde, 2000; Schamel et al., 1998) we compiled the main facts about sedimentary sequences, facial changes, density contrasts and paleogeographic implications for the purposes of our modeling.
From the stratigraphic works by Duque-Caro (Duque-Caro, 1997a) and Laverde (Laverde, 2000), we selected the main unconformities observed in seismic data, assigned ages according to the mentioned authors, and on the basis of the average composition made an approximation to the percentual lithologic composition of sandstone (Ss), shale (Sh), limestone (Lm) and Chert (Cht) for basin decompaction purposes. The values are shown in Table 1. The last column depicts the density variability for each layer for gravity modeling purposes, based on density logs, lithology, depth to top of layer and the functions described later on. Offshore, we followed the reflectors in the deep seismic lines that tie the Colombia basin with the DSDP 153 site.
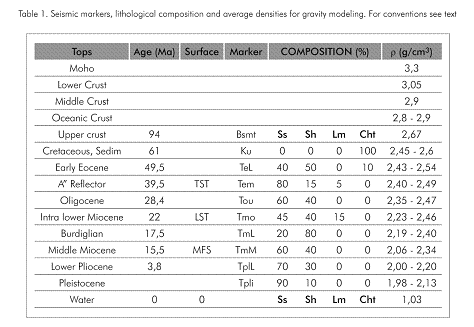
The middle Miocene unconformity (TMm), is an important marker from the Pacific side of Colombia (where it represents a major uplift and erosive event) to the Guajira basins, passing through the Urabá sub basin and Lower Magdalena basins. It represents an epoch of intense tectonic activity in the entire Caribbean.
GRAVITY COMPILATION
Gravity data
Gravity data acquisition in Colombia can be traced back to the late 20's, when several surveys using torsion balance were recorded in the Middle Magdalena Basin. The exploration of the Lower Magdalena Basin onshore includes the oldest oil well drilled in the country (Las Perdices-1, drilled by Texaco in 1906) and the first aeromagnetic acquisition carried in Colombia in 1947. We have included in our study gravity maps from the area circa 1943.
One of the oldest compilations was performed by J. Henao in 1961 integrating the 1940-1941 campaign by Mott-Smith, a 1947 Shell survey in the Tacamocho-Zambrano region, Petty's survey in Cicuco in 1956, a Coveñas-Montería survey by Gravity meter Exploration for Texas Oil in 1943-1944 and the 1959 Gravity compilation published by the Geographical Institute. The relatively flat topography and pronounced density contrasts that revealed basin configurations led to the acquisition of numerous surveys, and more recently aerogravity from fixed wing platforms (not included in our compilation). In 1991, Kellogg and others published simple Bouguer and free air maps based on a compilation of open file data for Colombia, Panamá, and adjacent marine areas. The most complete compilation to date was the GETECH SAGP project (Green and Fairhead, 1993) when world wide available databases, digital and analog maps and tables were compiled into a single product, where we collaborated with Derek Fairhead and his colleagues for the compilation. The SAGP did not put too much effort to bring to an unique datum the individual surveys, and for that reason we recompiled the most significant surveys, put them into a single datum and reprocess the entire region. Table 2 summarizes the surveys used in the compilation.
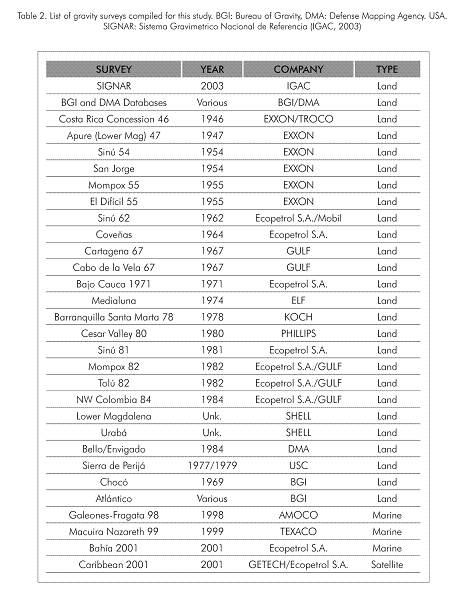
Not described in Table 1 are the compilations available at the BGI, the DMA (USA) and Ingeominas in Colombia along main roads, for a total of about 16500 land gravity stations, north of Lat. 7º N.
Offshore data
For the near shore area, marine gravity acquired along seismic surveys was incorporated as available. In addition, BHP-Billiton acquired in 2006 the Fuerte survey and CGG acquired the Colombia Delta in 2006 but they were not used in the compilation due to confidentiality terms. The marine surveys lack the principal facts control of land surveys and must be adjusted by leveling in order to maintain internal consistency.
For the offshore areas beyond the continental shelf, we used a grid of satellite-derived Free Air gravity, commissioned to GETECH, University of Leeds (UK) by Ecopetrol S.A. A proprietary re-picking technique was employed by GETECH using the GEOSAT and ERS-1 geodetic missions, which yields an approximate equatorial track spacing of 5 km, while the sampling along the orbit ground track is 350 m for the ERS-1 mission and 700 m for the GEOSAT satellite. The resulting product was compiled on a grid of 2 km by 2 km. (GETECH, 2001).
We are aware of the existence of a sea bed gravity survey along the coast of Colombia, but it was not used in the present compilation.
Processing
During the University of Leeds compilation of gravity data for South America (Green & Fairhead, 1993) it became evident that several files used in the compilation, containing data from the BGI, USGS, DMA and others, included duplicate stations but the labeling system often prevented identification of the duplicate stations. The principal facts for the gravity land stations were not consistent, which resulted in the same station displaying highly different values of FAA, elevation and CBA. Very common as well is a station with the same elevation and principal facts, reported at different locations.
The final SAGP result, therefore, is contaminated with high frequency noise resulting from the original data itself. On a regional grid of the order of hundreds of kilometers, these artifacts are often not noticeable. But for detailed work, for instance when performing gravity profiles for 2D modeling, this dataset is not enough, because variations in gravity values are commonly on the order of 16 mGal, with an RMS of +/- 4 mGal which results in undesirable noise.
In 1995 IGAC (Instituto Geográfico Agustín Codazzi, Colombia) undertook the definition and updating of a new gravity datum for Colombia, SIGNAR: Sistema gravimétrico Nacional de Referencia (Martínez, Sánchez, & Flórez, 1995; Sánchez, 2003). The main objective was the determination of a Geoid for Colombia, and the new gravity datum was made available to Ecopetrol in 2006. We undertook the effort of leveling each individual survey relative to the new SIGNAR datum, since very often we ignore the actual tie stations to the main network. We used a least squares approach to remove a first order function that best adjusted the entire survey to the IGAC network. A flat, dipping plane was adjusted to each individual survey, honoring the main facts of the gravity stations belonging to the main datum when available. The process was lengthy and we had to discard spurious stations that introduced errors beyond an acceptable level. The final product resulted in a unified database where the observed gravity showed a more consistent trend.
A similar approach had been used by IGAC (Sánchez, 2003) adjusting individual surveys to the datum by means of the RMS error, but their result did not include all the available stations and we noticed a higher order of adjustment in the leveling. For that reason we did not use their extended database, beyond just the first, second and third order stations.
Free air anomaly onshore was merged with the grids for Free air anomalies offshore to produce a leveled, FAA/FAA map, shown in Figure 3. The straight feature near the NW corner of the map corresponds with the Hess escarpment, where more than 1000 m of vertical relief exists. The Free air anomaly offshore is highly affected by the bathymetry and the low densities of unconsolidated sediments on the ocean floor. A 3D Bouguer correction can be used to remove the terrain effects. Since this map is a compilation of several surveys over a large area, it is difficult to find a single density that best fits the entire region. In addition, for comparisons, most publications show the Free air anomaly offshore. For the onshore portion, however, the complete Bouguer anomaly (i.e. terrain corrected) best shows subsurface density variations and basin boundaries.
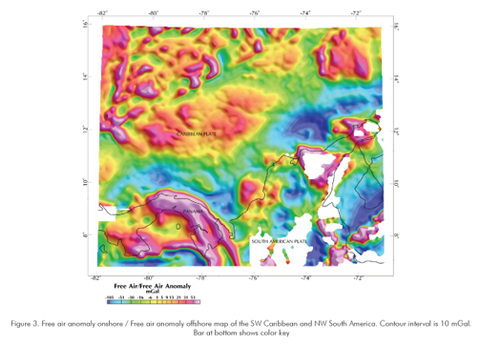
As an example, we used reduction densities of 2,6 g/cm3 onshore and 2,2 g/cm3 offshore to produce the anomaly map shown in Figure 4a: Complete Bouguer onshore, Complete Bouguer offshore - CBA/CBA and the residual "isostatic" gravity anomaly with the structural elements within the Lower Magdalena Basin superimposed. The above mentioned values are the densities that best minimize the topographic and bathymetric effects on the observed gravity field of the LMP.
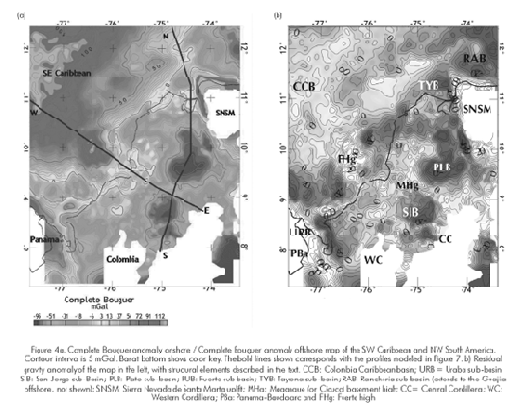
GRAVITY MODELING
Gravity data modeling in 2 ½ dimensions allowed us to test different scenarios for the density contrasts in the upper crust. For a gravity modeling sequence we require: a) an observed gravity field, b) a density model and c) an initial geologic model.
Observed field
We choose the complete Bouguer anomaly (reduction density of 2,6 g/cm3) onshore / free air anomaly offshore for our modeling. We produced maps of Free air onshore / Free air offshore as well as complete Bouguer / complete Bouguer onshore and offshore. The selection of one set or the other defines the modeling surface, background density and density above the datum to be used. For our case we placed the gravity stations on top of the terrain boundary (level of observation) and we used a background density equal to the reduction density (2,6 g/cm3). This last value was obtained after numerous trials to define the reduction density that minimizes the topographic effects.
Density model
The quantitative interpretation of the gravity field requires a density model that actually reflects the density contrasts in-situ. We divided the density model in two parts for this study: the density of the sediments and the crustal basement densities. As a starting point we used the densities as logged in oil wells. From the stratigraphic column we have a record of sediments spanning the late Cretaceous (cherts and siliciclastic rocks of the Cansona Formation) to modern, unconsolidated deposits.
The sedimentary density distribution is more affected by burial than lithology as normally expected (with the important exception of carbonates, which show densities on the order of 2,6 – 2,7 g/cm3). Geologic formations presented a broad range of density variability from well to well, but this range converged when we plotted the available data against depth, regardless of lithology.
The result is shown in Figure 5, where scattered points represent Bulk densities (in g/cm3) from the FDC or CDL logs (already corrected for mud cake). The thick line represents one of several best fits; this particular one has the form:
Where:
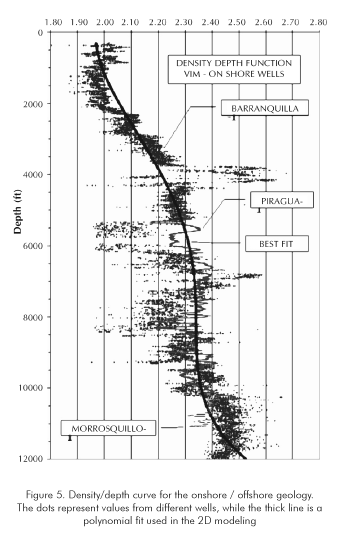
Densities of the crustal layers are evaluated in two steps. From density logs, we can estimate the average density of the crystalline basement. The well Cicuco-Este-1 drilled 170 ft of granitic basement, with a density of 2,66 g/cm3 as shown in Figure 6. The measured value is very close to the typical reduction density of 2,67 g/cm3 (Chapin, 1996) and represents the density of the basement (or upper crust layer in our model).
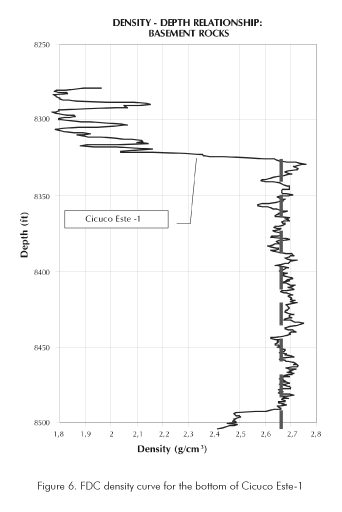
The crystalline lithosphere in northern South America may be represented by a layered model composed (from top to bottom) of upper, middle and lower crust, above the lithospheric mantle. A first approximation is available from seismic tomography by the CRUST 5.0 and 2.0 databases (Bassin, Laske, & Masters, 2000; Mooney, Laske, & Masters, 1998) which provide values gridded at 2 by 2 degrees of the Moho, lower, middle and upper crust and hard sediments.
Refraction studies provide also a semi-direct measurement of compressional velocities (Vp) which, in turn, can be correlated with densities. Recent refraction studies have been conducted along the Pacific coast of Colombia (Agudelo, 2005; Marcaillou, 2005), and in the Venezuelan Caribbean Basin (Diebold et al., 1981; Guedez, Magnani, & Levander, 2006; Mauffret and Leroy, 1997; Mauffret et al., 2001; Schmitz, Martins, Jácome, Sánchez & Rocabado, 2005). Older refraction campaigns remain the only source of information for the Colombia Caribbean Basin (Houtz and Ludwig, 1977; Ludwig et al., 1975; Case, 1975; Case, MacDonald, & Fox, 1990; Edgar et al., 1971). Onshore refraction surveys in Colombia include the Nariño I,II and III projects near the border with Ecuador (Meissner, Flueh, Stibane, & Berg, 1976) and the Choco transects by Flueh (Flueh et al., 1981). Table 3 displays a compilation of some of the reported values, to show the general tendency in Vp of the earth layers.
While the compressional velocity (Vp) values are often measured directly, most of the densities in this study have been derived from empirical relationships. One of the most well known relationships between density and Vp is that of the Nafe-Drake curves (Nafe, 1963), which have recently been offered in the form of polynomial approximations by Brocher (Brocher, 2005) valid for compressional velocities between 1,5 and 8,5 km/s:
Where:
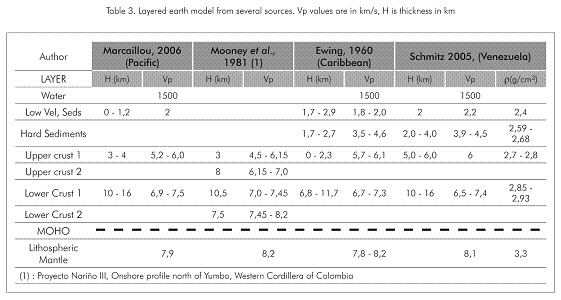
Initial geologic model
Abundant seismic reflection data from oil exploration covers the LMP. We had access to full datasets of SEG-Y data and interpretation software, and selected lines were chosen along regional transects. The structural interpretation (in time) provides an initial geometrical constrain for the gravity modeling, with ground control from well logs. Oil industry biostratigraphic interpretations are used to establish the age of the horizons depicted in the interpretation. However, the seismic reflection data provides little or no information about the middle and lower crust. Figure 7a shows a seismic reflection profile across the San Jorge Basin near the Chinu fault and the locations of three wells that drilled crystalline basement, San Jorge-1, Tirón-2, and Magangué-2. Basement rocks included felsic igneous and metamorphic rocks.
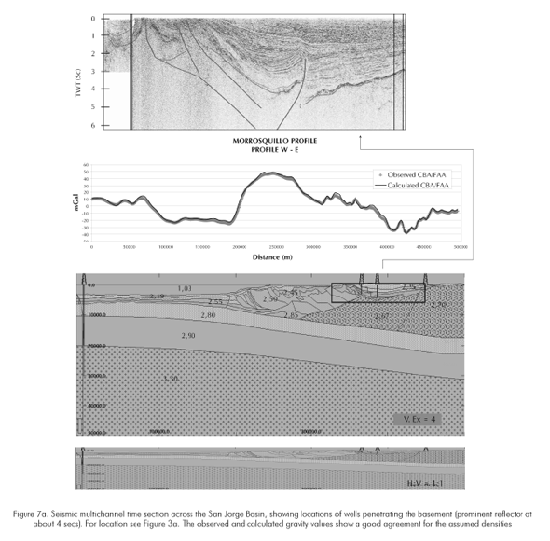
The seismic reflection data in time was converted to depth using a combination of interval velocities (derived from the migration velocity field) and published data for convergent margins. Velocities extracted from the available refraction data (Case et al., 1990; Ludwig et al., 1975) served as independent control.
Based on seismic observations offshore of the Colombia-Ecuador trench (Agudelo, 2005; Marcaillou, 2002), in the Colombia Caribbean Basin (Case, 1975; Edgar, et al., 1971) and in the Venezuela Caribbean Basin (Levander, et al., 2006) we built a layered model for the oceanic crust containing an upper layer with a velocity of 5,7 to 6,1 km/s and a lower layer with velocities between 6,7 and 7,3 km/s. Seismic refraction profiles No 17 and 18 (Edgar, 1971), and profile B (Houtz, 1977) acquired in the Colombian Basin found an upper layer of oceanic crust with velocities between 5,8 and 6,1 km/s and thicknesses up to 2300 m. The oceanic crust underlies layers with velocities of 3,5 to 4,6 km/s. These are too high for pelagic, unconsolidated sediments, so probably they include sediments with a high percentage of carbonate composition. For comparison, the Nazca plate oceanic crust, subducting near the border between Colombia and Ecuador, has shown refracted velocities between 4,5 to 6,2 km/s for an upper crust 2-3 km thick, and between 6,2 to 6,5 km/s for a lower oceanic crust with a thickness between 4 to 6 km (Agudelo, 2005).
The crustal models (Bassin et al., 2000; Mooney et al., 1998) provided the initial geometric model used for the middle and lower crust. The resulting gravity model for two profiles (CBA/FAA anomaly) are shown in Figure 7.
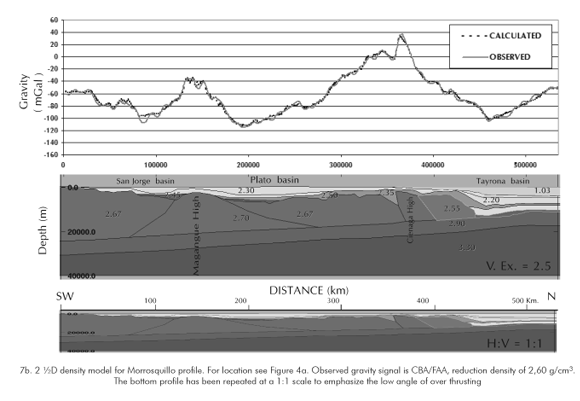
Gravity response of the moho
Through 2D modeling, we evaluated the contributions of sedimentary layers to the gravity field. Seismic data provides control on the geometry, while body densities can vary within a narrow range. The name of the game here is density accuracy. However, the rules of the game to be defined in advance include both the regional/residual separation and the geometric model to be tested. Along profiles where no seismic control exists, we must have some a priory knowledge of the "regional" field to minimize the degree of uncertainty.
The Bouguer gravity field measures the effects due to all sources within the earth. In applied geophysical exploration it is customary to separate the signal in a residual, this is, the anomaly of interest relevant to the geologic goals of exploration, and a regional: the superposition of all sources deeper than our targets. In our approach, the full anomaly is modeled without regional/residual separation in order to model the response at crustal scales.
Residual anomaly separation involves subjectivity that makes model comparisons difficult (Chapin, 1996; Karner, & Watts, 1983; Spector, & Grant, 1970; Yuan, Sobolev, & Kind, 2002). Therefore we would like to establish a separation method that can be reproduced elsewhere.
Interpreting the first order 3D gravity signal requires an area large enough to register the long wavelength signals including that of the crust-mantle boundary. Maps of the Moho in the Caribbean Basin (Case et al., 1990; Feng, S. van der Lee, &, Assumpcao, 2007) suggest that the Moho discontinuity dips gently to the SE, and varies in depth from 25 km in the middle of the Colombia oceanic basin to approximately 43 km under the continental Lower Magdalena Basin (Flueh et al., 1981).
Jacobsen (1987) proposed using upward continuation as a standard suboptimal filter. To extract sources below a certain depth (Zo) he suggested an upward continuation of 2 Zo above the measurement plane. Thus, if we assume a thickness of the upper crust between 9 km to 25 km, an upward continuation to a 50 km plane should reveal the deepest upper crustal signals.
Spectral methods are common as a first proxy for regional/residual separation. The Spector and Grant (1970) depth estimation methodology assumes that, for large samples, in a radially averaged plot of the logarithm of the power spectrum (E) vs. the wave number (rad/km) the depth to the causative sources can be approximated by a straight line for an ensemble of blocks at approximately the same depth (h), and the slope of the line is -2h. Figure 8 shows the Free air anomaly map of northwest South America, from 77º W to 67º W and from 14º S to 12º N. The map was produced combining the Free air anomaly map of Colombia obtained in this study with Free air anomalies obtained from repicking of the satellite derived gravity (GETECH, 2001). Where necessary, like the Panamá isthmus, the map was completed using public domain data included in the DNAG geophysics of North America compilation (Hanna et al., 1989). With the exception of the DNAG data set (6 km by 6 km grid) all the other grids were produced with a resolution of 2 km by 2 km.
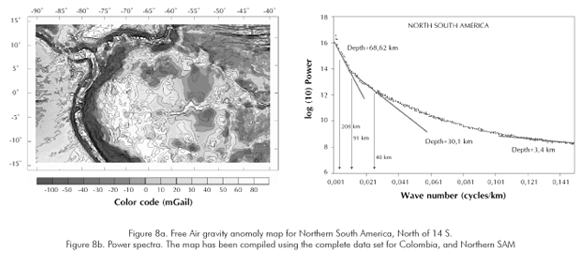
The radially averaged power spectra of the above grid is shown in Figure 8b. The power spectra shows a linear trend between wavelengths of 40 km and 91 km (depth estimate of 30 km), a shallow trend with a depth estimate of 3,4 km (below wavelengths of 4,8 km), and a deep source with estimates around 69 km, between wavelengths of 91 and 200 km. The meaning of these depths cannot be uniquely determined, but comparison with similar datasets (Chapin, 1996; Karner, & Watts, 1983) suggests that the 30 km pick is an expression of the average depth to the Moho discontinuity. The shallowest depth estimate (3,4 km) may represent an averaged depth to crystalline basement. The deepest value (69 km) is consistent with deep discontinuities found by Dave Chapin (1996) and Karner and Watts (1983) for both oceanic and continental lithosphere. The Nariño Project (Meissnar et al., 1976) discovered an increase in the Vs and Vp velocities at approximately 69 km depth for a refraction line between La Cocha (1º N, 77º W) and Bogotá (4º N, 74º W). Based on spectral separation at different scales for the South American, Caribbean and Nazca plates, we speculate that the 69 km depth represents a major lithospheric upper mantle discontinuity.
For the Colombia Caribbean basin and the adjacent Lower Magdalena Basin in Colombia between 7º N and 16º N and between 71º W and 82º W, the deepest depth estimate (~ 30 km) is characteristic of average Moho depths, while shallower estimates (~ 9 km) are related to the top of crystalline basement, independently controlled by seismic, well, and gravity modeling in the Plato, San Jorge, and Tayrona basins. The University of Leeds South American free air gravity dataset exhibits a power spectrum (see Figure 3 in Chapin, 1996) with two clear slope breaks, the first at the 125 km wavelength and the second one at 315 km. Chapin's explanation for the last one is that at longer wavelengths, the free air gravity is dominated by isostatic effects.
Using this spectral separation approach, we produced an initial model in 3D using wavelengths longer than 5 km and shorter than 120 km as the observed field, and a simplified geologic model consistent of low pass filtered topography, bathymetry, a first approximation of the base of sediments as mapped with seismic data and a flat Moho surface that is inverted to match both the observed field and the refraction data control points. We conclude that the spectral methods provide a good approximation for the configuration of the Moho in Northern South America, using Butterworth filters. The oceanic Moho is within a depth range of 20 to 25 km, with good control from seismic refraction data, while the continental Moho ranges from 27 km to 45 km, the deepest values under the Eastern Cordillera / Mérida Andes orogens. Control points onshore are available in Venezuela (Schmitz et al., 2005)) and west Colombia (Flueh et al., 1981) The obtained depth to Moho map is show n in Figure 9. In general it compares closely with both the Case (Case et al., 1990) and the CRUST 2.0 model, (Bassin et al., 2000) and honors the available refraction data.
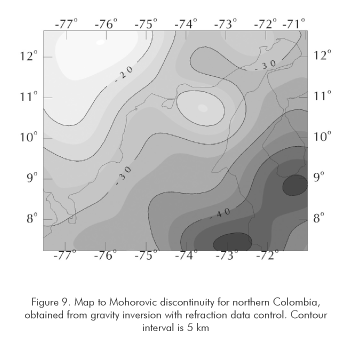
BASEMENT GEOMETRY
The term "geologic basement" is used here in the sense of the top to crystalline (metamorphic or plutonic) rocks overlain by sedimentary beds. The basement offshore would correspond to the equivalent, non-sedimentary rocks at the base of the sedimentary column. In the case of the Lower Magdalena province we must distinguish between continental basement (or continental upper crust) and oceanic basement, different in age, composition and geometry. From the geophysical point of view, we must also make a difference between the acoustic, the dense and the magnetic basement, depending on which physical property we measure (acoustic impedance, density or susceptibility, respectfully). They do not map necessarily to the same surface, and during the modeling we allow some adjustments between "basement" drilled by oil wells (crystalline basement) and the surface represented by geophysical models.
The qualitative interpretation of the gravity anomaly of the Lower Magdalena show features that can be correlated with known geo phenomena in the region, as shown in Figure 3b. From South to North, we notice low values associated with depocenters (sub-basins) and high gravity values associated with the Serranía de San Lucas crystalline outcrops, with the Panamá-Baudó oceanic arc (Case, Braunstein, & Thompson, 1982; Case et al., 1990) and the Luruaco area.
The depocenters can be successfully delineated with the help of 2D and 3D gravity modeling, especially when well control exists (as is the case for the Plato and San Jorge basins). Depocenters with less control and a higher level of uncertainty include the Sinú, Bahía, and Tayrona basins.
We use the cooperative approach to perform "backstripping" of the gravity signal, starting with the estimated Moho, and then producing maps of depth to basement. Drilled wells and 2D seismic profiles provide boundary conditions for the 2D modeling. This controlled Moho provides the basis for basement modeling in areas where seismic data shows poor imaging or is absent, and this sequence is iterated for several sections across the region. The resulting product is shown in Figure 9.
The Plato and San Jorge depocenters, as well as the lineament North of the San Jacinto fault (so called the "Bolivar High") were recognized since the initial surveys by Mott-Smith in 1941. Structural phenomena like the Remolino High, Magangué Arch, Tablón High and El Difícil High had also been mapped by Henao (Henao, 1961). The "Tolú Viejo" gravity high from South Coveñas to the North into the Gulf of Morrosquillo was important in the location of the Tolu wells in 1949. A second maxima south of Lorica marks the Magangue (or Cicuco) high. The 2D model shown in Figure 7 requires a sliver of higher density sediments (2,5 g/cm3) at the base of the deformed belt in front of Morrosquillo. The body has a positive gravity and magnetic anomaly associated, but has not been sampled.
Our interpretation of the depth to basement (onshore) is shown in the map in Figure 10. Basement reaches depths of 9 km in the Plato Basin, and north of it near 6 km in the Southern part of the Tayrona basin. These two depocenters are separated by a basement high coincident with the Salamanca barrier island where the Salamanca-1 well reported quartz-diorites drilled at a depth of 2352 m BSL. The south west boundary of the Plato basin is formed by the basement high of Magangué or Cicuco, drilled by numerous wells. This high separates the Plato basin from the San Jorge basin. There, depths of 5500 m are interpreted in the hanging block of the Mojana normal fault. The San Jorge depocenter extends to the west and is separated by the Sinú region by the transpressive North Romeral fault, which is interpreted here as a deep seated flower structure at the Puerta Negra location.
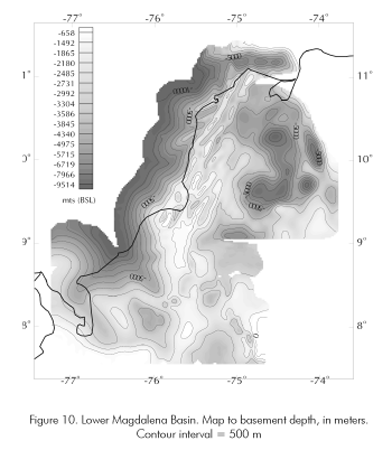
Finally, the Sinú basin reaches depths beyond 7 km near the coast line. The Porqueria-1 well reported more than 3300 m of Pliocene sediments alone. The Sinú basin is separated from the Urabá basin on the west by the strike slip/thrust Uramita fault. Depths in the Urabá basin reach almost 7 km South of the Necoclí-1 well. The basement rises gently towards the west, outcropping in Panamá.
FLEXURAL ANALYSIS
The geometry of the basement forms the basis for basin analysis studies. As an example, we apply here a known concept of flexural analysis to investigate the evolution of the Plato basin. The origin of the lower Magdalena basin has been defined as a forearc basin (Ladd et al., 1984), a foreland basin formed as the result of over thrusting by Caribbean-Arc rocks (Macellari, 1995), the result of faulting forming grabens and horsts (Caro, 2003) or a back-arc basin (Flinch, 2003). The geometry of the basement can be approximated from a flexural point of view to a flexed slab that bends as a response to tectonic forces applied to the same (Airy isostasy corresponds to an elastic thickness of cero, but higher elastic thicknesses are related with a more resistive slab).
Figure 11 shows a W-E oriented seismic line crossing the Plato basin. It has depth control by two wells (Balsamo-2 and San Ángel-3). We then attempt a simple flexural modeling for an extended profile of the basin assuming a vertical load applied at the center of a pre-existing, unbroken slab under the Plato basin. The result is shown in Figure 11b: the upper curve represents the flexural response for a Te of 25 km, the lower one is the response for a Te of 50 km. We conclude that an effective elastic thickness (Te) of about 27 km best matches the present shape of the basement, while larger values of Te would result in a basin that is too deep.
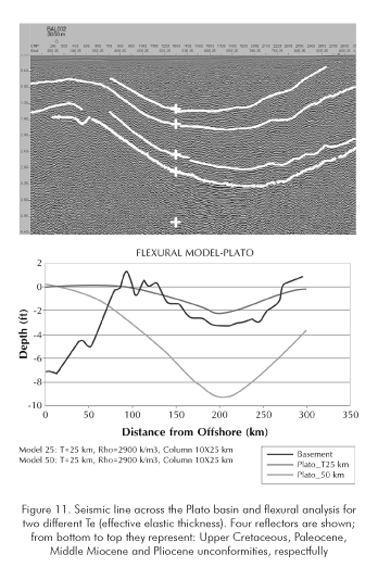
For the purposes of back stripping, we apply the sediment composition shown in Table 1. We follow the methodology described by Angevine, Heller, & Paola (1990). When we apply back stripping, the resulting sediment decompaction curves are shown in Figure 12. We notice that the shape of the curve resembles more the shape for a foreland basin, on early stages, rather than a forearc basin. (Insets taken from Angevine et al., 1990). Based on the seismic interpretation, the flexural analysis and the backstripping of the shown section, we propose the following evolutionary history for this sector:
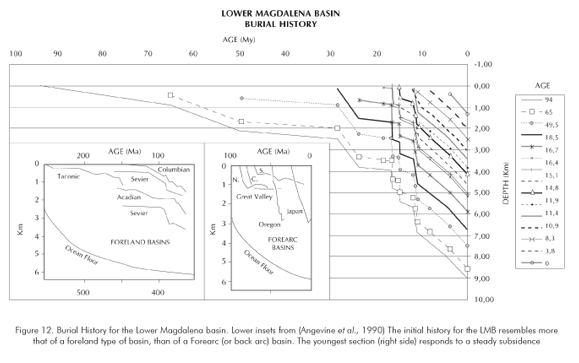
a) A platform until the end of the Cretaceous.
b) During the Paleogene and early Miocene the basin subsided uniformly, creating accommodation space by possible sea deepening.
c) Between the lower and the Middle Miocene a considerable tilting towards the East created a deeper basin in that direction. Before the Middle Miocene unconformity the San Jorge basin was subject to intense tectonism.
d) The tilting of the basin continues until the Lower Pliocene. This stage resembles that of a foreland basin. Tectonic loads could be the product of transpressive events at the San Jacinto fault.
e) From the Lower Pliocene (3,8 Ma) to present, the basin basically subsided at a very rapid rate. Plio-Pleistocene sediments are as thick as 12000 ft in the Sinú basin and around 15000 ft in the Magdalena fan.
CONCLUSIONS
• We built a complete data set with the available gravity data to produce maps of free air, complete Bouguer anomaly and combined anomaly maps for northern Colombia. 2 ½ D modeling provides the basis for basement geometry interpretation, basement composition and basement type discrimination, using well data, surface geology and seismic reflection data as boundary conditions.
• Basement drilled by numerous oil wells, outcrops and density models show that the crust underlying the Plato and San Jorge basins is continental in nature, and varies from granitic composition (Magangué high and El Difícil high) to low grade metamorphic rocks. The basement is a prominent reflector that can be followed in seismic lines and is controlled by oil wells.
• The reflections are not evident north of the San Jacinto fault system and West of the North Romeral fault, in the Sinú basin. There, gravity modeling alone provides the basis for postulating a very deep basin, filled with low density sediments and with evident presence of localized, yet considerable (2 km or more in diameter) bodies that require low density (1,8 to 2 g/cm3) to match the observed values. In same places they can be correlated with mud volcanoes in surface, like the case of the mud volcano near Mulatos in the Sinú area. This might imply that the relatively small vents that pour unconsolidated mud to surface in several places in the Sinú are in reality the necks of larger diapirs at depth, and these diapirs form an almost continuous belt that can be traced ocean ward in the NE direction, following the direction of the younger Sinú deformed belt. High resolution bathymetry shows the presence of mud volcanoes, cone-like shapes, donut-like and similar shapes at the sea bottom, several of which have been sampled by oil companies by means of sea bottom coring programs, evidencing unconsolidated mud extruding from them, very often impregnated with hydrocarbon traces.
• The interpretation of the model for the basement in the Sinú area does not require high densities typical of mafic rocks, but the observed anomaly can be explained with densities in the order of 2,6 – 2,7 g/cm3. This value is not conclusive of the absence of oceanic crust, but is better explained as bodies composed out of acidic rocks like the adjacent basin in San Jorge. Similar modeling made in the Pacific side, where well known outcrops of Cretaceous mafic and ultramafic rocks form the basement accreted to the continent, require values of the order of 2,8 to 2,9 g/cm3 to model the basement. We conclude that the rocks forming the basement in the Sinú basin are not of the same composition that those of oceanic affinity in the Western Cordillera, and can be assimilated more to a typical attenuated continental crust. For this reason we postulate a transitional continental basement for the Sinú basin.
• The 2 ½ gravity models don't require a high density mantle wedge at the base of the crust, as should be the case for a highly dipping subducting slab. The geometry of the Moho, as a continuous surface dipping gently from the ocean side under the continent, seems supported by tomographic profiles published by van der Hilst and Mann (van der Hilst, & Mann, 1994) and more recently by Vargas, Pujades, and Montes (2007), where the shape of the continental wedge / oceanic crust boundary resembles that of a very low angle/flat subduction zone (β angle between 2º to 3º), and is interpreted here as a low angle over thrusting of northern South America riding in a highly oblique direction over the underlying Caribbean plate.
ACKNOWLEDGEMENTS
This work was possible thanks to the sponsorship to the first author by Ecopetrol S.A., Colombia. Ecopetrol S.A. allowed the publication of proprietary data. Geophysical data was also contributed to the project by IGAC (William Martínez and Laura Sánchez), GETECH (Derek Fairhead) and Víctor Graterol (Venezuela data). The modeling software used in this paper was graciously licensed to the project by GM360 (Kwok Chan) and LCT-FUGRO. Dr. Fabio Cediel and two other anonymous reviewers greatly improved the manuscript. Finally, the stay of the first author at University of South Carolina was possible thanks to a main scholarship provided by Ecopetrol S.A., and fellowships granted by Chevron-Texaco, the SEG Foundation and the Geophysical Society of Houston. To all of them our deepest gratitude and prays.
REFERENCES
Agudelo, W. (2005). Imagerie sismique quantitative de la marge convergente d'Equateur-Colombie. Doctoral thesis, Paris 6, Paris, 203 pp. [ Links ]
Angevine, C. L., P. L. Heller, & C. Paola (1990). Quantitative sedimentary basin modeling. AAPG Continuing Education Course, Note Series, 32: 132. [ Links ]
Audemard, F.(2002). Transpressional tectonics of northern South America, Abstracts with Programs. Geological Society of America, 34: 76. [ Links ]
Baquero-Santa, E. (1983). Estructura y litología de un área al NW de la Península Guajira: un estudio de estratigrafía sísmica. Tesis de pregrado, Universidad Nacional de Colombia, Bogotá, 117 p. [ Links ]
Bassin, C., G. Laske, & G. Masters (2000). The current limits of resolution for surface wave tomography in North America, EOS Transactions AGU, 81, f897. [ Links ]
Bowland, C., L. & E. Rosencrantz (1988). Upper crustal structure of the western Colombia Basin, Caribbean Sea. Geological Society of America Bulletin, 100: 534-546. [ Links ]
Brocher, T. (2005). Empirical relations between elastic wavespeeds and density in the earth's crust. Bulletin of the Seismological Society of America, 95: 2081 - 2092. [ Links ]
Caro, M. (2003). Structural evolution of the San Jacinto fold belt, NW Colombia. MsC Thesis, University of Calgary, Calgary, 72 p. [ Links ]
Case, J. E. (1975). Geophysical studies in the Caribbean Sea. In A.E.M. Nairn, and F. G. Stehli, (eds.). New York, Plenum Press. [ Links ]
Case, J. E., J. Braunstein, & A. F. Thomson (1982). Crustal framework of Caribbean geologic terranes. Abstracts with Programs, Geological Society of America. 14: 461. [ Links ]
Case, J. E., W. MacDonald, & P. J. Fox (1990). Caribbean crustal provinces. Seismic and gravity evidence. In G.Dengo, & J.E. Case (Ed), The geology of North America (Vol. H). The Caribbean region. Boulder, CO. Geological Society of America. [ Links ]
Case, J. E., W. R. Moore, L. G. Durán, & A. López R. (1971). Junction of the Andean and Panamanian chains in northern Colombia. Transactions of the Caribbean. Geological Conference, Memorias - Conferencia Geológica del Caribe, 5: 11-13. [ Links ]
Cediel, F. & C. Cáceres (2000). Geological map of Colombia, Scale 1:1,000,000, (3rd. ed.). Geotec Ltd Ed., Bogotá, Colombia. [ Links ]
Cediel, F., C. Cáceres, & P. Shaw (2003). Tectonic assembly of the northern Andean block. The circum-Gulf of Mexico and the Caribbean: Hydrocarbon habitats, basin formation and plate tectonics, Memoir 79, AAPG, 815 - 848. [ Links ]
Chapin, D. (1996). A deterministic approach toward isostatic gravity residuals - A case study from South America, Geophysics, 61: 1022 - 1033. [ Links ]
Diaz L., & B. Ramos (2004). Mapa Geológico de Colombia a Escala 1: 1 000,000, ARIANA ltda. (Ed.). Bogotá, Colombia. [ Links ]
Diebold, J. B., P. L. Stoffa, P. Buhl, & M. Truchan (1981). Venezuela Basin crustal structure. Journal of Geophysical Research, 86: 7901-7923. [ Links ]
Donnelly, T. W., D. Beets, M. J. Carr, T. Jackson, G. Klaver, J. F. Lewis, R. Maury, H. Schellenkens, A. L. Smith, G. Wadge, & D. Westercamp (1990). History and tectonic setting of Caribbean magmatism. In G. Dengo, and J. E. Case (Eds.),. The Caribbean region. Boulder, CO. Geological Society of. America, 339-374. [ Links ]
Driscoll, N. W., & J.B. Diebold (1998). Deformation of the Caribbean region, one plate or two. Geology, 26: 1043-1046. [ Links ]
Duque-Caro, H. (1979). Major structural elements and evolution of northwestern Colombia. AAPG Memoir 29: 329-351. [ Links ]
Duque-Caro, H. (1984). Structural style, diapirism, and accretionary episodes of the Sinú-San Jacinto terrane, southwestern Caribbean borderland. Geological Society of America, 162: 303-316 [ Links ]
Duque-Caro, H. (1990a). Mayor Neogene events in Panamic South America. In R. Tsuchi (Ed.). Tokio University: Tokio Press. [ Links ]
Duque-Caro, H. (1990b). Neogene stratigraphy, paleoceanography and paleobiogeography in northwest South America and the evolution of the Panamá seaway. Palaeogeography, Palaeoclimatology, Palaeoecology, 77: 203-234. [ Links ]
Duque-Caro, H. (1997a). The Llanos Basin, Colombia. In search of greater stratigraphic resolution. J. Petroleum Geol., 20: 96-99. [ Links ]
Duque-Caro, H. (1997b). The marine neogene of Colombia; major biostratigraphic, paleoceanographic and tectonic constraints. Second Congress of the Regional Committee on Atlantic Neogene Stratigraphy (RCANS), Salamanca, Spain, October. [ Links ]
Edgar, N. T., J. I. Ewing, & J. Hennion (1971). Seismic refraction and reflection in Caribbean sea. American Association of Petroleum Geologists Bulletin, 55: 833-870. [ Links ]
Escalona, A. (2006). Multiphase tectonic evolution of Northwestern Venezuela and its offshore area. Implications for the Distribution of Source and Reservoir Rocks. AAPG Annual Convention, April 9-12, Technical Program, Houston, TX. [ Links ]
ESRI-ILEX. (1995). Evaluacion geológica regional de la cuenca del Sinú-San Jacinto. In R. Allen, Clark, W., Laverde, F., Schammel, S., Ballesteros, I. (Ed.). Bogotá, 248. [ Links ]
Feng, M., S. van der Lee, & M. Assumpcao (2007). Upper mantle structure of South America from joint inversion of waveforms and fundamental mode group velocities of Rayleigh waves. J. of Geophysical Research, 112:16. [ Links ]
Fliedner, M. M., & S. L. Klemperer (2000). Crustal structure transition from oceanic arc to continental arc, eastern Aleutian Islands and Alaska Peninsula. Earth and Planetary Science Letters, 179:567-579. [ Links ]
Flinch, J. F. (2003). Structural evolution of the Sinú-Lower Magdalena area (northern Colombia). AAPG Memoir, 79: 776-796. [ Links ]
Flueh, E. R., B. Milkereit, R. Meissner, R. P. Meyer, J. E. Ramirez, J. C. Quintero, & A. Udias (1981). Seismic refraction observations in northwestern Colombia at latitude 5,5 degrees N. In H. Miller, and U. Rosenfeld (eds.), Zentralblatt fuer Geologie und Palaeontologie, Teil I. Allgemeine, Angewandte, Regionale and Historische Geologie, 3-4: 231-242. [ Links ]
GETECH. (2001). Colombia satellite gravity and Bathymetry - Atlantic region, Leeds, UK, ECOPETROL, Internal Report, 34. [ Links ]
González, H. (2001). Mapa geológico del departamento de Antioquia. Bogotá, Ingeominas, 241. [ Links ]
Green, C.M., Fairhead, J.D. (1993). The South American Gravity Project. In Recent Geodetic and Gravimetric Research in Latin America (Ed.). W. Torge, A. González-Fletcher, & J. Tanner. Spring-Verlag, Berlin. [ Links ]
Guedez, M., C. Zelt, B. Magnani, & A. Levander (2006). Crustal structure across the caribbean south america plate boundary at 70°w, results of the bolivar project. Speciality Meeting Nº 1. Geologycal Society of America, Backbone of the America. Medoza, Argentina, 60. [ Links ]
Guzmán, G. (2003). Mapa geológico de Sinú-San Jacinto y Borde Oeste Valle Inferior del magdalena. In Ingeominas (Ed.), mapas geológicos. Bogotá, Ingeominas. [ Links ]
Henao, D. (1961). Gravity compilation of COLPET´s concessions in Northern Colombia. Report Nº 283, Colombian Petroleum Company. New York, USA. [ Links ]
Hernández, R., & C. Guerrero (2006). Expresión profunda de dominios oceánico y continental, y propagación de su deformación hacia la cobertera sedimentaria del "Offshore" Caribe. X simposio Bolivariano, Exploración Petrolera en las Cuencas Subandinas, 4, Cartagena, Colombia. [ Links ]
Hopkins, H.R., N.T. Edgar, J.B. Saunders, H.M. Bolli, R.E. Boyce, W.S. Broecker, T.W. Donnelly, J.M. Gieskes, W.W. hay, R.M. horowitz, F. Maurrasse, H. Perez-Nieto, W. Prell, I. Premoli, W.R. Riedel, N. Schneidermann & L.S. Waterman (1973). Geology of the Aruba Gap abyssal plain near DSDP Site 153. Initial reports of the Deep Sea Drilling Project, 45: 1039-1050. [ Links ]
Houtz, R. E., &, W. J. Ludwig (1977). Structure of Colombia Basin, Caribbean Sea, from profiler-sonobuoy measurements. J. of Geophysical Research, 82: 4861-4867. [ Links ]
Irving, E. M. (1975). The structural evolution of the northernmost Andes of Colombia. Boletín Geológico, Ingeominas, 19: 89. [ Links ]
Jacobsen, B. H., 1987. A case for upward continuation as a standard separation filter for potential-field maps. Geophysics, 52: 1138-1148. [ Links ]
Karner, G. D., & A. B. Watts (1983). Gravity anomalies and flexure of the lithosphere at mountain ranges. J.of Geophysical Research, 88: 10449 - 10477. [ Links ]
Kellogg, J.N., Godley, V.M., Ropain, C., Bermúdez, A., and Aiken, C.L.V., 1991, Gravity Field of Colombia, Eastern Panama and Adjacent Marine Areas, The Geological Society of America, Map and Chart Series MCH 070, Boulder, Colorado. [ Links ]
Kellogg, J. N., V. Vega, T. C. Stallings, & C. L. V. Aiken (1995). Tectonic development of Panamá, Costa Rica, and the Colombian Andes; constraints from Global Positioning System geodetic studies and gravity. Special Paper, Geological Society of America, 295: 75-90. [ Links ]
Kerr, A. C., & J. Tarney (2005). Tectonic evolution of the Caribbean and northwestern South America, the case for accretion of two Late Cretaceous oceanic plateaus. Geology, 33: 269-272. [ Links ]
Kerr, A. C., J. Tarney, P. D. Kempton, M. Pringle, & A. Nivia (2004). Mafic pegmatites intruding oceanic plateau gabbros and ultramafic cumulates from Bolivar, Colombia. Evidence for a "wet" mantle plume? J. of Petrology, 45:1877-1906. [ Links ]
Kerr, A. C., J. Tarney, A. Nivia, G. F. Marriner, & A. D. Saunders (1998). The internal structure of oceanic plateaus; inferences from obducted Cretaceous terranes in western Colombia and the Caribbean. Tectonophysics, 292: 173-188. [ Links ]
Kerr, A. C., R. V. White, P. M. E. Thompson, J. Tarney, & A. S. Saunders (2003). No oceanic plateau; no Caribbean Plate? The seminal role of an oceanic plateau in Caribbean Plate evolution. AAPG Memoir, 79: 126-168. [ Links ]
Ladd, J., M. Truchan, M. Talwani, P. Stoffa, P. Buhl, R. Houtz, A. Mauffret, & G. Westbrrok (1984). Seismic reflection profiles across the soothern margin of the Caribbean. In W. Bonini, and e. al, /(eds.), The Caribbean- South American plate boundary and regional tectonics. Memoir 162, Geological Society of America. [ Links ]
Larue, D. K. (2000). Active Strike-Slip and Collisional Tectonics of the Northern Caribbean Plate Boundary Zone, Edited by J.F. Dolan and P. Mann. (1999). Geological Society of America, Special Paper 326: 174. Marine and Petroleum Geology,17:656. [ Links ]
Laverde, F. (2000). The Caribbean basin of Colombia, a composite cenozoic accretionary wedge with under-explored hydrocarbon potential. VII Simposio Bolivariano exploración en las cuencas subandinas, 23, Caracas, Venezuela. [ Links ]
Levander, A., M. Schmitz, H. G. Ave Lallemant, C. A. Zelt, D. S. Sawyer, M. B. Magnani, P. Mann, G. L. Christeson, J. E. Wright, G. L. Pavlis, & J. Pindell (2006). Evolution of the southern Caribbean Plate boundary, Eos, Transactions. American Geophysical Union, 87: 97. [ Links ]
Lewis, J.F., Draper, G., Proenza, J.A., Espaillat, J., & Jimenez, J. (2006). Ophiolite-related ultramafic rocks (serpentinites) in the Caribbean Region. A review of their occurrence, composition, origin, emplacement and ni-laterite soil formation. Geologica Acta, l 4: 7-28. [ Links ]
Ludwig, W., R.E. Houtz, & J.I. Ewing (1975). Profiler-Sonobuoy measurements in Colombia and Venezuela Basins, Caribbean Sea. AAPG Bulletin, 59: 115-123. [ Links ]
Macellari, C.E. (1995). Cenozoic sedimentation and tectonics of the southwestern Caribbean pull-apart basin, Venezuela and Colombia, Petroleum basins of South America. AAPG Memoir 62: 757-780. [ Links ]
Mantilla-Pimiento, A., C.A. Alfonso-Pava, G. Jentzsch, & J. Kley (2005). Crustal structure of the southwestern Colombian Caribbean area. 6th International Symposium on Andean Geodynamics -ISAG 2005- , 472-476. [ Links ]
Marcaillou, B., 2005, Regimes tectoniques et thermiques se la marge Nord Equateur - Sud Colombie (0º - 3, 5º N) - Implications sur la sismogenese, Paris VI, Paris, 197. [ Links ]
Martínez, W., L. Sánchez, & J. Flórez (1995). Determinación de nuevas estaciones de gravedad absoluta en Colombia. Geofísica Colombiana, 3: 21-87. [ Links ]
Mauffret, A., & S. Leroy (1997). Seismic stratigraphy and structure of the Caribbean igneous province. Tectonophysics, 283: 61. [ Links ]
Mauffret, A., S. Leroy, E. d'Acremont, A. Maillard, B. M. de Lepinay, A. T. Dos Reis, N. Miller, A. Nercessian, R. Pérez-Vega, & D. Pérez (2001). Une coupe de la province volcanique Caraibe, premiers resultats de la campagne sismique Casis 2. A transect of the Caribbean volcanic province, first results of the seismic cruise CASIS 2. Comptes Rendus de l'Academie des Sciences - Series IIA -. Earth and Planetary Science, 333: 659. [ Links ]
Maya, M. (1992). Catálogo de dataciones isotópicas en Colombia. Boletín Geológico - Ingeominas, 32: 127 - 188. [ Links ]
Meissner, R.O., E.R. Flueh, F. Stibane, & E. Berg (1976). Dynamics of the active plate boundary in Southwest Colombia according to recent geophysical measurements. Tectonophysics, 35: 115-136. [ Links ]
Mooney, W. D., G. Laske, & T. G. Masters (1998). CRUST 5.1, A global crustal model at 5º X 5 º. Journal of Geophysical Research, 103: 727-748. [ Links ]
Nafe, J.E., Drake, C.L. (1963). Physical properties of marine sediments. The Sea., 3, New York. Interscience, 784–815. [ Links ]
Nivia, A. (1996). The Bolivar mafic-ultramafic complex, SW Colombia. The base of an obducted oceanic plateau. J. of South American Earth Sciences, 9: 59. [ Links ]
Nivia, A., & J. Gómez (2005). Consideraciones acerca del modelo geológico evolutivo del occidente colombiano (Colombia). X Congreso Colombiano de Geología, Bogotá, Colombia. [ Links ]
Pindell, J.L. (1994). Paleogeographic evolution and hydrocarbon systems of northern South America. Annual Meeting Abstracts - American Association of Petroleum Geologists and Society of Economic Paleontologists and Mineralogists, 235. [ Links ]
Reyes, A., M. (2000). Evolución tectono-estratigráfica del valle inferior del Magdalena, Colombia. VII simposio Bolivariano, exploración petrolera en las cuencas subandinas, Caracas, Venezuela. [ Links ]
Reyes, J.P., & M.C. Rueda (Eds.). (2001). Evaluación de la prospectividad del sector Atlántico. Informe Técnico, Ecopetrol S.A. - Instituto Colombiano del Petróleo (ICP), 177pp. [ Links ]
Reyes, J. P., M.C. Rueda, M. Mantilla, & L. E. Ardila (2002). Evolution and exploration implications of the Colombian Caribbean accretionary prism. Annual Meeting Expanded Abstracts - American Association of Petroleum Geologists, 147-148. [ Links ]
Rogers, R.D. (2003). Jurassic-Recent tectonic and stratigraphic history of the chortis block of Honduras and Nicaragua (northern Central America). Doctoral thesis, University of Texas at Austin, Austin, USA. [ Links ]
Russo, R. M., & R. C. Speed (1994). Spectral analysis of gravity anomalies and the architecture of tectonic wedging, NE Venezuela and Trinidad. Tectonics, 13: 613-622. [ Links ]
Sánchez, L. (2003). Bestimmung der Hogenreferenzflache fur Kolumbien. Doctoral Thesis, Technishe Universitat Dresden, Dresden, 102 pp. [ Links ]
Schamel, S., R.B. Allen, D. Schelling, D. Wavrek, F. Laverde M, & C. I. Ballesteros T (1998). Hydrocarbon potential of the Sinú-San Jacinto region of northern Colombia. AAPG Annual Meeting Expanded Abstracts, Salt Lake City, UT, USA. [ Links ]
Schenk, C.J., Viger, R.J., and Anderson, C.P., 1999, Maps showing geology, oil and gas fields, and geologic provinces of South America: U.S. Geological Survey Open-File Report 97-470D, one CD-ROM. [ Links ]
Schmitz, M., A, C.I. Martins, M.I. Jácome, J. Sánchez, and V. Rocabado (2005). The major features of the crustal structure in north-eastern Venezuela from deep wide-angle seismic observations and gravity modelling. Tectonophysics, 399:109– 124. [ Links ]
Spector, A., & S. Grant (1970). Statistical models for interpreting aeromagnetic data. Geophysics, 35: 293-302. [ Links ]
Tanner, J.G., C.L. Aiken, P. Dehlinger, W. Dewhusrt, M. de la Fuente, V. Godley, R. Godson, W.F. Hanna, T.G. Hildenbrand, M.D. Kleinkopf, G. McCalpin, R.K. Mc Connell, H. Meyers, N.W. O`Hara, A.R. Palmer, D. Scheibe, R.E. Sweener, & L. Thorning (1988). Gravity anomaly map of North America. The Leading Edge, 7 (11), 15-18. [ Links ]
Van der Hilst, R., & P. Mann (1994). Tectonic implications of tomographic images of subducted lithosphere beneath northwestern South America. Geology, 22: 451 - 454. [ Links ]
Vargas, C.A., L.G. Pujades, & L. Montes (2007). Seismic structure of South-Central Andes of Colombia by tomographic inversion. Geofísica Internacional, 46:117-127. [ Links ]
Weber, J.C., T.H. Dixon, C. DeMets, W.B. Ambeh, G. Mattioli, J. Saleh, G. Sella, R. Bilham, & O. Pérez (2001). GPS estimate of relative motion between the Caribbean and South American plates, and geologic implications for Trinidad and Venezuela. Geology, 29: 75 - 78. [ Links ]
Yuan, X., S.V. Sobolev, & R. Kind (2002). Moho topography in the central Andes and its geodynamic implications. Earth and Planetary Science Letters, 199: 389-402. [ Links ]
(Received Sept. 25, 2007; Accepted Oct. 29, 2007)