Services on Demand
Journal
Article
Indicators
-
Cited by SciELO
-
Access statistics
Related links
-
Cited by Google
-
Similars in SciELO
-
Similars in Google
Share
CT&F - Ciencia, Tecnología y Futuro
Print version ISSN 0122-5383On-line version ISSN 2382-4581
C.T.F Cienc. Tecnol. Futuro vol.4 no.2 Bucaramanga July/Dec. 2010
DETERMINATION OF MOLECULAR WEIGHT OF VACUUM RESIDUE AND THEIR SARA FRACTIONS
Adan-Yovani León1* and Martha-Josefina Parra1*
1Ecopetrol S. A. - Instituto Colombiano del Petróleo (ICP), A.A. 4185 Bucaramanga, Santander, Colombia
e-mail: adan.leon@ecopetrol.com.co marthajparra@gmail.com
(Received, June 29, 2010; Accepted, Dec. 9, 2010)
*To whom correspondence may be addressed
ABSTRACT
The molecular weight of a number of vacuum residue and their SARA fractions (Saturated, Aromatics, Resins, and Asphaltenes) typical of the Barrancabermeja refinery in Colombia were determined using the following methods: Gel Permeation Chromatography (GPC), Vapor Pressure Osmometry, (VPO), and Simulated Distillation at High Temperature adjusted to the Gamma distribution function. Molecular weight results in vacuum residue and saturated and aromatics fraction obtained from the three techniques used show consistency within an acceptable range. However, the molecular weight results in resins and asphaltenes fraction obtained by the VPO technique significant differences compared with the results found using the techniques of GPC and DS-Gamma.
Keywords: vacuum residues, molecular weight, gel permeation chromatography, petroleum fraction.
RESUMEN
Se determinaron los pesos moleculares para un número de fondos de vacío típicos de la refinería de Barrancabermeja de Colombia y sus fracciones SARA (saturados, aromáticos, resinas y asfaltenos), usando los métodos de cromatografía de permeación en gel (GPC), osmometría de presión de vapor (VPO) y destilación simulada de alta temperatura ajustada con la función de distribución Gamma. Los resultados de peso molecular en fondos de vacío y sus fracciones de saturados y aromáticos obtenidos a partir de las tres técnicas empleadas muestran consistencia dentro de un rango admisible. Sin embargo, los resultados del peso molecular de las fracciones de resinas y asfaltenos obtenidos por la técnica de VPO, presentan diferencias significativas en comparación con los resultados encontrados usando las técnicas de GPC y DS-Gamma.
Palabras clave: fondos de vacío, peso molecular, cromatografía de permeación en gel, fracciones de petróleo.
1. INTRODUCTION
In the study of well-defined chemical system, their physical-chemical properties can be measured in a reproducible mode with high reliability. However, in the matter of oil fractions such as SARA fractions (Saturated, Aromatics, Resins, and Asphaltenes) originating from heavy crude oils and even from vacuum residue, it is considered that their properties are the result of a mixture of compound families with different chemical structures that acquire an average and characteristic behavior, (Wiehe & Liang, 1996; León, Parra, & Grosso, 2008).
The vacuum residue composition and its fractures depend of the nature of the oil and the processing conditions in the refineries. The fractions of heavy crude oil and its vacuum residue are constituted by a large variety of chemical structures with various molecular weights (Wu & Preusnitz, 1998).
With the growing demand for heavy crudes and vacuum residue, various studies on the composition and characterization of its fractions have been performed, due to the need to interpret its behavior before the various operation processes. Therefore, several studies have been aimed at the characterization of heavy crudes unconventional and their SARA fractions; especially to determining their molecular weights.
In the measurement of molecular weights, it is common to use the methods of Gel Permeation Chromatography (GPC), and Vapor Pressure Osmometry (VPO). However, Sheu, De Tar, & Storm (1991), Yarranton,Alboudwarej, & Jakher (2000) consider that the molecular weight results obtained with various methods for heavy crude fractions, as in resins and Asphaltenes, show significant differences mainly attributed to their complex nature, to the tendency of association with organic solvents, changes in temperature, and to concentration. On the other hand, Masuda, Okuma, Kanaji, & Matsumara (1996) and Guzmán, Bueno, & Carbognani (2009), consider the results also depend on the selection of the standards and calibration standards that may affect in the structural difference in respect to the fractions to be analyzed.
The GPC technique is used more often in determining the distribution of molecular weights. The GPC method uses a column that contains a filling of a microporous gel, which allows a gradual elution of species of greater to lesser molecular size in a mixture.
The distribution of components in a mixture can be represented in various ways, amongst these forms is the average molecular weight in number and weight represented as Mn and Mw, and their ratio is identified with the polydispersity index, (D = Mn/Mw).In definition, the Mn is the result of adding the molecular weight of each chemical species divided by the total number of species present. Therefore, the Mw is the average of molecular weights of the species present, based on their mass fraction.
The polydispersity index to determine the degree of dispersion of a mixture of chemical species or molecules. When D is equal to 1, all chemical species in the mixture have the same molecular weight and in that case there is no dispersion. Therefore, the greater the value of D, the greater the dispersion in the distribution of molecular weights, which means that there is a great amount of chemical species of different molecular weight and shape, (Gacén, Maíllo, Knott, & Altenhofen, 1985).
Cervo and Thies (2010) in their work were able to show that supercritical extraction of heavy crude fractions consist of a large proportion of aromatic hydrocarbons polycondensates grouped with average molecular weights between 200 and 2000g/g-gmol. Moreover, they indicate that the lighter fractions are composed of monomers and dimers, whereas the heavier fractions, show increases of polymerization represented by trimers and tetramers.
Kharrat (2009) applied a sequential extraction technique asphalting subfractions with tetrahydrofuranhexane in different proportions. With the change in solvent polarity, showed that asphaltenes are grouped into sub-fractions of different polarity, structure and molecular weight. NMR was possible to establish that the subfractions of asphaltenes consist of a hypothetical molecule aromatic Polycondensates minimum variation and cyclic alkyl substitutions. However, although there are mixtures with very similar average molecular weights, it is possible to identify a wide difference of molecular weight distribution.
VPO is another of the techniques used to determine average molecular weights. This technique is based on the different vapor pressure produced by the addition of a small quantity of solute to a pure solvent, considering as criterion that the vapor pressure of a pure solvent is greater in comparison to any solution containing a solute. A drop of pure solvent and a drop of solute -solvent (solution), are placed in separate thermistors surrounded by the vapor of the pure solvent. The difference in pressure between the two drops generate different temperature readings in each of the thermistors, which generate a difference in voltage proportional to the number of particles or number of moles dissolved in the solution (Peramanu, Pruden, & Rahimi, 1999; Yarranton et al., 2000 and Buenrostro, Andersen, García, & Lira, 2002).
The molecular weight is a property of great interest in the oil industry for the development of correlations, in order to predict other physical-chemical properties for improvement of refining performance. For example, to model the extraction model of multicomponents in bitumen, heavy crude, and vacuum residue, there are many mathematical methods of thermo-dynamic character, based on equations state and theory regular solution. For the development of these models the critical properties of the components are evaluated in respect to their molecular weights (Akbarzadeh, Alboudwarej, Ayatollahi, & Yarranton, 2004); Golam & Mansouri, 2006; Sabbagh, Akbarzadeh, Badamchi-Zadeh, Svrcek, & Yarranton, 2006 and Parra & Cañas, 2007).
In the same oil line, Borges, Belsay, & Acevedo (2007) isolated and characterized fractions from extra heavy crude. The molecular weight of the asphaltenes, resins, and carboxylic acids were determined based on the VPO and GPC techniques.
Once the intervals of molecular weight were found for the acid fraction, it was determined that this fraction has characteristics between asphaltenes and resins. On another side, Navarro, Álvarez, Groso, & Navarro (2004) characterized the heavy fractions of Castilla Crude oil to place in evidence the interaction between resins and asphaltenes. Within this characterization the molecular weight of these fractions were determined through the GPC and VPO techniques. Due to the difference in the results, a molecular weight range of between 2000 and 2200 g/g-mol and between 500 and 1300 g/g-mol was proposed for asphaltenes and resins, respectively.
In order to interpret the chemical distribution of heavy crudes and vacuum residue with greater certainty, various authors as Keng, Chunming, Yunxiang, & Renan (1997) and Zhao, Zhiming, Chunming, Keng, & Renan (2005) have been developing methods for the extraction of fractions with fluids in supercritical conditions. With the elemental analysis, the molecular weight determined by GPC and VPO, and the structural parameters obtained by NMR analysis, they have been able to predict the possible representative molecules of the fractions obtained and a great number of correlations of physical-chemical properties (Tie-Pan, Yun-Xiang, Tong, & Renan, 1997; Guangua & Wang, 1999).
Amongst the characterization methods for crudes there is the one proposed by Behrenbruch and Dedigama (2007); they presented a method for the prediction of the properties of variable nature crudes, based on the distillation curve and the parameters of the Gamma distribution function.
With similar works, León et al. (2008), present an alternative method to estimate the molecular weight of vacuum residue and their SAR fractions (saturated, aromatics, and resins), based on the technique of simulated distillation at high temperature and Gamma distribution function. With the technique of simulated distillation at high temperature, the recovery percentage is not complete for heavy fractions such as vacuum residue and its SAR fractions. However, for the development of this method, components with greater molecular weight to the component with Carbon number C100 from the calibration curve were assumed. In addition, the quantities of the components greater than C100 were estimated until completing 100% of the distribution, taking into account the molecular weights found for the model molecules. For every vacuum residue and its SAR fractions, as of the C100 component, increases in ΔPM molecular weight were adjusted to a maximum reading of PMmax corresponding to 100% of the recovery.
With the fractions in weight estimated with the components with molecular weights greater than C100, and with the simultaneous adjustment of the α and β parameters of the Gamma function with Microsoft ExcelTM solver, the experimental curve and the gamma distribution curve are constructed based on the results of molar fraction density and the probability data found with the function of probability density for each component. The average molecular weight estimated with the function of gamma probability density is estimated with the expression PM = αβ. Finally, the molecular weight of the asphaltenes is determined by balance of matter, considering the principle of the rule of mixtures, as of the compositional percentage of the SARA analysis and the molecular weights found for the vacuum residue and its SAR fractions.
With previous works, the labor herein is aimed at the comparison of the results of molecular weight obtained for a set of typical vacuum residue of the Barrancabermeja refinery and their SARA fractions, through the methods of Gel Permeation Chromatography (GPC), Vapor Pressure Osmometry (VPO) and the method proposed by simulated distillation at high temperature adjusted to the Gamma distribution function.
2. EXPERIMENTAL METHOD
The selected vacuum residue originates from the vacuum distillation units of the Barrancabermeja Refinery, to cover a wide interval of compositions. The characterization of the vacuum residue was carried out following the ASTM methods standardized by the Instituto Colombiano del Petróleo (ICP), such as: density D-4052/70, sulfur D1552, basic nitrogen in hydrocarbons by UOP 269 Potentiometric Tritation and SARA D-2007compositional analysis.
To meet the purpose of this research, the SARA separation analysis was initially performed for each of the vacuum residue.Afterwards, the molecular weights of the vacuum residue and their SARA fractions were evaluated, based on the methods of Gel Permeation Chromatography (GPC), Vapor Pressure Osmometry, (VPO) and simulated distillation at high temperature with Gamma distribution.
Analysis of SARA separation
The liquid chromatography is preceded by a precipitation of asphaltenes; a ratio of 1:40 in volume of vacuum residue and n-heptane was used in the separation, as per theASTM D-4124 standard. The separation of maltenes was validated with the ASTM D-2007 standard; the elution of saturated hydrocarbons was carried out with n-heptane; the aromatic hydrocarbons were treated by reflux extraction, with a 1:1 mixture in volume of toluene-acetone in a silica column; later, the resin fraction was obtained by reflux extraction, with a mixture of 1:1 of toluene-acetone in a column of clay. This study has used a separation method of liquid chromatography at a preparative scale in order to obtain significant quantities of each of the SARA fractions.
Gel Permeation Chromatography (GPC) molecular weight estimation
Tetrahydrofuran THF stabilized with butylated Hydroxytoluene was used as mobile phase. The polystyrene/polydivinylbenzene column used (PLgel MIXED-E) allows the measurement of molecular weights in the range of 50 to 100,000 g/g-mol. The samples are injected in the solution with THF with an average concentration to 8000 ppm. The team used a WATERS 600 controller with controlled temperature to 35°C. The elution components record is performed with an arrangement of WATERS 996 diodes, fixed at a wavelength of 254 nm.
The method used a pattern sample constituted by a mixture of aromatic species and phthalocyanines, followed by a set of polyestyrenes to cover a wide range of molecular weights. The concentration of the standards was varied as per the sensitivity of the reply in the detector, Guzmán et al. (2009).
Vapor Pressure Osmometry (VPO) molecular weight estimation. The estimation of molecular weights by VPO, was carried out in the K-7000 KNAUER vapor pressure osmometer. The molecular weights were obtained in solution with dichlorobenzene in the concentration interval of 8 to 34 g/Kg, under 125°C work temperatures. As sample pattern the following were used 1, 4, 8, 11, 15, 18, 22, 25 - Octabutoxy-29H, 31H-phthalocyanine. The average constant of the team was of 988,694.
Simulated Distillation and Gamma Distribution Function molecular weight estimation
Beginning with the analysis of simulated distillation at high temperature, we obtain the distribution profile of samples such as heavy crude, atmospheric and vacuum residue. The ratio between the retention times and the correspondingebullition temperatures are establishedthrough a calibration curve, from a normal mixture of paraffin of n-C5 up to n-C100, prepared in carbon disulfide CS2, in a chromatograph of gases HP 6890, under adequate specifications of the ASTM D-6352-04 standard.
In the results of this analysis we obtain the percentage ratio of samples in distilled weight with temperature. However, as of the distillation curve and the percentage of composition of the standard sample, we determined the average molecular weight of the vacuum residue under study and their SAR fractions (Saturated, Aromatics, and Resins).
Considering the molecular weight of the vacuum residue PMFV-, as a property rule of mixtures calculated from molecular weight and the mole fractions of each SARA fraction, we have the following expression:
The measurement of molecular weight asphaltene fraction is found by the clearance of the above equation, Leon et al. (2008).
3. RESULTS AND DISCUSSION
Table 1 shows the result of some physical-chemical properties and the SARA of the typical vacuum residue selected from the Barrancabermeja refinery.
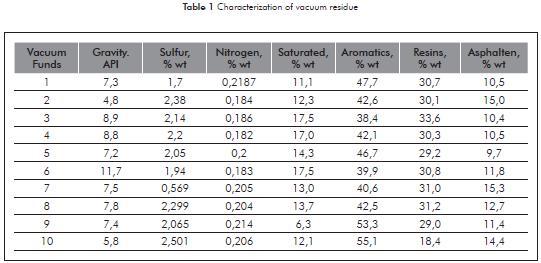
Figure 1 illustrates the results of the molecular weights of the vacuum residue and their SARA fractions, based on the three methods used.
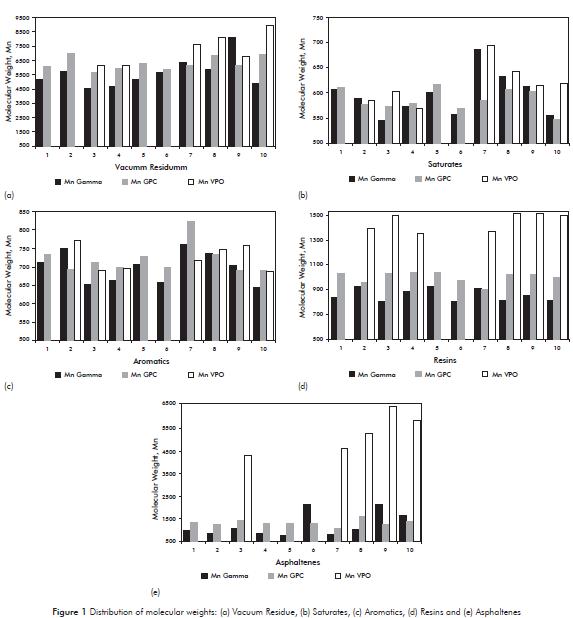
The vacuum residues are characterized by high content of heteroatoms such as nitrogen, sulfur, and oxygen, distributed in a great variety of functional groups (Table 1). The content of heteroatoms in the vacuum residue is distributed in great part in resins, and in lesser proportion, in the asphaltenes. The nature and increase of content of hetero-compounds in the resin and asphaltene fractions allow the increase of polarity and the degree of association of the species due to the formation of various types of intermolecular interaction (Zhu, Fu, Sheng, Xiang, & Bowen, 1998; Mullins, Sheu, Hammami & Marshall (2007); Castellanos, Borrero, & Garrido, 2009).
The results of molecular weights illustrated in Figure 1, is evident that the compound of saturate and aromatic fractions have lesser polarity and therefore are found in a lesser degree of association in comparison to the resin and asphaltene fractions.
The molecular weight of vacuum residue and the saturate and aromatic fractions obtained through the GPC and VPO techniques, present tendencies similar to variations in range of 7 to 103,6 to 107, and 2 to 110 g/g-mol, respectively. While the resin and asphaltene fractions present significant differences with variations in the range of 319 to 506, and 2853 to 5235 g/g-mol, respectively.
This work as in the studies carried out by various researchers such as Guzmán et al. (2009) indicate that there are two important factors in the difference in molecular weight obtained for the resin and asphaltene fractions through VPO and GPC. In the first place, it is due to the effects of the association related to the high concentration in solution which allow the formation of multiple aggregates. In the second place, to the method exposed for each technique to process the sample solution; where the VPO technique is based on the effect that the degree of association of solute produces in the vapor pressure of the solvent, while the GPC technique controls the molecular distribution of the compounds through a current that acts as mobile phase.
The results of this work present a tendency similar to that reported in other research. For example, Hunt and Winans (1997), found a wide difference in the molecular weights reported for a same group of asphaltenes and resins in the range of 814 to 1274 g/g-mol, and 2360 to 3248 g/g-mol, through the techniques of chromatography with mass spectrometry and VPO respectively.
The reported results show that the estimation of average molecular weight in the resin and asphaltene fractions through the VPO technique is not an easy task, due to the heterogeneity of the species that compose these fractions, and the high tendency to from aggregates.
In addition to the association effects, this research considers that the nature of the pattern sample to calibrate the equipment of each of the techniques used plays an important role in the tendency of the results.
In similar works carried out by Borges et al. (2007), they used patterns of octylated asphaltenes in THF and pyrene to evaluate the GPC and VPO techniques respectively; for which they found that in the results of molecular weight there were evident differences of approx. 100 g/g-mol for a same group of isolated resins and asphaltenes of extra heavy crude. In accordance to previous works, it is indicated that the difference in values of molecular weight by GPC and VPO, can be reduced with the selection of patterns with natures similar to the samples that are to be analyzed.
The D polydispersity index reported in Table 2, have different values for the vacuum residue and its SARA fractions, except to note that the polydispersity has similar values between samples of the same nature. However, the polydispersity values are the lowest for the proportion of saturated and aromatic, this indicates that these fractions are composed of less complex structures compared with the resin and asphaltene fractions. The results of the polydispersity index allow evidencing that although the SAR fractions originate from different vacuum residue with different concentration, they present a similar distribution tendency of species, possibly with similar structures.
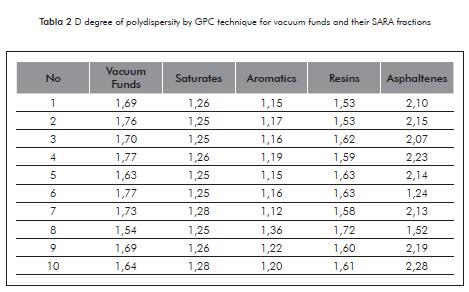
Moreover, if we compare the results obtained by GPC and simulated distillation can be observed that the difference in average molecular weights for each vacuum residue and its fractions are lower. Considering the results of this work and research done by Schabron and Speight (1998), it may indicate that the average by GPC and simulated distillation are more accurate, because these techniques to quantify and separate the molecules of a complex mixture in simple structures with minimal associations, compared with the results obtained by the VPO technique.
Table 3 illustrates the results of the association relation of the vacuum residue and their SARA fractions, obtained through the three techniques used. The molecular weight in each group of the saturated and aromatic fractions present similar tendencies, therefore it is evident that the molecular weights of the vacuum residue depend on the structural complexity and the percentage of resin and asphaltene fractions.
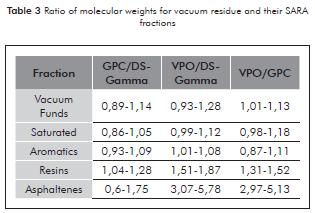
The ration of molecular weight in vacuum residue and its SARA fractions in respect to the GPC and DS-Gamma techniques allow proof that their results are within an allowed interval with similar tendencies, since these two techniques are considered of high resolution and their results correspond to the average of a distribution of chemical species with a wide spectrum of sizes.
Table 4 reports the parameters of the Gamma distribution function for determining the molecular weight of vacuum residue and their fractions SAR. In the appendix A to illustrate the trends of the probability density function adjusted to the distillation curve and the Gamma distribution.
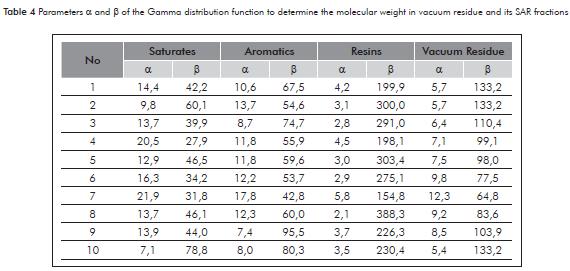
The ration of molecular weight amongst the VPO, GPC and DS-Gamma techniques show that the three techniques allow the attainment of values within an acceptable range in vacuum residue and its saturated and aromatic fractions with results consistent with other published works. However, we place in evidence that the ratio in respect to molecular weights of resins and asphaltenes through the VPO technique present significant differences due to aggregation effects.
In this work it is evident that the average molecular weight of vacuum residue and their SARA fractions varies with the experimentation conditions, and the technique used for measurement, which may present differences with wide orders of magnitude.
The results found show that the techniques studied can be applied in determining the molecular weights of vacuum residue and saturate, aromatic, and resin fractions with readings within an admissible range. Meanwhile, to determine the molecular weight of asphaltene fractions, we recommend using distribution techniques that allow the attainment of a gradual distribution of molecular sizes.
4. CONCLUSIONS
- The results obtained in this work indicate that the average molecular weight for vacuum residue and their SARA fractions depend on the analytic technique used.
- The difference between the results of molecular weight is greater when the VPO technique is used, in comparison to the GPC and simulated distillation adjusted to the Gamma function techniques, due to the association effects.
- To determine molecular weights of complex fractions such as resins and asphaltenes through GPC and VPO, it is necessary to use pattern samples with tendencies similar to the nature of the fractions to be analyzed.
ACKNOWLEDGEMENTS
This research was supported by ECOPETROL S.A - Instituto Colombiano del Petróleo (ICP)-[Colombian Institute of Petroleum].
REFERENCES
Akbarzadeh, K., Alboudwarej, H., Ayatollahi, S., & Yarranton, W. (2004). Estimation of SARA fraction properties with the SRK EOS. JCPT. 43 (9): 31-39. [ Links ]
ASTM D4124-09. Standard Test Method for Separation of Asphalt into Four Fractions. Anual Book of Standard, ASTM International, http://www.astm.org., West Conshohocken, PA, 2003. [ Links ]
ASTM D6352-04. Standard Test Method for Boiling Range Distribution of Petroleum Distillates in Boiling Range from 174 to 700°C by Gas Chromatography. Anual Book of Standard, ASTM International, http://www.astm.org., West Conshohocken, PA, 2009. [ Links ]
ASTM D2007. Standard Test Method for Characteristic Groups in Rubber Extender and Processing Oils and Other Petroleum-Derived Oils by the Clay-Gel Absorption Chromatographic Method. Anual Book of Standard, ASTM International, http://www.astm.org., West Conshohocken, PA, 2003. [ Links ]
Behrenbruch, P., & Dedigama, T. (2007). Classification and characterization of crude oils based on distillation properties. J. Petro. Sci. Eng. 57, 166-170. [ Links ]
Borges, R., Belsay, C., & Acevedo, S. (2007). Caracterización estructural de distintas fracciones aisladas del crudo extrapesado Carabobo. Revista Latinoamericana de Metalurgia y Materiales. 27(2): 83-94. [ Links ]
Buenrostro, E., Andersen, S., García, J., & Lira, C. (2002). Solubility/molecular structure relationships of asphaltenes in polar and nonpolar media. Energy & Fuels., 16 (3): 732-74. [ Links ]
Castellanos-Borrero, E., & Garrido, A. (2009). Desulfuración de diesel de bajo azufre de una planta de fraccionamiento de líquidos de gas. Ingeniería Química. 477, 62-69. [ Links ]
Cervo, E. G., & Thies, M. C. (2010). Control of molecular weight distribution of petroleum pitches via multistage supercritical extraction. J. of Supercritical Fluids. 51, 345-352. [ Links ]
Gacén, J., Maíllo, J., Knott, J., & Altenhofen, U. (1985). Interpretation of the differential solubility of poly (ethylene terephthalate) in function of temperature. Bol. Intextar. 87, 51-60. [ Links ]
Golam, R., & Mansouri, S. (2006). Cubic EOS calculates heavy oil SARA fractions. Oil and Gas J. 11, 48-49. [ Links ]
Guangua, Yang & Ren-And, Wang (1999). The supercritical fluid extractive fractionation and the characterization of heavy oils and petroleum residua. J. Petro. Sci. Eng. 22, 47-52. [ Links ]
Guzmán, A., Bueno, A., & Carbognani, L. (2009). Molecular weight determination of asphaltenes from Colombian crudes by size exclusion chromatography (SEC) and vapor pressure osmometry (VPO). Petroleum Science and Technology, 27 (8): 801-816. [ Links ]
Hunt, Jerry-E., & Winans, Randall-E. (1997). Characterization of asphaltenes from processed resids. Preprint, for presentation at the 213th ACS National Meeting to be held in San Francisco, CA., April 13-17. [ Links ]
Keng, C., Chunming, X., Yunxiang H., & Renan, W. (1997). Supercritical fluid extraction reveals resid properties. Oil & Gas J. 20, 66-69. [ Links ]
Kharrat, A. M. (2009). Characterization of Canadian heavy oils using sequential extraction approach. Energy & Fuels, 23, 828-834. [ Links ]
León, B. A., Parra, M. R., & Grosso, J. L. (2008). Estimation of critical properties of typically Colombian vacuum residue SARA fractions. CT&F - Ciencia, Tecnología y Futuro, 3 (4): 129-142. [ Links ]
Masuda, K., Okuma O., Kanaji M., & Matsumara, T. (1996). Chromatographic characterization of preasphaltenes in liquefied products from Victorian brown coal. Fuel, 75 (9): 1065. [ Links ]
Mullins, O. C., Sheu, E. Y., Hammami, A., & Marshall, A. G. (2007). Asphaltenes, Heavy oils and petroleomics. New York: Springer: 21-26. [ Links ]
Navarro, L., Álvarez, M., Groso, J., & Navarro, U. (2004). Separación y caracterización de resinas y asfaltenos provenientes del crudo Castilla. Evaluación de su interacción molecular. CT&F - Ciencia, Tecnología y Futuro. 2 (5): 52-67. [ Links ]
Parra, M., & Cañas, W. (2007). Thermodynamic model for solvent deasphalting of vacuum residue. Proceedings annual meeting, AIChe J., Nov. 4. [ Links ]
Peramanu, S., Pruden, B., & Rahimi, P. (1999). Molecular weight and specific gravity distributios for Athabasca and cold lake bitumen and their satúrate, aromatic, resin, and asphaltene fractions. Ind. Eng. Chem Res., 38 (8): 3121-3130. [ Links ]
Sabbagh, K., Akbarzadeh, K., badamchi-Zadeh, A., Svrcek, W. Y., & Yarranton, W. (2006). Applying the PR - EOS to asphaltene precipitation from n-alkane diluted heavy oils and bitumens. Energy and fuels. 20, 625-634. [ Links ]
Schabron, John-F., & Speight, James-G.(1998). Correlation between carbon residue and molecular weight. Petrol. Science and Technology, 16, 361-364. [ Links ]
Sheua E. Y., De Tara M. M., & Storma D. A. (1991). Reological properties of vacuum residue fraction in organic solvents. Fuel, 70, 1151-1156. [ Links ]
Tie-Pan, S., Yun-Xiang, H., Tong, S., & Ren-An, W. (1997). Characterization petroleum vacuum reidue by supercritical fluid extraction and fractionation. Ind. Eng. Chem. Res., 36; 3988-3992. [ Links ]
Wiehe, I. A., & Liang, K. S. (1996). Asphaltenes, resins and other petroleum macromolecules. Fluid Phase Equilibria, 117, 201-210. [ Links ]
Wu, J., & Preusnitz, M. (1998). Molecular Thermodynamic Framework for Asphaltene-Oil Equilibria, AIChe J., 44, 188-1199. [ Links ]
Yarranton, H. W., Alboudwarej, H., & Jakher, R. (2000). Investigation of asphaltene association with vapor pressure osmometry and interfacial tension measurements. Ind. Eng. Chem. Res., 39, 2916-2924. [ Links ]
Zhao, S., Zhiming, X., Chunming, X., Keng, H., C. & Renan, W. (2005). Systematic characterization of petroleum residua based on SFEF. Fuel., 84, 635-645. [ Links ]
Zhu, Y., Fu, J., Sheng, G., Xiang, T., & Bowen, M. (1998). Eochemical significance of pyrrolic nitrogen compounds in various kinds of crude oils from the Taim Basin. Chinese Science Bulletin, 43 (16): 1366-1370. [ Links ]
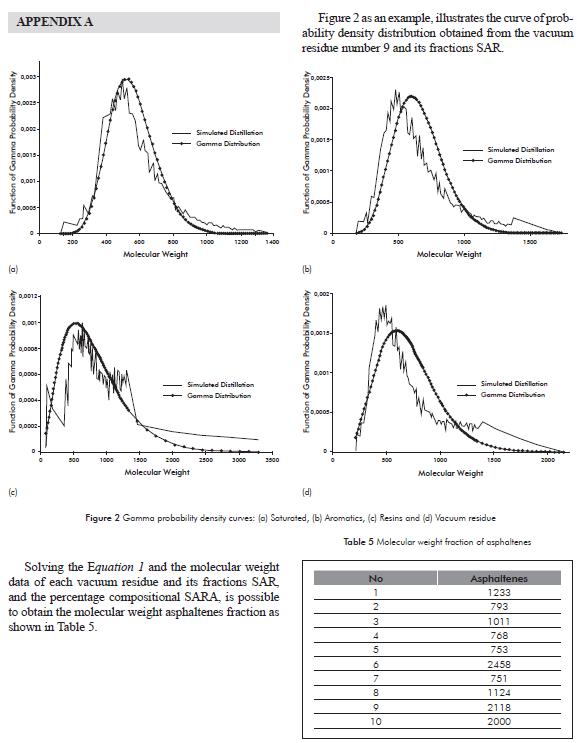