Services on Demand
Journal
Article
Indicators
-
Cited by SciELO
-
Access statistics
Related links
-
Cited by Google
-
Similars in SciELO
-
Similars in Google
Share
Ciencia y Tecnología Agropecuaria
Print version ISSN 0122-8706On-line version ISSN 2500-5308
Cienc. Tecnol. Agropecuaria vol.21 no.2 Mosquera May/Aug 2020 Epub Mar 30, 2020
https://doi.org/10.21930/rcta.vol21_num2_art:1193
Plant genetics and biodiversity
Evaluation of sucrose and GA3 in an in vitro shoot culture of Alpinia purpurata (Zingiberaceae)
1Universidad de Córdoba. Montería, Colombia.
2Universidad de Córdoba. Montería, Colombia.
3Universidad de Córdoba. Montería, Colombia.
Alpinia purpurata is an ornamental plant species native to the Asia-Pacific with commercial potential due to its red inflorescence adapted to the northern Colombian coast; however, the lack of propagation protocols hinders commercial cultivation. To adjust a micropropagation protocol for clonal plant material production, the aim of this study was to evaluate the effect of sucrose and gibberellic acid (GA3) in the culture medium on the number of new shoots per explant, shoot length, root number, root length and number of leaves. Explants were cultured in semisolid MS (Murashige & Skoog) medium with sucrose (30, 45 and 60 mg/L) and GA3 (0.0; 0.25; 0.5; 0.75 and 1.0 mg/L). A factorial experimental design with two factors was employed, with 15 treatments, 10 replicates per treatment and 150 experimental units distributed with a completely randomized design. Cultures were stored five weeks at 25 °C with a 12 h photoperiod (40 μmol m-2s-1). Anova and Tukey’s test allowed detecting the statistical effects of sucrose and GA3 supply for all variables. An increase in sucrose concentration resulted in a higher number of shoots, while an increase in GA3 induced longer shoots. Root length and leaf number decreased as GA3 and sucrose increased.
Keywords inflorescence; micropropagation; ornamental plants; plant growth substances; red ginger
Alpinia purpurata es una planta ornamental nativa de las islas del Pacífico asiático con potencial por su inflorescencia roja, adaptada a las condiciones de la costa Atlántica colombiana; sin embargo, la falta de protocolos de propagación, evita su cultivo comercialmente. Con el interés ajustar un protocolo de micropropagación para la producción clonal de material de siembra, el presente estudio tuvo como objetivo, evaluar el efecto de la sacarosa y el ácido giberélico (GA3) en el medio de cultivo, sobre el número de nuevos brotes por explante, longitud de brotes, número de raíces, longitud de raíces y número de hojas. Los explantes fueron cultivados en medio semisólido ms (Murashige & Skoog, 1962) suplido con sacarosa (30, 45 y 60 mg/L) y combinados con GA3(0,0; 0,25; 0,5; 0,75 y 1,0 mg/L). Se empleó un arreglo factorial con dos factores, 15 tratamientos y 10 repeticiones por tratamiento, para un total de 150 unidades experimentales distribuidas en un diseño completamente al azar. Los cultivos fueron almacenados cinco semanas a 25 °C con 12 h de fotoperíodo (40 μmol m-2 s-1). El Anova y la prueba de Tukey permitieron detectar que la sacarosa y GA3 afectaron significativamente todas las variables: el incremento en la concentración de sacarosa indujo mayor número de brotes, y los brotes en presencia de GA3 tuvieron una mayor longitud. Se observó una tendencia a disminuir la longitud de las raíces y el número de hojas en la medida en que se aumentó la dosis de sacarosa y GA3.
Palabras clave inflorecencia; jengibre rojo; micropropagación; plantas ornamentales; sustancias de crecimiento vegetal
Introduction
Alpinia purpurata (Vieill) K. Schum.) or commonly known as red ginger, is an ornamental plant species that belongs to the Zingiberaceae plant family. It is native from the Asia-Pacific islands and is known worldwide for its exotic tropical flowers that have an intense red color and a long shelf life (Criley, 1989; Hansen, 1993). Plants of A. purpurata grow most frequently in humid environments with at least 1 200 mm of rain annually, with an average temperature above 20 °C, and at altitudes of up to 600 m above the sea level (De la Peña & Illsley, 2001). This species is herbaceous with multiples stems of 0.8-2.5 m long that grow from a single point (Criley, 1989; Hansen, 1993). Leaves are elliptic, narrow, with an acute apex and a cuneate base, 20-50 cm long and 3.5-15.0 cm wide, and green in color (Criley, 1989; Hansen, 1993). Flowers grow in a determined spike-like inflorescence, i.e., a cincinnus, with 1-5 flowers each, obovate, 3-5 cm long, red, obtuse or acute, with glabrous bracts and tubular bracteoles, 20-40 mm long (Criley, 1989; Hansen, 1993). Pedicels are 2 mm long, and the calyx is 10-20 mm long; the corolla is 30-50 mm long, glabrous, with a petal-like form (Criley, 1989; Hansen, 1993). The fruit is a capsule up to 30 mm in diameter, subglobose, and with red seeds when mature (Criley, 1989; Hansen, 1993; De la Peña & Illsley, 2001).
Plants of Alpinia purpurata naturally propagate through clonal and sexual methods (Hoyos, 1998). Clonal propagation occurs from buds that grow in the inflorescence; aging flowers form new shoots that emerge from the axils of the red bracts; when these flowers fall, and the emerged shoots touch the substrate, adventitious roots are formed; this allows them to grow as a new single plant (Hoyos, 1998). Alternatively, rhizome formation produces underground buds that emerge and develop in new stems becoming new plants joined at the base (Hoyos, 1998). Sexual propagation is less frequent; seeds have a wide germination gap, from three months up to three years (De la Peña & Illsley, 2001; Neal, 1965). Germination percentages are low because of dormancy. Seedlings are usually less vigorous than clonal propagated shoots and variability is present in new individuals obtained from a single plant (Hoyos, 1998; Neal, 1965).
In Colombia, particularly in the northern coast (Atlantic region), red ginger grows naturally in wet soils and rainy areas (Suárez et al., 2008). Although Colombia is a strong competitor worldwide in fresh flower trade, red ginger is not included among the flowers exported by the country, and it is neither widely commercialized locally (“Estas son las flores…”, 2018; Procolombia, 2018). Commercial red ginger plants for stem, flower or foliage production and commercialization are not common, and those that are commercialized in cities are usually extracted from natural populations in rural areas (Builes, 2003; Suárez, 2008). Tropical flowers and foliage are an important item in global flower trade, and red ginger has enormous potential because of its red intense color and long shelf life (Builes, 2003; Suárez, 2008). However, high-quality plant material to cultivate commercially for massive flower production is not available (Procolombia, 2018). With natural clonal propagation, obtaining high quantities of propagules is a slow process, and seedling quality hinders cultivation expansion (Cassells, 2012). Micropropagation is a clonal propagation method that allows massive plant material production under controlled environmental conditions, reduced space and free of microbial and pest contamination (Cardoso et al., 2018; Kane, 1996). Studies on micropropagation of A. purpurata through the culture of explants with pre-existing meristems have been reported (Kochutressia et al., 2010; Vijaykuma et al., 2015). Shoot growth and organ size are critical for plant survival and recovery when transferred to ex vitro conditions. Gibberellins have been associated with internode shoot elongation and increased in vitro shoot length, while sucrose promotes plant organ growth (Ayano et al., 2014; Camara et al., 2018; Gabryszewska, 2011; Han et al., 2011; Rizza et al., 2017). Nevertheless, no studies have been reported attempting to increase the size of micropropagated A. purpurata plants by using gibberellic acid (GA3) or sucrose. In the current study, A. purpurata plants were micropropagated and the effect of different GA3 and sucrose concentrations on the size of the plants were evaluated.
Materials and methods
Plant material and explant disinfection
Explants were obtained from buds grown on old plant inflorescences in the field of Universidad de Córdoba (Monteria, Colombia). Leaves and necrotic tissues were cut off, and sections of 2-3 cm long were washed with bacteriological soap and tap water followed by 1 h immersion in 0.2 % benzoimidazole. Thereafter, explants were immersed in a 1.25 % NaClO solution with two drops of Tween 20® in a beaker with a stir bar for continuous mixing during 15 min; then, in a laminar flow hood, explants were washed three times with sterile water, and the bleached basal end explants were removed using a sterile surgical blade.
In vitro establishment and multiplication
Explants were placed in 200 cc borosilicate flasks containing 30 mL of semisolid MS (Murashige & Skoog, 1962) medium supplied with (in mg/L) myo-inositol (100), sucrose (30 000), thiamine HCl (0.4) and Phytagel® (3000) (Sigma Co.). A single explant was placed per recipient; each recipient was covered with two layers of heavy-duty aluminium foil and sealed with Nescofilm®. Cultures were stored at 25 °C with a 12 h photoperiod (40 μmol m-2 s-1) using fluorescent lamps that provided cool white light. Cultures were transferred to a fresh medium of the same formulation every four weeks. After four subcultures were carried out (5 months under culture conditions), explants established in vitro were transferred to a medium with the same formulation but supplied with 1.0 mg/L benzylaminopurine (BAP) for shoot multiplication. Explants were subcultured and multiplied four times to proliferate enough material for treatment evaluation.
Evaluation of gibberellic acid and sucrose on shoot performance
To evaluate the effect of gibberellic acid (GA3) and sucrose on shoot growth and development, proliferated shoots were individually established in 150 mm × mm borosilicate test tubes containing 20 mL of liquid MS medium supplied as indicated for shoot establishment and multiplication stages but without Phytagel®. The experiment employed was a two-way factorial design with 15 treatments consisting of five levels of gibberellic acid (0.0; 0.25. 0.5; 0.75 and 1.0 mg/L) independently combined with three concentrations of sucrose (30, 45 and 60 g/L). Each treatment was replicated 15 times for a total of 150 experimental units distributed using a complete randomized design. Cultures were stored at 25 °C with a 12 h photoperiod (40 μmol m-2 s-1) using fluorescent lamps that provided cool white light. After five weeks, variables such as the number of shoots, shoot length, number of roots, root length, and number of leaves were recorded and analyzed per treatment.
The data obtained were analyzed with an Anova (α = 0.05) (S. A. S. version 9.2) based on the model Yij = μ + ai + bj + Єij ; where . was the general mean, i was the effect of GA3, . was the effect of sucrose, and Єij was the effect of the experimental error. Means were separated using Tukey’s test (α = 0.05).
Results and discussion
Evaluation of gibberellic acid and sucrose on shoot performance
Number of shoots
The Anova allowed detecting statistical differences (Pr ≤ 0.05) among all treatments compared to the number of new shoots formed in vitro. The data showed that explants cultured in media supplied with 45 and 60 g/L sucrose deprived of GA3 produced more shoots per explant compared to any other treatment (table 1). In contrast, the presence of GA3 in the medium negatively affected shoot formation, and a concentration of ≥ 0.5 GA3 prevented shoot formation independently of the sucrose concentration (table 1).
Shoot length
The Anova allowed detecting statistical differences (Pr≤ 0.05) among all treatments compared to the shoot length variable. The collected data showed that shoots cultured in media supplied with ≥ 0.5 mg/L GA3 produced longer shoots compared to those cultured in other culture media (table 2). Furthermore, increasing sucrose concentration to > 45 g/L produced shorter shoots independent of the GA3 concentration. Although Tukey’s test grouped several treatments, the data showed that the longest shoots were produced when these were cultured in media supplied with 0.75 and 1 mg/L GA3 combined with 45 mg/L sucrose (table 2).
Root number
The Anova allowed detecting statistical differences (Pr≤ 0.05) among all treatments concerning the variable number of adventitious roots formed. Tukey’s test indicated that shoots cultured in media supplied with 30 and 45 mg/L sucrose, in the absence of GA3, formed the highest number of adventitious roots; however, these were not different from other treatments supplied with GA3 (table 3). The general data collected and analyzed did not show a defined pattern on the effect of GA3 or sucrose and their concentrations.
Table 3 Effect of gibberellic acid (GA3) and sucrose on the number of adventitious roots developed in red ginger (Alpinia purpurata) explants cultivated in vitro
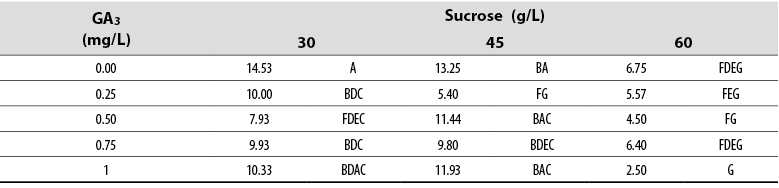
*Numbers with the same letter are not different according to Tukey’s test (α ≤ 0.05)
Source: Elaborated by the authors
The Anova applied to the rooting data allowed detecting statistical differences among all treatments (Pr ≤ 0.05). Tukey’s mean separation test showed that shoots cultured in media supplied with 30 and 45 mg/L sucrose and with no GA3, formed longer roots compared to those cultured with other GA3 and sucrose combinations. The general data indicated that roots are prone to reduce their length as GA3 and sucrose concentrations in the media increase.
Number of leaves
The Anova allowed detecting statistical differences (Pr ≤ 0.05) among all treatments with respect to the variable number of leaves per treatment. Tukey’s test showed that a supply of 30 g/L sucrose with no GA3 in the medium induced the highest number of leaves per single shoot; in contrast, the combination of 60 g/L with either 0.75 or 1.00 mg/L GA3 formed the lowest number of leaves per shoot (table 4). The general data showed that leaf number tends to decrease as GA3 and sucrose concentrations increase in the culture media (table 5).
Table 5 Effect of GA3 and sucrose on the number of leaves developed in red ginger (Alpinia purpurata) explants cultivated in vitro
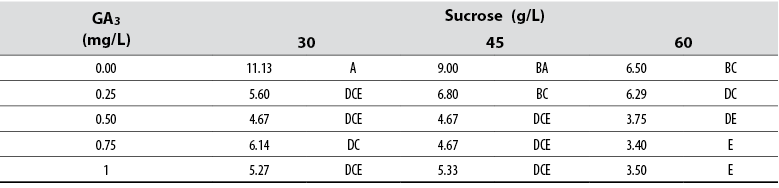
*Numbers with the same letter are not different according to Tukey’s test (α ≤ 0.05)
Source: Elaborated by the authors
Red ginger (A. purpurata) is an herbaceous plant with colorful, large, and long-lasting inflorescences that are very appreciated in the international flower market where a single stem flower costs around US$5.6 (Wholeblossom, 2018). Red ginger grows naturally in the northern coast (Atlantic region) of Colombia and is adapted to the tropical, humid and warm environments of this region; however, commercial crop production is hindered by the absence of an efficient protocol to provide massive quantities of high-quality plant material for commercial cropping (Suárez et al., 2008). Micropropagation has proved to be an efficient method for producing large amounts of propagules with superior health and genetic fidelity standards for several ornamental plant species (Cardoso et al., 2018; Kunakhonnuruk et al., 2018; Park et al., 2017).
Red ginger has been propagated through explant micropropagation with pre-existing meristems using inflorescence buds as initial explants cultured in MS medium supplied with BAP/IAA (Indoleacetic acid) or naftalenacetic acid (NAA), i.e., a combination for shoot induction and multiplication (Illg & Faria, 1995). Rhizome buds were cultured in a medium supplied with several combinations of BAP with kinetin for shoot production (Kochuthressia et al., 2010), and rhizome buds cultured in MS medium provided with different levels of BAP with NAA or 2,4 diclorofenoxiacetic acid (2,4-D) were used as explants for callus induction and indirect shoot organogenesis (Vijaykumar & Namdeo, 2015). In contrast to these studies, in the current research, instead of using cytokines or auxins for shoot induction and multiplication, gibberellins and sucrose were used to evaluate their effects on shoot growth and development.
The data showed that the treatments employed induced significant effects for all variables. Although shoot multiplication was not the main purpose in this work, statistically high sucrose concentrations (45 and 60 g/L) were observed to increase the number of shoots, being equivalent to a supply of 1.0-2.0 mg/L BAP combined with 1.5-2.0 mg/L kinetin in the culture medium for shoot multiplication according to studies as the one carried out by Kochuthressia et al. (2010).
Gibberellins are known to increase shoot length by inducing cell elongation and division (Ayano et al., 2014; Camara et al., 2018; Han et al., 2011; Rizza et al., 2017). In the current study, shoots were longer (> 12 cm) when cultured in a media supplied with higher levels of GA3 (0.75 or 1.0 mg/L) and also superior in size when compared to other studies in this same species (7.9 cm) in absence of GA3 (Kochuthressia et al., 2010). A supply of GA3 in the culture media of explants from different species has proved to increase culture size and contribute to a better performance of seedlings when transferred to ex vitro conditions, and subsequently, to the field (Dos Santos et al., 2017; Hesami et al., 2018; Kang et al., 2004). In contrast, high levels of sucrose in the culture medium decreased the osmotic potential, and therefore, nutrients and water retention forces in the medium increase; furthermore, this also increases the difficulty for cultured plants to obtain water and organic/inorganic compounds from the medium (Hassan, 2017; Hazubska-Przybył et al., 2016).
Roots are plant organs specialized in water and nutrient uptake (Augstein & Carlsbecker, 2018; Hodge et al., 2009). Plant species can be classified as easy, difficult, or recalcitrant to form adventitious roots (Hartmann et al., 2002). Usually, in vitro root formation is induced by supplying the medium with plant growth regulators (PGR) such as naphthaleneacetic acid (NAA), IAA or indole-3-butyric-acid (IBA); gibberellins are known to prevent adventitious root formation, and sucrose to reduce root size (Gao et al., 2018; Rahman & Blake, 1988), as we have also noted in this study. The data showed that sucrose and GA3 supply reduced root size as concentrations were increased (supplied) in the medium; however, no specific pattern was observed concerning adventitious root formation. Alpinia purpurata is classified as an easy-to-root species due to the in vivo formation of large quantities of adventitious roots (Suárez et al., 2008). A basal medium with the conventional supply of 30 g/L sucrose generated in the highest number of adventitious root formation (> 10 roots per explant) in red ginger; this result clearly showed that not only GA3 and high sucrose levels have a detrimental effect on adventitious root formation and root growth, but also that red ginger shoots easily form adventitious roots in the absence of root inducers (auxins) in the culture medium.
Similarly to rooting, leaf formation seemed to be negatively affected by high levels of sucrose in the culture medium; nonetheless, the effects of increases of GA3 are not apparent. As in vitro plant growth is heterotroph rather than autotroph, leaves are demanding organs rather than energy source organs. As indicated before, high sucrose levels in the medium lowered the osmotic potential resulting in fewer nutrients available for plant tissues, including energy compounds, such as carbohydrates. Decreasing leaf formation could be a response of the plant growing in vitro that has a limited nutrient supply, and therefore, reduces the number of leaves (Carloni et al., 2017; Martins et al., 2015).
Plant growth regulators are substances that, at very low amounts, affect growth and developmental processes in plants (Gaspar et al., 1996; Rademacher, 2015). Gibberellins are PGRs that promote cell elongation and division, and therefore, plant organ growth and development (Hedden et al., 2015; Kuhnle et al., 1983). When used in vitro, gibberellins are often employed to promote length in micropropagated shoots to increase plant survival when transferred to ex vitro conditions, and to improve plant performance when planted in the field (Ali et al., 2018; Brondani et al., 2012). This research showed the beneficial effects of GA3 to increase the shoot length of micropropagated A. purpurata shoots; however, organ formation, such as leaves and roots, as well as their size —equally crucial for plant survival and growth— were negatively affected by the supply of GA3. Nevertheless, further field performance experiments of micropropagated plants with or without GA3 supply in the micropropagation medium are recommended.
Conclusions
The supply of gibberellic acid (GA3) combined with sucrose statistically affected the number of shoots, shoot length, root formation, root length, and leaf formation on A. purpurata shoots cultured in vitro.
Increasing the supply of GA3 in the culture medium reduced the number of shoots per A. purpurata explant but increased shoot length. Leaf length and number in A. purpurata shoots decreased as the sucrose content increased in the culture medium. Micropropagated shoots performed better in length, increasing when GA3 levels were 1.0 mg/L independent of the sucrose level.
Acknowledgments
The authors would like to express their gratitude to the following entities for their support: Instituto de Biotecnología Aplicada del Caribe (ibac) and Universidad de Córdoba. The assistance of Carmen Polo Tordecilla is also gratefully acknowledged.
REFERENCES
Ali, S., Khan, N., Nouroz, F., Erum, S., Nasim, W., & Adnan Shadid, M. (2018). In vitro effects of GA. on morphogenesis of CIP potato explants and acclimatization of plantlets in field. In Vitro Cellular and Developmental Biology-Plant, 54(1), 104-111. https://doi.org/10.1007/s11627-017-9874-x [ Links ]
Augstein, F., & Carlsbecker, A. (2018). Getting to the roots: A developmental genetic view of root anatomy and function from Arabidopsis to lycophytes. Frontiers in Plant Science, 9(1410) 1-15. https://doi.org/10.3389/fpls.2018.01410 [ Links ]
Ayano, M., Kani, T., Kojima, M., Sakakibara, H., Kitaoka, T., Kuroha, T., Angeles-Shim, R., Kitano, H., Nagai, K., & Ashikari, M. (2014). Gibberellin biosynthesis and signal transduction is essential for internode elongation Subburaman in deepwater rice.Plant Cell and Environment, 37(10), 2313-2324. https://doi.org/10.1111/pce.12377 [ Links ]
Builes, M. (2003). Diagnóstico del mercado de los platanillos (Zingiberales) en el departamento de Antioquia [tesis de pregado]. Corporación Universitaria Lasallista. [ Links ]
Brondani, G., de Wit Ondas, H., Baccarin, F., Natal, A., & de Almeida, M. (2012). Micropropagation of Eucalyptus benthamii to form a clonal micro-garden. In Vitro Cellular and Developmental Biology-Plant, 48(5), 478-487. https://doi.org/10.1007/s11627-012-9449-9 [ Links ]
Camara, M., Vandenberghe, L., Rodríguez, C., Oliveira, J., Faulds, C., Bertrand, E., & Soccol, C. (2018). Current advances in gibberellic acid (GA.) production, patented technologies and potential applications. Planta, 248(5), 1049-1062. https://doi.org/10.1007/s00425-018-2959-x [ Links ]
Cardoso, J., Sheng, L., & Teixeira da Silva, J. (2018). Micropropagation in the twenty-first century. Methods in Molecular Biology, 1815, 7-46. https://doi.org/10.1007/978-1-4939-8594-4_2 [ Links ]
Carloni, E., Tommasino, E., López Colomba, E., Ribotta, A., Quiroga, M., & Griffa, S. (2017). In vitro selection and characterization of buffelgrass somaclones with different responses to water stress. Plant Cell, Tissue and Organ Culture, 130(2), 265-277. https://doi.org/10.1007/s11240-017-1220-9 [ Links ]
Cassells A. (2012). Pathogen and biological contamination management in plant tissue culture: phytopathogens, vitro pathogens, and vitro pests. Methods in Molecular Biology, 877, 57-80. https://doi.org/10.1007/978-1-61779-818-4_6 [ Links ]
Criley, R. (1989). Development of Heliconia and Alpinia in Hawaii: Cultivar selection and culture. Acta Horticulturae, 246, 247-258. https://doi.org/10.17660/ActaHortic.1989.246.30 [ Links ]
De la Peña, G., . Illsley, C. (2001). Los productos forestales no maderables: su potencial económico, social y de conservación. https://geaac.org/images/stories/OM_prodforestnomaderablepotenciales_190510.pdf [ Links ]
Dos Santos, D., Cardoso-Gustavson, P., & Nievola, C. (2017). Stem elongation of ornamental bromeliad in tissue culture depends on the temperature even in the presence of gibberellic acid. Acta Physiologiae Plantarum, 39, 230. https://doi.org/10.1007/s11738-017-2536-0 [ Links ]
Estas son las flores que más exporta Colombia. (2018). El Tiempo. https://www.eltiempo.com/colombia/otras-ciudades/las-flores-que-mas-exporta-colombia-223144 [ Links ]
Gabryszewska, E. (2011). Effect of various levels of sucrose, nitrogen salts and temperature on the growth and development of Syringa vulgaris L. shoots in vitro. Journal of Fruit and Ornamental Plant Research, 19(2), 133-148. [ Links ]
Gao, Y., Wu, C., Piao, X., Hang, L. Gao, R., & Lian, M. (2018). Optimization of culture medium components and culture period for production of adventitious roots of Echinacea pallida (Nutt.) Nutt. Plant Cell, Tissue and Organ Culture, 135(2), 299-307. https://doi.org/10.1007/s11240-018-1464-z [ Links ]
Gaspar, T., Kevers, C., Penel, C., Greppin, H., Reid, D., & Thorpe, T. (1996). Plant hormones and plant growth regulators in plant tissue culture. In Vitro Cellular and Developmental Biology-Plant, 32(4), 272-289. [ Links ]
Han, K., Dharmawardhana, P., Arias, R., Ma, C., Busov, V., & Strauss, S. (2011). Gibberellin-associated cisgenes modify growth, stature and wood properties in Populus. Plant Biotechnology Journal, 9(2), 162-178. https://doi.org/10.1111/j.1467-7652.2010.00537.x [ Links ]
Hansen, J. (1993). Field phenology of red ginger, Alpinia purpurata. Proceedings of the Florida State Horticultural Society, 106, 290-292. https://journals.flvc.org/fshs/article/view/92679/88871 [ Links ]
Hartmann, H., Kester, D., Davies, F., & Geneve, R. (2002). Plant Propagation: Principles and Practices. Prentice-Hall. [ Links ]
Hassan, M. (2017). In vitro conservation of date palm somatic embryos using growth-retardant conditions. En J. Al-Khayri, S. Jain & D. Johnson (Eds.), Date Palm Biotechnology Protocols Volume II. Methods in Molecular Biology (vol. 1638). Humana Press. https://doi.org/10.1007/978-1-4939-7159-6_6 [ Links ]
Hazubska-Przybył, T., Kalemba, E., Ratajczak, E., & Bojarczuk, K. (2016). Effects of abscisic acid and an osmoticum on the maturation, starch accumulation and germination of Picea spp. somatic embryos. Acta Physiologiae Plantarum, 38, 59. https://doi.org/10.1007/s11738-016-2078-x [ Links ]
Hesami, M., Daneshvar, M., & Yoosefzadeh-Najafabadi, M. (2018). An efficient in vitro shoot regeneration through direct organogenesis from seedling-derived petiole and leaf segments and acclimatization of Ficus religiosa. Journal of Forestry Research, 30(3) 807-815.https://doi.org/10.1007/s11676-018-0647-0 [ Links ]
Hedden, P., & Sponsel, V. (2015). A century of gibberellin research. Journal of Plant Growth Regulation, 34(4), 740-760. https://doi.org/10.1007/s00344-015-9546-1 [ Links ]
Hodge, A., Berta, G., Doussan, C., Merchan, F., & Crespi, M. (2009). Plant root growth, architecture and function. Plant and Soil, 321(1-2), 153-187. https://doi.org/10.1007/s11104-009-9929-9 [ Links ]
Hoyos, J. (1998). Plantas tropicales ornamentales de tallo herbáceo. Sociedad de Ciencias Naturales La Salle. http://www.tecniciencia.com/tcl-PLANTAS-TROPICALES-ORNAMENTALES-DE-TALLO-HERBACEO-9802350346.php [ Links ]
Illg, R., & Faria, R. (1995). Micropropagation of Alpinia purpurata from inflorescence buds. Plant Cell, Tissue and Organ Culture, 40(2), 183-185. https://doi.org/10.1007/BF00037673 [ Links ]
Kane, M. (1996). Micropropagation from pre-existing meristems. En R. N. Trigiano & D. J. Gray (Eds.), Plant tissue culture concepts and laboratory exercises (pp. 75-86). CRC Press. [ Links ]
Kang, Y., Min, Y., Moon, H., Karigar, C., Prasad, D. Lee, C., & Choi, M. (2004). Rapid in vitro adventitious shoot propagation of Scopolia parviflora through rhizome cultures for enhanced production of tropane alkaloids. Plant cell Reports, 23(3), 128-133. https://doi.org/10.1007/s00299-004-0820-0 [ Links ]
Kochuthressia, K. P., Britto, S. J., Raj, M., Jaseentha, M. O., & Senthilkumar, S. R. (2010). Efficient regeneration of Alpinia purpurata (Vieill.) K.Schum. plantlets from rhizome bud explants. International Research Journal of Plant Science, 1(2), 43-47. [ Links ]
Kuhnle, J., Moore, P., Haddon, W., & Fitch, M. (1983). Identification of gibberellins from sugarcane plants. Journal of Plant Growth Regulation, 2(1), 59-71. https://doi.org/10.1007/BF02042234 [ Links ]
Kunakhonnuruk, B., Inthima, P., & Kongbangkerd, A. (2018). In vitro propagation of Epipactis flava Seidenf, an endangered rheophytic orchid: a first study on factors affecting asymbiotic seed germination, seedling development and greenhouse acclimatization. Plant Cell, Tissue and Organ Culture, 135(3), 419-432. https://doi.org/10.1007/s11240-018-1475-9 [ Links ]
Martins, J., Verdoodt, V., Pasqual, M., & De Proft, M. (2015). Impacts of photoautotrophic and photomixotrophic conditions on in vitro propagated Billbergiazebrina (Bromeliaceae). Tissue and Organ Culture, 123(1), 121-132. https://doi.org/10.1007/s11240-015-0820-5 [ Links ]
Murashige, T., & Skoog, F. (1962). A revised medium for rapid growth and bioassay with tobacco tissue culture. Physiologia Plantarum, 15(3), 473-497. [ Links ]
Neal, M. (1965). Gardens of Hawaii (50.. edición). Bishop Museum Press. http://hbs.bishopmuseum.org/pubs-online/bpbm-sp.html [ Links ]
Park, H., Kim, D., & Sivanesan, I. (2017). Micropropagation of Ajuga species: a mini review. Biotechnology Letters, 39(9), 1291-1298. https://doi.org/10.1007/s10529-017-2376-4 [ Links ]
Procolombia. (2018). Flores. http://www.procolombia.co/node/1255 [ Links ]
Rahman, M., & Blake, J. (1988). The effects of medium composition and culture conditions on in vitro rooting and ex vitro establishment of jackfruit (Artocarpus heterophyllus Lam.). Plant Cell, Tissue and Organ Culture, 13(3), 189-200. https://doi.org/10.1007/BF00043668 [ Links ]
Rademacher, W. (2015). Plant growth regulators: Backgrounds and uses in plant production. Journal of Plant Growth Regulation, 34(4), 845-872. https://doi.org/10.1007/s00344-015-9541-6 [ Links ]
Rizza, A., Walia, A., Languar, V., Frommer, W., & Jones, A. (2017). In vivo gibberellin gradients visualized in rapidly elongating tissues. Nature Plants, 3(10), 803-813. https://doi.org/10.1038/s41477-017-0021-9 [ Links ]
Suárez, I., Marrugo, G., & Peña, M. (2008). Efecto del sustrato y tamaño del propágulo en el enraizamiento de ginger rojo (Alpinia purpurata). Revista Colombiana de Ciencias Hortícolas, 2(2), 225-231. https://doi.org/10.17584/rcch.2008v2i2.1190 [ Links ]
Vijaykumar M. K., & Namdeo A. G. N. (2015). Micropropogation of Alpinia purpurata using low cost media for quantification of rutin. Scholars Research Library der Pharmacia Lettre, 7(5), 50-57. [ Links ]
Whole Blossoms. (2018). Red Ginger Flower. http://www.wholeblossoms.com/tropical-flowers/ginger-flower/red-ginger-flower.html [ Links ]
Received: November 22, 2018; Accepted: November 13, 2019