1. INTRODUCTION
In recent years, the need for enhanced energy has been directly proportional to the accelerated pace of population enlargement, the economy, and a fast evolution in both industry and transportation sectors (Khandal et al., 2022). So far, the energy demand issues are still linked to the energy acquired from fossil fuels, referred to as non-renewable fuels. At the same time, the transportation sectors are also growing with the national and global economies. In the past two decades, the steep rise in crude oil demand and its accompanying pollution are perceived as significant challenging issues all over the world (Yaqoob et al., 2022). Numerous extensive investigations have been conducted to minimize the emission of automobile combustion using diverse alternative fuels. Furthermore, the governments of all countries have established severe norms on emission rates to manage the pollution levels (Zhou et al., 2022). Nowadays, the use of hydrogen fuel to replace petrol and diesel has added extra curiosity among research scholars (Mohan et al. 2023). The emission rate of diesel engines causes environmental contamination that further results in the release of harmful gases and other particulate material, as added to various other insecure compounds (Sharma et al., 2020; Cavalcanti, 2021). Thus, due to the emission of such gases, air contamination occurs, thusresulting in global warming. The air pollution level produced by motor vehicles relies mainly on the use of fuel (Rangasamy et al., 2020; Feroskhan et al., 2021; Nayak et al., 2022).
At present, several investigations are underway aimed at minimizing the harmful impacts of exhaust emission gas derived from the use of diesel, LPG, and gasoline fuels. This is because using fossil fuels is harmful to human health and the environment, which leads tothe search for a substitute fuel (Sharma and Verma 2021). Gaseous biofuels are greatly favorable given the enhanced hydrogen-to-carbon ratio, minimum emission levels, and wider ignition limit, in addition to the formation of a homogenous blend (Yilmaz & Davis, 2016). Nowadays, a researchers are greatly focused on various fuels determined in the CI mode, namely hydroxyl gas, syngas, and liquefied petroleum gas (Yilmaz & Donaldson, 2007). The world's transport and agricultural industries are mostly dependent on internal combustion engines (ICE), particularly conventional engines. With the dramatic increase in cost, as well as fossil energy consumption in the current generation, different scientific studies are geared toward evaluating and discovering various renewable fuels (Ganesan et al., 2022; Sivakandhan et al., 2022). LPG is gaseous fuel, and it has been generally employed for commercial vehicles over the last decades. Hydrogen and LPG are also applied on stationery engines used for electrical power (Singh et al. 2018). The syngas is produced through the process of gasification, as well as for stationary engines. It is also comprised of natural gas, nitrogen, hydrogen, carbon monoxide (CO), and carbon dioxide (Senthur et al., 2022; Elumalai et al., 2022). Syngas is a burning oil that is renewable by nature. Energy assessments are important for optimizing the execution of Syngas and LPG fuelled gas (Kesharvani et al., 2023). Table 1 shows the nomenclature and descriptions of the article. The combustion in the fuel emission process is a complicated task, which is highly suitable for controlling emissions and energy management systems. The combustion process is used to estimate different fuel types and to determine the toxic properties that generate emissions. The emission is categorized based on the factors, and the data handling approaches are implemented with low temperature and the dual fuel to estimate the efficiency of fuel modes.
Moreover, in the past few years, researchers have been more interested in dual-fuel engines using hydrogen, compressed natural gas, LPG, biogas, syngas, etc. The compression ignition has a minimal effect on radiation from flame temperature. The exergy is determined to estimate the quality of energy in more demanding works. Several studies have been performed to evaluate the heavy impact derived from gas emissions. Different optimization techniques are introduced for analyzing the emission process with multi-objective techniques. The prediction process of emission parameters determined the initial heating process of diesel and investigated differentstrategies for emission control. However, the analysis of the durability rate affects the performance of emission reduction and greater expenses Therefore, a novel triple fuel system approach is proposed to show gas emissions in various gas emission processes. Also, it makes the engine loads work in stable conditions, which enhances the efficiency of the method.
NOVELTY
The demand for fuel consumption is maximized, which serves various purposes. However, the outer utilization affects the environment, and causes diverse effects all over the world. Nevertheless, various researches are performed to reduce generation of gas emission impacts such as LPG, gasoline, diesel, etc. Different investigations were undertaken that reduced the emission by gas recirculation rate and efficient training was provided , reducing the error rate. Nonetheless, the minimization of the durability rate affects the performance and error is reduced using huge datasets. Thus, a novel triple fuel system is proposed that extracts the impacts determined in fuels, and avoids the gas emission through validation at different load conditions. In this estimation, six diverse engine loads are considered for measuring the performance based on different parameters. Thus, the proposed method validated the emission process based on different loading conditions, and determined the efficiency by comparing parameters.
Jabbr et al. (2020) established an optimization technique with dual fuel parameters (i.e. diesel and hydrogen) compression ignition engine. In this study, various factors, namely the injection timing of a diesel engine, and the exhaust gas recirculation rate were considered. Using a multi-objective technique, various emissions such as nitrogen oxides and soot emissions were minimized, while the efficiency was maximized. Thermal efficiency, soot rate, and NOx rates were analyzed and determined. However, this technique failed to determine a durability rate, which significantly affects the theoverall system performance..
The prediction of emission parameters of a compression ignition engine containing a preheated bio gas-air mixture ,as well as diesel, was discussed by Prabhu et al. (2021). In this case, performance was predicted using an artificial neural network (ANN), and a few parameters such as correlation coefficients, mean square error, and brake thermal efficiency, all of which were analyzed and plotted. Further, the actual value and the predicted value were mapped using the Levenberg-Maequardt training method. The error values were calculated and the obtained error rate between the actual value and the predicted value was determined, and the output was obtained with minimal error. On the other hand, the high cost and time were significant limitations to this approach.
Karthic and Kumar (2021) investigated a hydrogen bio-fueled reactivity-controlled compression engine system using an open engine control unit system (ECU) system. This work systematically investigates diverse strategies to minimize diesel engine emissions. Its main purpose is to enhance the compression ignition engine by substituting diesel with biofuel. Various types of emissions such as hydrogen oxides, and carbon monoxide, and various parameters like brake power, brake thermal efficiency rate, ignition rate, and the heat release rate were analyzed and discussed.
The genetic algorithm II is determined by the methanol reactivity compression engine Jing et al. (2021). Three different types of analysis, namely brake-specific emission analysis, brake thermal efficiency, and reactivity-controlled compression ignition, were performed on a diesel engine. Pressure rate, brake thermal efficiency, diesel injection timing, and co-combustion ratio were the parameters used. The performance evaluations were accomplished, and the overall performance was effective and feasible; however, this technique failed to determine other combustion modes.
Colakoglu & Durmayaz (2021) proposed the exergy, energy, and environmental-based model using a solar-driven multi-generation technique. The solar-driven multi-generation methods are evaluated with energy exergetic quality factor (EQF), energy, exergoenvironmental, and environmental measures. The experimental results showed that the system acquired exergy efficiency (39.45%), EQF values (50.83%), and energy efficiency (55.57%). The multi-objective optimization has enhanced the performance of multi-generation systems.
An experimental analysis to investigate the consequence of direct water injection based on emission, knock, and combustion for dual fuels (i.e. diesel and LPG) was established by Ayhan (2021). In this work, the nitrogen oxide emission and knock intensity were minimized using the electronic control technique. It is considered that the ratio of LPG was greater than 30%, thereby preventing the damage while operating under full load (kg) conditions. The performance measures were evaluated, and the investigation analysis revealed that the fuel combustion rate was minimized, and the knock intensity rate was enhanced.
Esmaeilpour-Troujeni et al. (2021) formulated the rapeseed production optimization applying the exergy analysis method. The Multi-Objective Genetic Algorithm (MOGA) and Support Vector Machine (SVM) were used for optimizing and predicting the systems for rapeseed production. Hence, the consumption of irrigation water was reduced, while diesel fuel, chemical fertilizers, and farmyard manure were enhanced by 24.56%.
Table 2 Summary of existing works
Author and Year | Methods | Objective | Merits | Demerits |
---|---|---|---|---|
Jabbr et al. (2020) | multi-objective optimization technique | To study diverse factors namely injection timing of a diesel engine, and exhaustible gas recirculation rate | Nitrogen oxides and soot emissions were minimized, and the efficiency was maximized | Failed to perform a durability rate |
Prabhu et al. (2021). | Artificial neural network (ANN) and Levenberg-Maequardt training method | Predict parameters of emission from compression ignition engine | Output was obtained with minimum error | High cost and long time |
Karthic and Kumar (2021) | ECU system | Investigates diverse strategies to minimize diesel engine emissions | Emission rate was minimized | Trouble during investigation |
EsmaeilpourTroujeni et al. (2021) | Solar-driven multi-generation technique | To evaluate different efficiencies | Fuel combustion rate was minimized, and the knock intensity rate was enhanced | Time consumtion rate was high |
Ayhan (2021) | Electronic control technique | To investigate the effect of direct water injection based on emission, knock as well as combustion for dual fuels | Enhanced performances of multi-generation systems | Complexity was high |
Sharma et al. (2021) | Multi-Objective Genetic Algorithm (MOGA) | For optimizing and predicting the systems for rapeseed production | Minimum fuel utilization | Energy required was high |
Rajendran et al. (2020) | The fuel named Calophyllum inophyllum biodiesel | improving the utilization of LPG | Enhanced performance | The emission rate of nitrogen oxides was enhanced |
Singh et al. (2021) | Reactivity-controlled compression ignition (RCCI) | To investigate both high and low-reactivity fuel | Gases emission was minimized | Overall performance was low |
Subramanian and Thangavel (2020) | Experimental investigations on the emission | To perform experiments at five diverse torque conditions containing air in the inlet manifold | Maximum torque rate | Minimum durability |
Raja et al. (2022) | Taguchi method | Validate the stability of the ignition engine | Minimized emission | Maximized the expense rate |
Taghavifar and Perera (2023) | ANN | To extract ideal fuel from multiple features | Improved energy efficiency | Not determined renewable energy fuels in network factor |
Rajendran et al. (2020) explained the process of improving the utilization of LPG operated with a renewable fuel named Calophyllum inophyllum biodiesel under the concept of dual fuel. Various parameters, namely smoke opacity, fuel consumption, uncertainty, and overall brake power were formulated, and the values were computed and compared with diesel fuel. The experimental results revealed that both, the performance rate and the emission rate were enhanced accordingly. On the other hand, the emission rate of nitrogen oxides was enhanced, although degrading the system's performance.
The evaluation based on the Reactivity-Controlled Compression Ignition (RCCI) mode combustion engine utilizing gasoline and diesel fuels was demonstrated by Singh et al. (2021). In this paper, both high and low reactivity fuel was investigated for diesel and gasoline fuel based on the RCCI combustion mode. Furthermore, the particulate mass value and premixed addition ratio were evaluated, showing that the emission of gases was minimized simultaneously using RCCI instead of conventional CI engines.
Subramanian and Thangavel (2020) conducted experimental investigations on the emission, combustion, and performance characteristics of two different types of fuels, that is, diesel HHO gas and diesel hydrogen gas. The experiments were performed at five diverse torque conditions, containing air in the inlet manifold. Pressure rate, brake thermal efficiency, combustion duration, and carbon dioxide emission rates were evaluated. Making a better use ofexcess air, the brake thermal efficiency was minimized, and the torque rate was maximized.
Raja et al. (2022) discussed the evaluation of the the ignition engine condition based on the Taguchi method. The carbon nanotubes blended the fuel at different engine load conditions, and showed the association of varied ignition temperature levels. The obtained method validated the performance with a minimized emission rate and thus the gas emission is reduced, making the load condition stable. However, it required more time, and maximized the expense rate to obtain the best feasible operation.
Taghavifar and Perera (2023) illustrated the classification of energy exergy in the combustion modeling process. The Artificial Neural Network (ANN) is determined to examine various fuel types and extract the ideal fuel mixture. The combustion fuel is transmitted to exergetic parameters and validated with neural networks. Hence, the ANN approach enhanced energy efficiency and minimized the extraction of emissions. However, it does not include renewable energy fuels in the output network factor. The nomenclature and its explanations are provided in Table 2.
In this paper, three different analyses namely energy, exergy, and emission are studied for triple fuel mode (i.e. petrol, diesel, and LPG) at varying load (kg) conditions.
The major contribution of the article is described below.
To present a novel triple fuel mode at varying load (kg) conditions based on three different analyzing parameters, namely energy, exergy, and emission.
Various measurable factors such as emission rate, heat transfer rate, and cylinder pressure, are evaluated for the proposed model at steady-state conditions.
The analysis based on different exhaust gases, namely hydrocarbons, carbon monoxides, nitrogen oxides, as well as smoke released by the engine side is evaluated.
The remaining sections include: In section 2, the material selection and experimental setup with its specifications are presented. In section 3, a novel triple fuel mode is proposed and formulated. Section 4 discusses the experimental results, and section 5 concludes the article.
2 MATERIALS AND METHODS
In this case, the experimental evaluation is performed at a constant speed of about 1500rpm, containing six diverse engine loads (kg) of about (2.5, 5, 7.5, 10, 12.5, and 13.50 kg). Various measurable factors such as emission rate, cylinder pressure, and heat transfer rate are recorded at steady-state conditions.2.1.
EXPERIMENTAL PROCEDURE
In this paper, the experimentation is conducted with a 5.5 KW Kirloskar TV 1 engine intended for generating 3.5 kW at full load (kg) employing Apex Innovation India Ltd. The engine performance s analyzed using an additional software named "ENGINESOFT". The engine emission rate is measured using AVL Dismoke 480 BT and AVL Digas. Additionally, the smoke rate is measured using opacity (in %), and various exhaust emissions are measured in ppm and volume (%). Table 3 shows test engine details, and Figure 1 illustrates the pictorial representation of the test engine.
Table 3 Test engine details
S.No. | Factors | Units | Descriptions and Ranges |
---|---|---|---|
1 | Engine type and model | - | Research engine |
2 | Total number of cylinder | - | 1 |
3 | Rated speed | rpm | 1500 |
4 | Compression ration | - | 21:01 |
5 | Displacement volume | cc | 661 |
6 | Stroke | mm | 0.11 |
7 | Total power rated | kW | 3.1 |
8 | Bore | mm | 0.27 |
9 | Injection pressure and timing | bar/0btdc | 210 and 20 |
10 | Type of fuel injection | - | Direct mechanical type |
11 | Intake opening and closing valve | °abdc/°btdc | 35.5 and 4.5 |
12 | Exhaust opening and closing valve | °abdc/°btdc | 35.5 and 4.5 |
UNCERTAINTY EVALUATION
The particulars of the apparatus comprising the measuring variable, its descriptions, uncertainty, resolutions, as well as ranges are portrayed in Table 4. Various uncertainty parameter values are formulated as:
Table 4 Uncertainty Description
S.No. | Factors | Apparatus | Rate of uncertainty (mission) |
---|---|---|---|
1 | Temperature | Temperature sensor | 1 |
2 | Pressure | Piezo sensor | 0.3 |
3 | Engine RPM | PPR rotary encoder | 1 |
4 | Load (kg) | Strain gauge | 1 |
5 | Smoke | AVL Dismoke 480 BT | 1.5 |
6 | Brake power | Computed | 0.5 |
7 | Brake-specific energy consumption | Computed | 0.85 |
8 | Brake thermal efficiency | Computed | 0.7 |
9 | Carbon monoxide | AVL gas 444 | 2.6 |
10 | Nitrogen oxide | AVL gas 444 | 2.1 |
11 | Hydrocarbons | AVL gas 444 | 2.3 |
From equation(1), the term a represents the function of x 1 , x 2 …x N1 , x N . The partition values for the computed factors namely x 1 , x 2 …x N-1 , x N are denoted by (∂a/∂x 1 ) Δx 1 , (∂a/∂x 1 ) Δx 2 … (∂a/∂x N - 1 ) Δx N - 1 as well as (∂a/∂x N ) Δx N respectively. x1, x 2 …x N-1 , x N are diverse computed variables and Δx 1 , Δx 2 , Δx N-1 as well as Δx N are deviation variables respectively.
3 PROPOSED ENERGY AND EXERGY ANALYSIS BASED TRIPLE FUEL SYSTEM
In this article, three different analyses, namely energy, exergy, and emission are studied for a novel triple fuel mode (i.e. petrol, diesel, and LPG) at varied load (kg) conditions and in an optimized function based on a four-stroke diesel engine. The detailed mathematical expression proposed is formulated in the following sections.
ENERGY EVALUATION FOR THREE FUEL MODES
In this experimental evaluation, three diverse combustion cases are considered (a) single fuel mode (i.e. diesel fuel), dual fuel mode (i.e. petrol and LPG), and triple fuel modes (i.e. diesel, LPG, and petrol). The following equations provide the input fuel energy provided to the engine for three different cases.
For the single fuel mode, the input fuel energy E lSP supplied to the engine is
For the dual fuel mode, the input fuel energy E IBP supplied to the engine is (Sharma et al. 2020)
For the triple fuel mode, the input fuel energy E LTP supplied to the engine is
From equations (2) to (4), the flow rate of diesel, petrol, and LPG is denoted by M B , M P and M LPG in kg/s respectively. The low calorific rate of diesel, petrol, and LPG measures in MJ/kg is Lcv B , Lcv P and Lcv LPG
The following mathematical expressions are employed in converting the load (kg) measured in kg to brake power B p (kW) or torque T (N-m). Therefore, the engine torque is defined as,
The mathematical formula for determining the output shaft power is depicted in equation (6),
A predetermined quantity of energy gets wasted during the exhaust process and it can be obtained using the following equations.
For the single fuel mode, the output fuel energy in the exhaust gas E O,SF,EG is
For the dual fuel mode, the output fuel energy in the exhaust gas E O,SF,EG is
For the triple fuel mode, the output fuel energy in the exhaust gas E O,SF,EG is
In addition, three heat transfer modes, that is, conduction, convection, as well as radiation are evaluated to determine the heat loss analysis. Therefore, the general mathematical expressions are generated hereinafter,
The heat loss rate for conduction mode to the outer wall t OUT,WALL from the inner wall t IN,WALL is
The heat loss rate for the convection mode to the inner wall from the in-cylinder burnt gas t IN,BG is determined in equation (11).
The heat loss rate for the radiation mode to the surrounding area from the in-cylinder bumted gas is,
Therefore, the total heat loss from the three different modes is expressed in equation (13). Then,
The mathematical expression involved in deriving the overall heat loss is,
From equation (14), H TC mean the heat transfer coefficient. In accordance with the energy consumption (i.e. fundamental energy assumption), the input energy and the total output energy are analogous. The following equations depict the unaccounted energy loss in the engine for three fuel modes. Thus,
For single fuel mode, the unaccounted energy E SF,UA is,
For the dual fuel mode, the unaccounted energy E BF,UA is,
For the triple fuel mode, the unaccounted energy E TE,UA is,
To compute the brake thermal efficiency (BTE), the following equations are employed for three different types of modes.
For single fuel mode, BTE depicts,
For the dual fuel mode, BTE represents,
For the triple fuel mode, BTE characterizes,
For computing energy loss based on exhaust gas energy loss, the following equations are provided for three different types of modes. Thus,
For the single fuel mode, the exhaust gas energy loss expressed in percentage is,
For the dual fuel mode, the exhaust gas energy loss expressed in percentage is,
For the triple fuel mode, the exhaust gas energy loss expressed in percentage is,
The total heat transfer loss and unaccounted energy concerning three different modes are expressed in the following equations.
For the single fuel mode, the total heat transfer loss and unaccounted energy is
For the dual fuel mode, the total heat transfer loss and unaccounted energy is
For the triple fuel mode, the total heat transfer loss and unaccounted energy is
EXERGY EVALUATION FOR THREE FUEL MODES
The term exergy is defined by thermodynamics second law, which symbolizes the highest useful work obtainable from a system. The exergy evaluation is considered as a vital process that recognizes the inefficiency proportion in the thermal system. To simplify the exergy evaluation , two different ambient factors, pressure and temperature, containing 101.325 kPa and 28.15 K were used as the reference state. The following expression describes the balanced chemical equations for various gases (Dogan et al., 2020).
From equation (30),
The term ψ F1 , ψ F2 and ψ F3 means the molar flow rate of fuels Where
From equations (34) to (36), m w(F1) , m w(F2) and m w(F3) show the molecular weight of fuels.
The mass fraction of oxygen, carbon dioxide as well as water is determined using the following expressions.
From the above equations, the molar fraction and the total number of moles of various gases are represented by P 1 , Q 1 R 1 and M 1 , M 2 , M 3 respectively. The total number of hydrogen and carbon atoms for fuels is represented by x 1 , x 2 ,y 1 and y 2 respectively. N signifies the total number of moles. Equation (41) provides the change in the exergy rate.
Then the input fuel exergy equations for three fuel modes (i.e. single fuel, dual fuel as well as triple fuel modes) are defined in the following equations; where the molecular mass of various elements such as hydrogen, oxygen, carbon, and sulfur is denoted by H, O, C, and S respectively.
For the single, dual, and triple modes the input fuel energy supplied to the engine is formulated as
From equations (44) to (46), the exergy rate of diesel, petrol, and LPG measured in kJ/kg isε P , s LPG and ε D respectively.
Equation (47) depicts the work transfer equation of exergy analysis.
Then the input exergy fuel rate concerning loss based on exhaust gas exergy is,
From the above equation, M EG represents the mass flow rate measured in kg/s. C PMG , t EG , p EG and G represents the specific heat, temperature, pressure, and the as gas constant respectively. Then the input fuel exergy rate concerning heat transfer exergy loss is defined in equation (49).
From the above equation, the heat transfer rate at an instant mode is ϑ INS .
From the above equation, the total cylinder area and the cylinder diameter are represented by a t and DC respectively. çv and v symbolizes the calibration constants. T IN,GAS andt WALL represents the temperature inside the gas and the wall temperature respectively. The mathematical term involved in determining the exergy destruction rate is stated below.
According to the thermodynamics second law, the exergy efficiency is,
Then the exergy performance coefficient together with the exergy destruction factor is defined by,
Therefore, the mathematical expression to determine the diesel substitution is obtained in equation (54),
EMISSION EVALUATION
The emission evaluation based on various factors is explained in sections herein below.
HYDROCARBON EMISSION: In general, hydrocarbon is emitted due to the presence of HC gas in the inner wall of the cylinder. The general expression to formulate the emission of HC gas is discussed in equation (55) (Purushothaman & Nagarajan, 2009).
From equation (55),
S
E
signifies the service emission index.
V
ß(HC)
represents the unburnt hydrocarbon.
OX
and HT, indicates the fraction of oxidation as well as the fraction of oxidation exhaust.
CARBON DIOXIDE EMISSION: The existence of the emission stems from the presence of various gaseous fuels, and the combination of dilute fuels present in the air leads to a progressive increase in carbon dioxide emissions. The preheat air ingestion decreases the carbon dioxide level thereby enhancing the velocity rate (Gopal & Karupparaj, 2015).
CARBON MONOXIDE EMISSION: Carbon monoxide emission significantly relies on three vital factors namely carbon, oxygen, and combustion efficiency. During the combustion procedure, the fuel containing carbon is oxidized to obtain carbon dioxide. The combustion process is incomplete if the level of oxygen content is minimal resulting in greater emission of carbon dioxide gas. Additionally, the HC gas containing oxygen and hydrogen content is considered the most common reason for carbon monoxide emission (Devarajan et al., 2021).
NITROGEN OXIDES: The emission of nitrogen oxides depends highly on three major aspects namely time, cylinder temperature as well as oxygen. The mathematical term to determine the nitrogen oxide is computed using the Zeldovich mechanism.
From the above equation, the concentration of species at equilibrium is C 1 and C2.
From equation (56),
The term f rr in equation (57) represents the forward reaction rate and in equation (58), b rr(1) and b rr(2) the backward reaction rate (Harari et al., 2021).
4 EXPERIMENTAL RESULTS
The results and analysis of experimental observations for the three fuel modes (i) single fuel (ii) dual fuel as well as (iii) triple fuel are discussed in the following subsections. The real-time experimental step is shown in Figure 2.
BRAKE THERMAL EFFICIENCY (BTE) ANALYSIS
It is calculated by measuring the efficiency of the fuel of an internal combustion engine. In addition, enhancing the performance of the thermal efficiency significantly minimizes the cost and energy use. Here, the results are performed at a constant speed of about 1500rpm with six diverse engine loads (kg) of about (2.5, 5, 7.5, 10, 12.5, and 13.50 kg). Figure 3 portrays the brake thermal efficiency for various loads (kg) as mentioned above. Here, the x-axis represents the BTE, and the varying load (kg) is represented on the y-axis. The BTE with various loads (kg) at constant engine speed is compared for diesel, diesel with hydroxyl gas (HHO) (Sharma et al., 2020), diesel with compressed natural gas (CNG) (Pathak et al., 2021), diesel with hydrogen gas (HG) (Kokabi et al., 2021), diesel with biodiesel (Raja et al., 2022) and the proposed approach. From the figure, it is determined that for the load (kg) with the whole range, the dual fuel mode of BTE is minimal when compared with other fuel modes. As the load (kg) enhances, the BTE is enhanced due to the presence of the fuel-air mixture and the base fuel quality inside the eogioe cylinder.
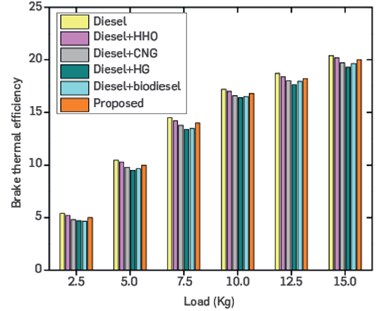
Figure 3 Evaluation results for brake thermal efficiency 4.2. Brake-Specific Fuel Consumption (BSFC) Analysis
The graphical representation of BSFC is delineated in Figure 4. The various load (kg) condition values vary from 2.5, 5, 7.5, 10, 12.5, and 13.50 kgs respectively. The experimental analyses are carried out at a constant speed rate of about 1500rpm. If the BSFC is low, the engine becomes more efficient. Similar to the BTE, the BSFC with various loads (kg) at constant engine speed is compared for diesel with HHO, CNG, HG, and biodiesel. The running cost of the engine is minimized in high load conditions compared with diesel fuel and triple foel.
ANALYSIS BASED ON EXHAUST ENERGY
In this case, the analysis based on different exhaust gases, i.e. hydrocarbons, carbon monoxides, nitrogen oxides, and smoke released from the vehicles on the engine side is evaluated briefly.
HC EMISSION ANALYSIS: In Figure 5, the evaluation results are based on determining the emission rate of hydrocarbons measured in parts per million (ppm) with respect to various loads (kg), where the load (kg) value varies from 2.5, 5, 7.5, 10, 12.5, 13.50 kgs. The HC emission rate with various loads (kg) at constant engine speed is compared for diesel, diesel with hydroxyl gas (HHO), diesel with compressed natural gas (CNG), diesel with hydrogen gas (HG), diesel with biodiesel, and the proposed approach. Furthermore, minimum flame speed and poor scavenging of the air-fuel mixture enhance the HC emission rate. The emission generated from HC is maximized in both the dual and triple fuel models when compared with diesel fuel (i.e. single fuel mode).
CO EMISSION ANALYSIS: The graphical analysis based on determining the emission rate of carbon monoxide measured in % with respect to various loads (kg), namely 2.5, 5, 7.5, 10, 12.5, and 13.50 kgs is represented in Figure 6. The CO emission occurs mainly when the air and diluted fuel are mixed, which further results in rapid enhancement of CO emission. Additionally, preheated air ingestion reduces the carbon dioxide level thereby enhancing the velocity rate. The results analyzed proved that CO emission is higher for both dual fuel and triple fuel modes when compared with diesel fuel.
NOX EMISSION ANALYSIS: In general, nitrogen oxide emission analysis involves two different mechanisms, namely the Fenimore or fast-growing mechanism, and the Zeldovich mechanism or thermal mechanism. In a fast-growing mechanism, the temperature rises to the maximum range of about 2000 Kelvin containing oxygen with the available amount. The formation of nitrogen oxide emission is mainly due to oxygen-containing excessive availability as well as minimum premixed combustion rate. This further leads to a high burning rate and enhanced temperature in the combustion chamber. Figure 7 represents the graph based on NOx emission with respect to various loads (kg), where the load (kg) value varies from 2.5, 5, 7.5, 10, 12.5, and 13.50 kgs respectively. Similar to other emission gases, the experimental analysis conducted revealed that the NOx emission is high for both dual fuel and triple fuel modes when compared with diesel fuel.
Table 5 depicts the parameter analysis of various fuel modes. The validation is estimated by varied load conditions to evaluate the effectiveness of single, dual, and triple fuel modes.
High emission of smoke results in damage to the engine. The formation of soot and incomplete combustion process occurs due to certain reasons (a) when there exists an overabundance of fuels, (b) in the availability of excessive oxidants, (c) inadequate residence time, etc. In addition, over-fueling may result in the emission of black smoke from the exhaust of heavy-duty engines. The longer combustion duration provides sufficient time to finish the combustion process thereby minimizing the thermal cracking effect. Figure 8 shows the smoke emission analysis with respect to various loads (kg), and the analysis of graphical results suggests that the smoke emission is high for both dual fuel and triple fuel modes when compared with diesel fuel.
Figure 9 (a) (b) (c) and (d) depict error analysis of various exhaust gas emission characteristics of HC, CO, NOx, and smoke. The increase in engine loads maximizes the emission as well as the cyclic injection quantity, while the inlet air quality remains stable. The emission generated from biodiesel is low compared to diesel. The HC and CO emissions at various loads are diminished by enhancing the loads. The minimization of fuels maximized the emission generated from NOx and thus increased the power with a high number of loads. The analysis of HC, CO, NOx, and smoke is processed based on 1.5, 3.5, 5.5, 7.5, 9.5,11.5, and 13.5 Loads in kg.
Figure 10 depicts the validation of brake power in the emission of fuel based on varied diesel factors. The brake-specific fuel consumption s diminished when the mass flow rate is maximized. The comparison is performed using different types of diesel with varied parameters, and the performance is maximized by increasing the efficiency of the proposed method.
DISCUSSION
The utilization of fuel consumption is maximized nowadays, and t is analyzed by compression ignition engine. Different flow rates are distributed to estimate the effects determined by the global economy. It is significant to reduce the emission of gas due to the utilization of fuels. The fuel rate is analyzed by the proposed triple fuel system model that determines the amount of emission extracted at varied load conditions. Its attainment is oba challenge to minimize the emission and reach stable conditions of the measurable factors. The effectiveness of the proposed model is evaluated by comparing it with single, dual, and triple fuel modes. It achieves a better efficiency in exhaust gas validation at varied load conditions. However, the negatives obtained before the fuel consumption are not determined, and the impacts determined in fuel mixtures are not used.
CONCLUSIONS
Recently, the utilization of fuels has been maximized, translating into huge emissions released from LPG, gasoline, diesel, etc., which contaminates the environment. Thus, to overcome the impacts generated by harmful gases, a novel triple-fuel system is proposed to analyze the formation of emissions at varied load conditions. The evaluations for maintaining the stable condition are performed by single cylinder four-stroke diesel engine at optimized condition. The "ENGINESOFT" software is used to validate the efficiency of the model and to show the emission rate. To perform such validation, six varied engine load conditions are determined such as 2.5, 5.0, 7.5, 10.0, 12.5, and 13.5 at a constant speed rate of 1500 rpm. The engine loads measure heat transfer rate, cylinder pressure, and emission rate by comparing with brake thermal efficiency, brake-specific fuel consumption, and exhaust gas analysis. The proposed method attained 4.8, 9.9, 13.7, 15.9, 17.3, and 19.5 at different load conditions. The BSFC for various loads of the proposed approach is 1.41, 0.87, 0.54, 0.34, 0.30, and 0.33 respectively. The experimentation results revealed that the validation of the proposed method based on the parameters attained at different load conditions and for load 13.5 achieved 19.5, 0.33, and for exhaust gas analysis the HC, CO, and NOx are 11.3, 0.0237, and 368 enhanced the efficiency. In the future, it will measure the impacts before using the fuels and will validate the flow rate with different optimized approaches. Furthermore, it assesses the economic impacts determined by fuel mixtures.