Introduction
Substrates, also called growth media (Gruda, 2019), are solid materials other than soil used for agricultural production in soilless culture (SLC) systems. SLCs have been developed globally for more than eight decades (Raviv & Heinrick, 2019; Savvas & Gruda, 2018). In Colombia, they are used in carnation and minicarnation production to avoid Fusarium oxysporum f. sp. dianthi when grown in soil.
SLCs are classified as hydroponic, aquaponic, aeroponic, and substrate (cultures on chemically active materials) (El-Kazzaz & El-Kazzaz, 2017; Raviv & Heinrick, 2019; Savvas & Gruda, 2018). SLCs are used when (1) there is pressure due to hard-to-control diseases or soil pests, (2) there are limiting factors such as salinization, depletion of natural fertility, or physical deterioration of soil, (3) there is a need for greater control of the supply of water and nutrients in the root environment, (4) plants must be transported from one place to another (Cárdenas et al., 2006; Othman et al., 2019), and (5) urban horticulture practices are implemented (Nwosisi & Nandwani, 2018). Commercial nurseries also use substrates for producing propagation material.
The physical and chemical properties of the materials used as substrates vary widely. Their characterization is fundamental since it determines the substrate’s capacity to store and release water and nutrients to allow aeration and promote root development (Gruda, 2019). Therefore, the substrate must be chosen wisely and used appropriately (Mayo-Prieto et al., 2020). The choice of a substrate depends on availability, cost, chemical, physical and hydraulic properties, homogeneity, the objective of the SLC and cultivated species, weather conditions, the management experience, and the installation potential (Biauxuli & Aguilar, 2002). The main characteristics to be evaluated for selecting a substrate are decomposition rate, bulk density (Db ), total pore space, the pore space for aeration, water retention capacity (WRC), pH, electrical conductivity (EC), and cation exchange capacity (CEC) (Henao & Flórez, 2006).
Substrates can be of organic origins, such as rice husk, coconut fiber, and peat, or mineral origins, such as rock wool and perlite. Compared with organic materials, those of mineral origin are more stable, i.e., they maintain their properties for more extended periods, which is why they are widely used, mainly in Europe. In Colombia, their use has not been widespread due to their high cost and low availability. Rice husk is the most used material in commercial crops in Colombia because of its accessibility, low cost, and stability. However, using it raw, fresh from the crop, is not recommended as it may contain residues of agrochemicals, phytosanitary agents, or weed seeds. Its moisture retention capacity is also too low (Monsalve et al., 2009), making irrigation and fertigation management difficult. So, rice husk must first go through a roasting process, increasing the material’s cost. In recent years, rice husk has been used in other ways, such as concrete preparation, which threatens its future availability.
In the search for alternative materials to rice husk for individual or combined use, research has been carried out locally with other substrates such as coconut fiber, carbon slag, and zeolite, mixed in different proportions between them or with rice husk (Cárdenas et al., 2006; Chaparro et al., 2006; Farias et al., 2006; Flórez et al., 2006; Monsalve et al., 2009; Nieto & Flórez, 2006; Peña et al., 2014; Petitt, 2011).
Most information published on SLC and the use of substrates in horticulture focuses on the growth, production, and quality of the harvest of horticultural species. However, the substrates’ physical and chemical characteristics that affect plants’ development and performance is little mentioned. Knowledge of the properties of substrates should be used as a selection criterion. This paper aims to characterize the most important chemical, physical, and stability properties of some mineral and organic materials for potential use in SLCs in Colombian territory.
Materials and methods
Eleven organic (table 1) and seven mineral (table 2) substrates that may be highly available for not being demanded by other processes (such as raw materials for construction, fertilizers, concentrates) were selected. Organic substrates from the same plant species, but with different morphology and particle size, were included to observe the effect of these characteristics on the substrate’s physical properties.
Chemical properties of the selected materials were established as follows: pH with the potentiometric method; EC by conductometer reading at 25 °C in the saturated paste extract; CEC and exchangeable bases (Ca2+, Mg2+, K+, and Na+) using 1M ammonium acetate at pH 7. To determine the CEC, the exchanged NH4+ was displaced by NaCl, and then titration was performed. The bases were determined in the ammonium acetate extract by atomic absorption spectrophotometry. The concentration of the total elements was calculated by calcinating the sample at 600 °C and quantifying the elements Ca, Mg, K, Na, Cu, Fe, Mn, and Zn by atomic absorption spectrophotometry, P by colorimetric titration with molybdate and ammonium vanadate, and B by colorimetric titration with azomethine-H. The concentration of heavy metals was measured by solubilization with acid digestion and Cd, Co, and Cr titration, Pb by flame atomic absorption, and As, Hg, and Se by hydride generation atomic absorption.
Table 2 Materials of a mineral nature evaluated as substrates
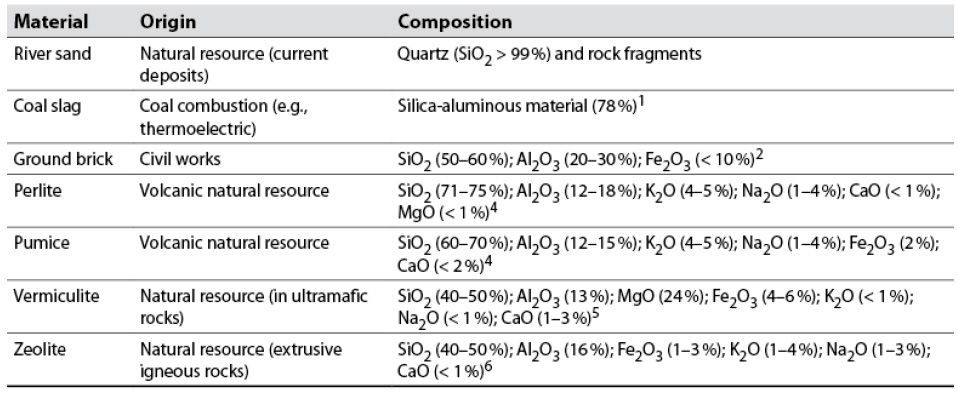
Source: Elaborated by the authors based on 1Robayo et al. (2016); 2Lourenço et al. (2010); 3Samar and Saxena (2016); 4Amato et al. (2012); 5Campos et al. (2010); 6Jha and Singh (2016).
The physical properties were determined as follows: Db by weighing a known volume of dry matter; actual density (Da) by the pycnometer method; WRC at 33, 500, and 1,500 KPa with the pressure plate method; and grain size by the dry separation of the particles using 2 and 8 mm-sieves.
The stability properties (C/N ratio, hemicellulose, cellulose, lignin, and silicon concentrations) of organic materials were determined according to the method suggested by Van Soest et al. (1991).
Results and discussion
pH
Of the substrates evaluated, those with a pH between 5.5 and 7 (table 4) have the advantage of being within the appropriate pH range, at which most of the nutrients are soluble and available to plants (Savvas & Gruda, 2018). These substrates include rice husk, dry palm leave, and coconut by-products, whose pHs close to neutrality show optimal conditions as a growth medium for plants (table 3).
All mineral materials have a slightly acidic to strongly alkaline pH, especially the pumice and perlite substrates, both of volcanic origin, due to their moderate to strongly alkaline nature (table 3). At these pHs, there may be limitations to the absorption of minor elements by the plant, mainly iron, whose solubility is extremely low at high pHs because of the formation of iron hydroxides, oxyhydroxides, and oxides (Lemanceau et al., 2009).
Mineral substrates generally have higher pHs than organic substrates, reflecting the acidic nature of organic compounds and the high contents of alkaline (Na) and alkaline earth (Ca and Mg) cations in minerals.
Table 3 Chemical properties and total nutrient concentration of the evaluated materials
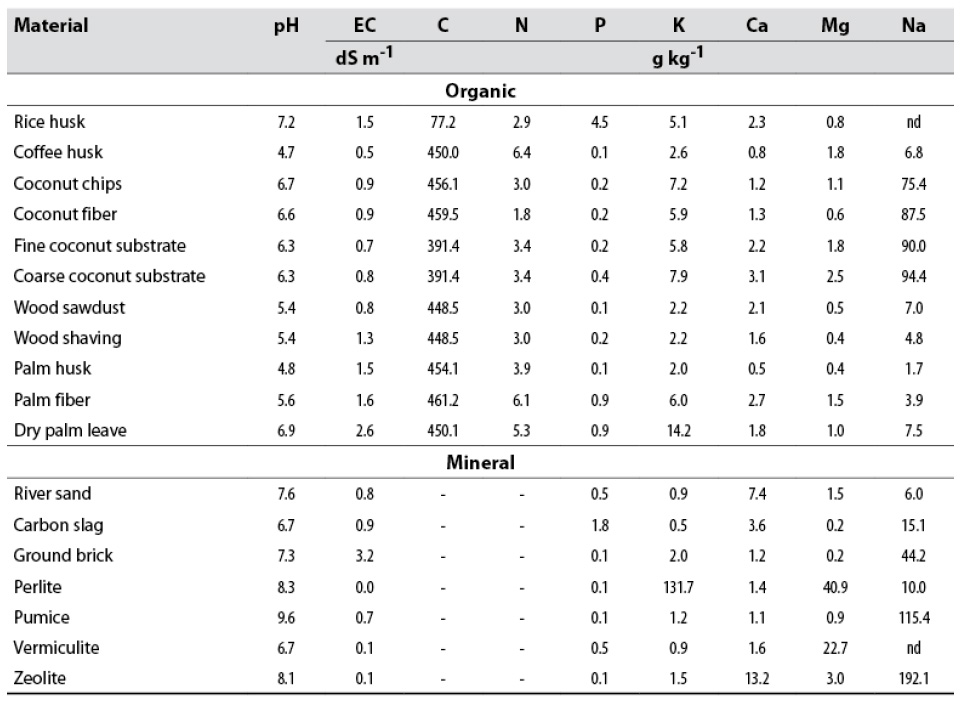
Note.nd: not determined.
Source: Elaborated by the authors
Acidic substrates are easier to handle than more alkaline ones if employed individually since the pH can be increased in the same way as in soils using lime. The pH differences in the substrates could help prepare mixtures and obtain substrates within the appropriate pH range.
Electrical conductivity
Except for dry palm leave and ground brick, EC was less than 2 dS/m in the substrates, regardless of their origin (table 3). The optimal range in substrates varies between 0.75 and 1.99 dS/m (Abad & Noguera, 2005). Above 2 dS/m, Ansorena (1994) points to the presence of soluble salts that could pose a risk for plants due to osmotic stress. The evaluated materials do not have a soluble salt concentration that limits plant growth. Ground brick is the substrate with the highest salt concentration; however, salinity problems are easier to handle in substrates than in soils, which can be solved by washing them with abundant good quality water (low EC and low sodium adsorption ratio). Alternatively, substrates with high and low EC can be mixed.
Total concentration of major and minor elements
The fundamental difference between organic and mineral substrates is the predominance of carbon and nitrogen in the former and silicon and aluminum in the latter, as all the mineral substrates evaluated are aluminosilicates. For this characterization, only C, N (table 3), and Si (table 7) were determined in the organic substrates to measure the materials’ stability over time. From the plant’s nutrient supply perspective, the total elemental composition is not a relevant chemical property of the substrate since SLC systems generally involve fertigation plans. Nonetheless, the applied nutrients’ concentration is affected by the material’s chemical composition, as N and P in organic substrates, whose availability is associated with the substrate’s mineralization rates. Moreover, the release of some elements present in the solid organic or mineral compound will entail the substrate’s decomposition and, consequently, its role as a root growth medium would be compromised.
The other elements, K, Ca, Mg, and Na are found at concentrations between 0.2 and 19 %, both in mineral and organic substrates, equal to those detected in geological, edaphic, and plant materials (tables 3 and 4). Iron is much higher in mineral substrates (0.5-24 %) than in organic substrates (< 0.4 %), according to the nature of the material. The other elements, Mn, Zn, Cu, and B, are present at trace levels (< 0.1 %) both in mineral and organic substrates. For all elements, there is high variability among substrates.
Table 4 Total micronutrient concentration of the evaluated materials
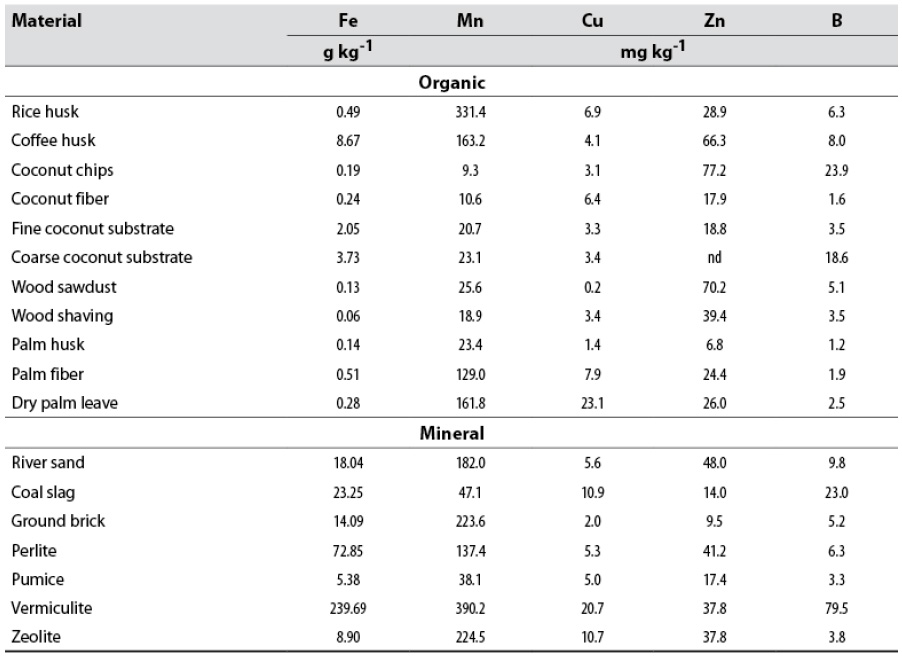
Note.nd: not determined.
Source: Elaborated by the authors
For organic substrates, C contents close to 40 % were obtained, except for rice husk with 8 % (table 3). Schmitz et al. (2002) also reported the low carbon concentration in rice husk, with 17 %. It is widely known that silicon oxides are an essential component of this material (Prada-Matiz & Cortés-Castillo, 2010) and, therefore, C is below the average for organic materials.
N levels are similar in all the characterized materials and are within the range generally reported for humidified organic matter (< 1 %) (Blume et al., 2016b), but well below the range usually reported for fresh plant tissue (1–5 %) (Hawkesford et al., 2012). Comparatively, among the eleven organic substrates, coffee husk and palm fiber exhibited the highest total N levels (0.6 %).
Although rice husk had the highest P concentration (4.5 g/kg), it is below the ideal range shown by Ansorena (1994) (4.5-10 g/kg) (table 3). Okon et al. (2005) reported phosphorus values between 0.7 and 2.2 g/kg for burned rice husk.
The total concentrations of K, Ca, Mg, and Na show the expected levels for organic materials since, added together, they correspond to less than 2 % of dry matter. Coconut-derived substrates stand out due to the high Na concentration (table 3), which may be typical of the plant ecophysiology. While not considered halophyte, it is widely cultivated along coasts subject to seawater’s influence, growing in soils with high levels of sodium chloride (Ohler, 1999).
Regarding mineral substrates, the high K concentration in perlite stands out (table 3), which is a useful attribute for this material as it has the potential to release this nutrient in forms available to plants and compete with other ions with similar physicochemical characteristics (valence and diameter), such as sodium (White, 2012).
Zeolite has a high natural Na concentration because this family of minerals, comprising natrolite (Na2Al2Si3O10·2H2O) and clinoptilolite [(NaKCa0,5Sr0,5Ba0,5Mg0,5)6(Al6Si30O72)~20H2O] used in this study, have sodium in their composition. Sodium is also predominant in pumice. Ca levels are higher in zeolite, while Mg levels are higher in perlite and vermiculite. Since the mineral substrates evaluated are poorly soluble silicates, they are not expected to release these elements quickly into the solution; however, the release of both essential and non-essential elements for plants could cause toxicity depending on the amounts released. Such could be the case of Fe and Mn in vermiculite and Na in zeolite and pumice due to their high content of these elements (table 3).
Characterizing the cation exchange properties
The CEC of organic substrates was low in rice and palm husks (close to 10 cmolc/kg) and high in coconut substrates (> 40 cmolc/kg) (figure 1). According to the optimal levels of CEC (> 20 cmolc/kg) (Abad & Noguera, 2005), except for the husks above, all organic materials have an adequate cation retention capacity. As in this study, Kroeff et al. (2004) reported a low CEC in burned rice husk (8.5 cmolc/kg), compared to Quintero et al. (2011), who found values between 25.5 and 32.5 cmolc/kg. The low CEC of rice husk coincides with its low concentration of total carbon (figure 1). The latter is an indirect measure of functional groups in organic matter, which are the reactive parts of organic molecules participating in the CEC (Blume et al., 2016b).
In contrast to organic substrates, geological origin materials have very low CEC (< 10 cmolc/kg), except for vermiculite and zeolite (27 and 23 cmolc/kg, respectively). These two minerals are crystalline silicates with negative surface charges by isomorphic substitution independent of the medium pH (Sposito, 2008). River sand is considered an almost inert substrate with low CEC because it is mainly composed of quartz (99 % SiO2), a mineral with minimal isomorphic substitution, and a small specific surface (0.15 cm2/g) (Molina, 2014).
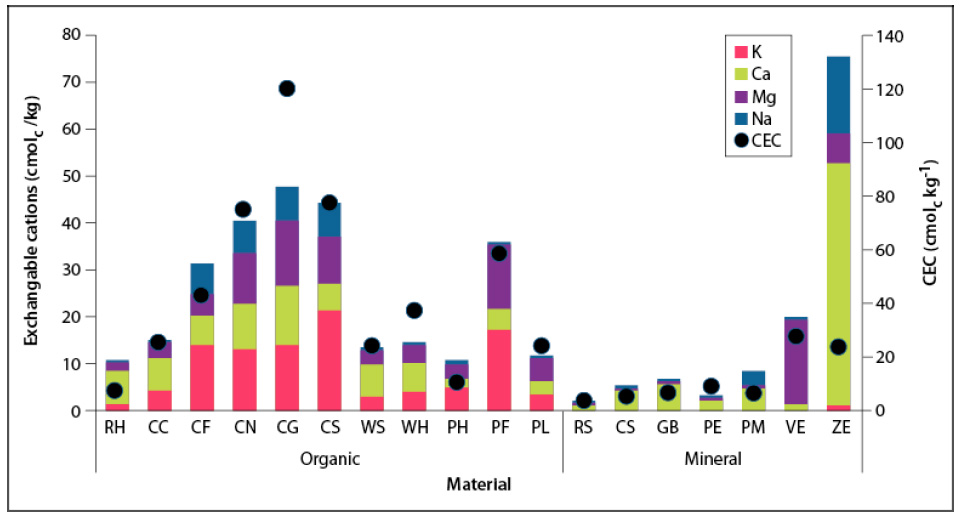
Source: Elaborated by the authors
Figure 1 Characterization of the cationic exchange complex of the evaluated materialsCharacterization of the cationic exchange complex of the evaluated materials. RH = rice husk, CC = coffee husk, CF = coconut fiber, CN = fine coconut substrate, CG = coarse coconut substrate, CS = coconut chips, WS = wood sawdust, WH = wood shavings, PH = palm husk, PF = palm fiber, PL = dry palm leave, RS = river sand, CS = coal slag, GB = ground brick, PE = perlite, PM = pumice, VE = vermiculite, ZE = zeolite.
Materials that have a high CEC create right cation adsorption conditions, which is convenient for the integrated management of irrigation and fertilization since nutrients such as K, Ca, Mg, and NH. can remain retained in the exchange complex, then be released into the solution in the growth medium, and thus be absorbed by plants (Silber, 2019). Due to low CEC levels and a decrease in the medium’s pH, elements such as potassium and magnesium can be in the water around, but not retained by, the substrate particles, favoring the leaching of these nutrients and hindering absorption by plants (Silber, 2019).
The surfaces of substrate particles can carry permanent or variable charges (Silber, 2019). Variable charges are related to the deprotonation of functional groups of molecules, such as cellulose, hemicellulose, and lignin, in response to increased pH, causing negative charges. Variable charges can be estimated as the difference between the CEC determined with 1M ammonium acetate at pH 7 and the effective cation exchange capacity (ECEC) estimated as the sum of exchangeable cations. This difference is huge for most organic materials evaluated (except rice and palm husks), with a variation range between 10 and 35 cmolc/kg. These values indicate the number of negative charges derived from increased pH in the substrate.
The ECEC is considered a CEC measure at the material’s pH (Blume et al., 2016a). The difference between both measures (CEC and ECEC) is related to the deprotonation of functional groups in response to an increase in the material’s pH when in contact with ammonium acetate at pH 7, creating variable charges depending on the pH (Sposito, 2008). The variable load of mineral substrates is low (< 5 cmolc/kg) since, for lamellar aluminosilicates such as vermiculite, over 80 % comes from isomorphic substitution (Prakash, 2002). OH- group deprotonation in the clays’ edges can be very low due to the proximity between these substrates’ pH (> 7) and that of ammonium acetate used for determination.
Zeolite shows a much higher ECEC than the CEC obtained with ammonium acetate, reflecting the solubilization of the mineral’s Ca and Na, both with high total contents (table 3), and resulting in an overestimation of both cations’ interchangeable levels (figure 1) and, therefore, the ECEC. Schollenberger and Simon (1945) reported the high solubility of calcium and sodium in ammonium acetate.
Interchangeable cations
Figure 1 shows that in organic substrates, the levels of exchangeable cations that fulfill the role of nutrients for plants are considered high to very high for potassium (1.4-17.5 cmolc/kg) and medium to high for calcium (1.8-12.4 cmolc/kg) and magnesium (1.9-13.8 cmolc/kg).
The opposite occurs in mineral substrates, especially the low levels of K and Mg. The exception is Ca in zeolite, as mentioned, and Mg in vermiculite. Vermiculite is a trioctahedral 2:1 clay with high magnesium levels within sheets and magnesium and potassium between the layers that make up the mineral structure (Prakash, 2002). The high concentration of exchangeable Mg has been documented by Peterson et al. (1965), who attributed it to the higher selective adsorption of the Mg ion over other ions such as Ca.
The concentration of exchangeable cations in organic and mineral substrates (figure 1), when regarded as available contents for plants, must be considered in fertigation programs for making rational formulations of nutritive salts.
The levels of sodium, which in high quantities can be harmful to the SLC system (Sonneveld & Van der Burg, 1991), are low in the substrates evaluated. The exception in organic materials are the by-products of coconut growing; Jeganathan (2008) had already reported the high concentration of exchangeable Na. These substrates also represent the highest concentrations of exchangeable K, Ca, and Mg (figure 1). In mineral materials, the exception was zeolite, whose high concentration of exchangeable Na (figure 1) agrees with the total Na content (19.2 %) (table 3). The high total sodium content in pumice is reflected in this element’s relatively high cation exchange complex levels, creating a potassium imbalance, with a Na/K ratio equal to 6.
Heavy metal concentration
The evaluated substrates’ total heavy metal contents showed a narrow range of variation for cadmium and selenium and a wide one for arsenic, chromium, and mercury (table 5). The maximum heavy metal concentration in the substrate considered safe can be equal to that established for soil. Typically, all organic materials have heavy metal concentrations below the maximum allowable concentration, according to the UK Department for Environment Affairs standard (Nicholson et al., 2003) (table 5), to be considered safe. Only vermiculite has a chromium concentration well above the maximum allowable for soil (400 mg/kg) according to the standard above (table 5). Presumably, those materials with high concentrations of a particular heavy metal have a tremendous potential to release it in labile forms once in contact with water and the exudates present in the rhizosphere. Then, the high concentration of chromium must be taken into consideration when choosing this substrate.
Table 5 Total heavy metal concentration of the evaluated materials
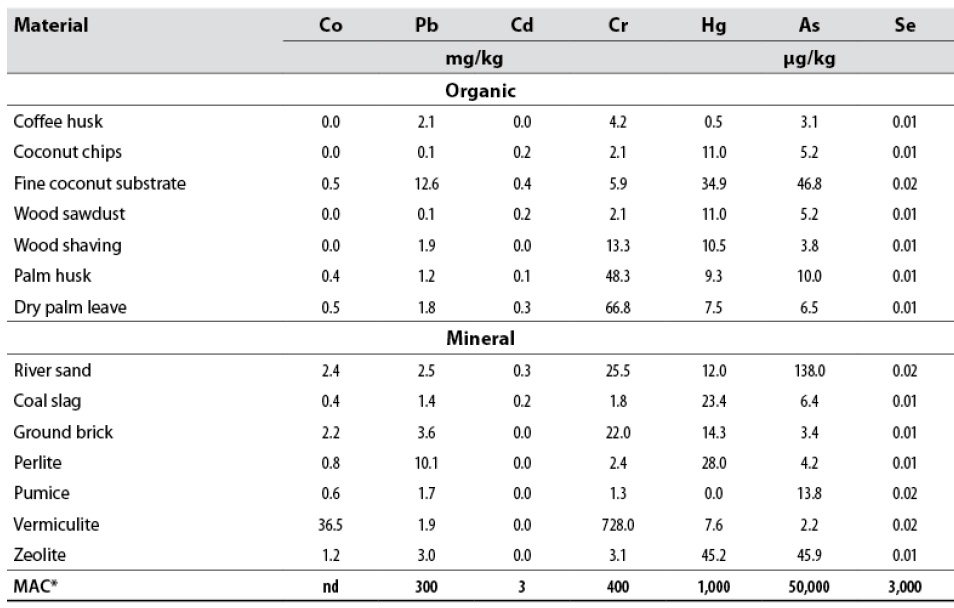
*Maximum allowable concentration of potentially toxic elements in soils after applying sewage sludge (Nicholson et al., 2003). nd: no data
Source: Elaborated by the authors
The correlations between the most relevant chemical properties of the substrates and the levels of heavy metals were not significant (data not shown), so the substrate’s nature or the type of material cannot be related to its potential toxicity. The positive relationship between total Fe and the cobalt (r = 0.96) and chromium (r = 0.94) contents is accentuated by the high levels of both heavy metals in vermiculite, which is the richest substrate in iron, just like total boron (r = 0.89).
Physical properties
According to the results presented in table 6, all organic substrates are light materials with high total porosity since their Db is within the optimal range of the ideal substrate (0.4-0.6 g/cm3), established by Ansorena (1994). River sand, coal slag, ground brick, and zeolite are above the optimal Db range. As mentioned by Quintero et al. (2012), Da is crucial for designing the container, as this property will determine the mechanical resistance conditions of the cultivation bed structures. The use of materials with higher Db requires more resistant supports to establish the cultivation beds, mostly ground brick and zeolite, with Da equal to 1.0 and 0.8 g/cm3, respectively (table 6). Besides, the transport and handling of these materials require more effort by cultivation operators.
The lowest porosity (equal to or less than 71 %) occurred in mineral origin substrates, although perlite, vermiculite, and pumice had a porosity greater than 80 %. All organic materials had total porosity > 90 %, except for dry palm leave (82 %), suggesting good root development and adequate conditions for water and oxygen supply.
Table 6 Physical analysis of the evaluated materials
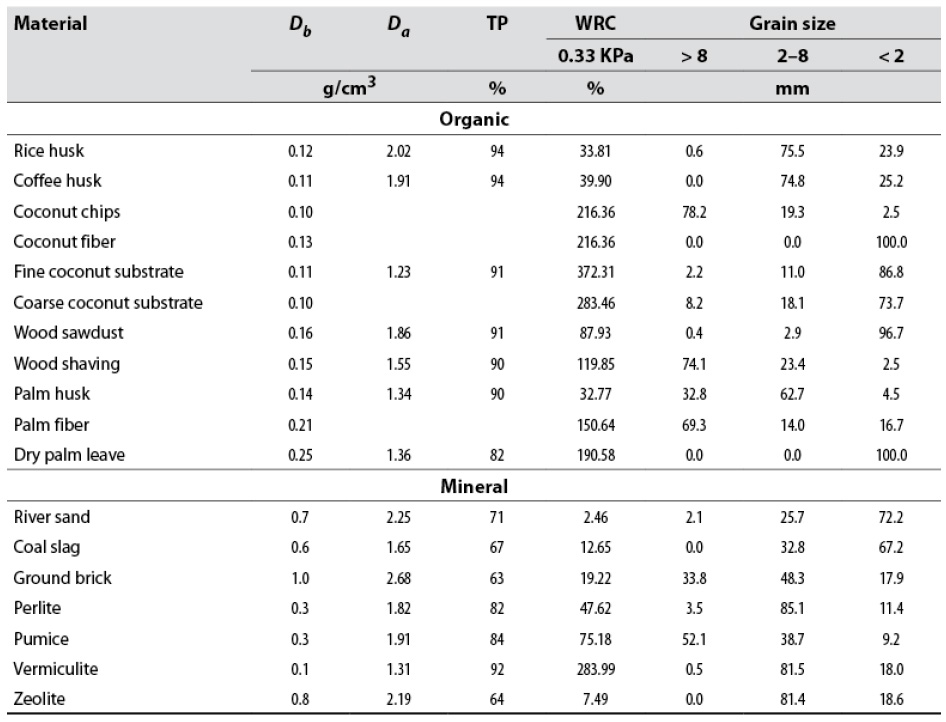
Note.Db = bulk density; Da = actual density; TP = total porosity, and WRC = water retention capacity.
Source: Elaborated by the authors
Except for rice, palm, coffee husks, and wood sawdust, organic materials have a WRC greater than 100 % at 33 KPa (table 6), indicating an optimal WRC. Regarding mineral materials, only vermiculite has a WRC greater than 100 % at 33 KPa (table 6). Vermiculites are expansive clays (Velde & Meunier, 2008) that can hydrate, which gives them a high WRC (Prakash, 2002). In contrast, river sand and zeolite showed the lowest WRC values, with 2.5 and 7.5 % at 33 KPa (table 6). Rice, palm, and coffee husks have a small WRC (< 40 % at 33 KPa); therefore, their physical properties must be improved through mixtures with materials with a high WRC.
Regarding grain size measurement, except for palm fiber, wood shaving, and coconut chips, organic materials have particle sizes less than 8 mm. Except for pumice, all mineral materials contain more than 65 % particles smaller than 8 mm. According to Ansorena (1994), the substrates must have a grain size of 0.25-2.5 mm to be used in the field. For thicker materials, grain size can be adjusted utilizing grinding processes, and even some, such as pumice, pearlite, and zeolite, are supplied with the desired grain size.
Stability properties of organic materials
Consideration should be given to substrates’ durability since tamper-resistant materials can be reused, reducing long-term production costs. Depending on the production system, be it commercial cultivation, urban agriculture, or nursery, stability may become relevant. In seedling processes, cycles do not take more than 30 days, while in crops such as carnation, they last two years, during which the substrate must not lose its properties.
The carbon-nitrogen (C/N) ratio is an indicator of the origin, maturity, and stability of the organic material (Abad et al., 2002). Except for rice husk (26.9), all materials have a C/N ratio greater than 40, mainly due to their high C content and low N content (table 7). Although this high C/N ratio may cause soluble N immobilization (Abad et al., 2002), N deficit can be supplied by fertilization (Noguera et al., 2000). In all organic materials, C is found primarily as cellulose and lignin and, to a lesser extent, as hemicellulose (table 7). This condition can make materials more resistant to microbial degradation (Abad et al., 2002) since, as stated by Blume et al. (2016b), lignin is a highly degradation-resistant polysaccharide.
The concentration of silicon oxide (SiO2) in rice husk is above 25 % (table 7), which agrees with the findings by Johar et al. (2012) and Yalçin and Sevinç (2001).
Silicon has been associated with lignin-carbohydrate complexes in the rice epidermis cell wall, which protects and mechanically strengthens the plant tissue (Feng, 2015). The Si content provides rice husk with excellent resistance to degradation (Quintero et al., 2006).
Conclusions
The substrates evaluated show a wide range of variation in their physical and chemical properties; so, the best characteristics of each can be used to make mixtures and obtain a suitable substrate for establishing SLC systems. For example, the use of coconut-derived materials will improve WRC, while the inclusion of vermiculite will increase CEC and, therefore, ion retention in the mixtures.
The substrates’ chemical composition must be considered to formulate fertigation solutions, reducing the number of nutrients in high contents. Coconut-derived substrates will not require very high levels of K in fertigation solutions. Also, the use of vermiculite would reduce magnesium levels compared to those customarily used in formulations.