Introduction
Phosphorus (P) is an essential nutrient for plants. It is part of molecules such as DNA, ATP, and RNA and is involved in protein and fatty acid biosynthesis, photosynthesis, glycolysis, and respiration, as well as cell division and multiplication (Corrales-Ramírez et al., 2014; Krishnaraj & Dahale, 2014; Wyngaard et al., 2016). In soils of the humid tropics, the mobility of this element is deficient; only 4% is available as orthophosphates, finding monobasic (H2PO4-1) and dibasic (HPO4-2) ions required for plant growth (Zhu et al., 2018). Moreover, it is possible to detect calcium phosphates such as fluorapatite, oxyapatite, and hydroxyapatite that, due to precipitation or fixation processes, are difficult to access for plant species, as well as hydrated iron or aluminum oxides that form insoluble complexes with P, not available in the soil (Cerón & Aristizábal, 2012; Daneshgar et al., 2018). Low P availability affects plant growth and agricultural productivity (Lynch, 2007; Satyaprakash et al., 2017), requiring its incorporation through chemically synthesized fertilizers in each growing season.
In Colombia, the soils of high plains are mostly of the Oxisol order (Tropeptic haplustox, isohyperthermic) (Soil Survey Staff, 1999, 2014). They are characterized by low nutrient contents with reduced percentages of organic matter (15,000–30,000 mg/kg), high iron and aluminum contents, and acidic pH between 3.8 and 5.0 (Rivas et al., 2004), meaning that P is not very available to plants and, despite being present at concentrations between 200 and 430 mg/kg, is mostly fixed by acidic conditions.
Given this condition, plants have developed adaptive strategies at the root level, such as morphological modifications with lateral root dispersion, the production of radical exudates, or the interaction with phosphate solubilizing bacteria (PSB), which favor the bioavailability of this element in the soil (Mehta et al., 2015; Richardson et al., 2011). The interaction between PSB and roots plays a crucial role in the mobility and cycling of P in many agroecosystems, particularly the tropics (Gumiere et al., 2019; Liang et al., 2020).
Bacteria of the Achromobacter, Aerobacter, Arthrobacter, Azospirillum, Azotobacter, Bacillus, Burkholderia, Erwinia, Escherichia, Flavobacterium, Gluconacetobacter, Micrococcus, Pantoea, Pseudomonas, and Rhizobium genera have been reported for their ability to solubilize phosphates that, due to fixation processes, are not available in acid soils. Some of them are associated with the rhizosphere of crops such as corn and rice (Corrales- Ramírez et al., 2014; Sandanakirouchenane et al., 2017). The solubilization mechanisms used by these microorganisms include the decrease in soil pH mediated by the production of low molecular weight organic acids such as acetic, citric, butyric, fumaric, gluconic, lactic, malic, oxalic, and succinic acids (Mehta et al., 2015; Stephen & Jisha, 2011). Besides, the production of acid phosphatases, alkaline phosphatases, lipases, and phytases also contributes to phosphate availability in the soil through organic P mineralization (Billah et al., 2019; Othman & Panhwar, 2014).
Considering that various genera of phosphate solubilizing microorganisms naturally adapted to local environments can be found in the rhizosphere, and given the low availability of this element for plants, this research aims to isolate, characterize, and identify Oxisol phosphate solubilizing rhizosphere bacteria from the Colombian high plains and evaluate their potential to promote plant growth under greenhouse conditions.
Materials and methods
Sampling and isolating phosphate solubilizing microorganisms
We collected ten composite samples, each consisting of four previously homogenized subsamples, from the rhizosphere of corn (Zea mays), soybean (Glycine max), rice (Oryza sativa), and Brachiaria sp. grass present in the savanna, whose soil was improved with the application of crop amendments, fertilizers, and rotation at different points of the Colombian high plains, in the Puerto López and Puerto Gaitán transect (04°09'42.231", -72°49'39.669"; 04°20'38.9754", -72°01'9.156") in the Meta Department. For isolating rhizosphere bacteria associated with each plant species, we selected two batches established with corn, two with rice, two with soybeans, and one with grass.
In composite samples, we determined the pH, the amount of P available by Bray II (Bray & Kurtz, 1945), and the percentage of organic matter using the Walkley and Black’s (1934) method with modifications. For counting colony-forming units (CFU) and isolating PSB, we weighed 10 g of rhizosphere with root fragments of the composite samples from each sampling point and put it in 90 mL of SMRS liquid culture medium with modifications: glucose, 10 g/L; Ca3(PO4)2, 5 g/L; (NH4)2SO4, 0.5 g/L; NaCl, 0.2 g/L; MgSO47H2O, 0.3 g/L; KCL, 0.2 g/L; MnSO4H2O, 0.004 g/L; FeSO47H2O, 0.002 g/L; pH: 7.0 ± 0.2 (Sundara & Sinha, 1963, as cited in Suliasih & Widawati, 2005). It was constantly shaken for 24 h at 200 rpm in a 3520 Orbit Shaker (Lab-Line Instruments Inc, USA).
From each sample, we made serial dilutions up to 10-6 in sterile saline at 0.85% (w/v). A 100 µL aliquot of each dilution was seeded in Pikovskaya (PKV) culture medium (Pikovskaya, 1948): glucose, 10 g/L; Ca3(PO4)2, 5 g/L; (NH4)2SO4, 0.5 g/L; NaCl, 0.2 g/L; MgSO47H2O, 0.1 g/L; KCL, 0.2 g/L; yeast extract, 0.5 g/L; MnSO4H2O, 0.002 g/L; FeSO47H2O, 0.002 g/L; agar-agar, 15 g/L, pH: 7.0 ± 0.2 and SMRS culture medium, supplemented with phosphorite (PR) (5 g/L) and added with bromocresol purple as a pH indicator. The Petri dishes with the media above were incubated for five days at 28 ± 2 °C until we observed the growth of colonies with haloes that acidified the medium, as noted by a color change. The selected strains were characterized microscopically and preserved in 20% glycerol at -20 °C until their subsequent evaluation and molecular identification.
Characterizing isolates as potential phosphate solubilizers
Determining the solubilization index (SI)
The 42 isolated strains were seeded in a Petri dish with SMRS supplemented with PR (5 g/L) and SMRS with tricalcium phosphate (TP) (5 g/L) as the only source of P. We placed three sterilized plastic rings (diameter: 0.5 cm) in each culture medium and inoculated 30 µL of a cell suspension (concentration: 108 CFU/mL) of each strain. They were incubated for 24 to 48 h at 28 ± 2 °C until we observed the formation of halos around the ring (Chakraborty et al., 2010). We measured the colony and hydrolysis halo diameters in the culture medium using a decimeter. We established three biological replicates in triplicate over time and, with the measurements obtained, determined the SI as a criterion for selecting promising strains, following the equation proposed by Awais et al. (2017):
The data were statistically analyzed using SAS, version 9.3 (SAS Institute, USA), through ANOVA with Tukey’s test (α = 0.05) to determine statistically significant differences between the means of the treatments evaluated (phosphorus source vs. strains).
Determining acid phosphatase activity
Tabatabai and Bremner’s (1969) method was used with p-nitrophenylphosphate (PNPP 0.025 M) as a colorimetric substrate. Additionally, we evaluated the evolution of the pH in the culture medium as an indicator of organic acid production. The bacterial strains were cultured in PVK (Pikovskaya, 1948) and constantly shaken at 180 rpm for nine days at 28 ± 0.5 °C (Heidolph 1000 Incubator, Heidolph Co., Germany). After the third, sixth, and ninth day of incubation, we took 5 mL aliquots of culture medium and determined the pH (Hanna Hi8314, Hanna Instruments, Germany). We also obtained samples of supernatant without bacterial cells (control sample) by centrifugation of 2 mL of culture at 24,400 xg for 10 min at 20 ± 0.5 °C (Hettich Rotina 420 Centrifuge 4701-01, Hettich GmbH & Co., Germany).
Then, we added 2 mL of 0.5 M modified universal buffer (MUB, pH 6.5) and 0.5 mL of the substrate (PNPP 0.025 M) to 1 mL of PVK supernatant medium. The reactions were carried out at 37 ± 1 °C for 60 min and stopped by adding 1 mL of 0.5 M CaCl2 and 4 mL of 0.5 M NaOH. The samples were filtered using filter paper (qualitative grade 401, VWR, Cat 5160282) with 12-15 µm pore size. The PNP formed was measured in a spectrophotometer (Optizen POP UV-Vis, Mecasys, South Korea) at 400 nm. We evaluated three independent replicas for each strain and analyzed the controls under the same experimental conditions. Enzyme activity (U) was defined as micrograms of PNP released per milliliter per hour (µg/mL/h) (Barrera et al., 2019).
Determining the phosphate solubilization percentage
The phosphomolybdenum method was used through Spectro Quant® Phosphorus (Merck KGaA, Darmstadt, Germany) to determine the solubilization percentage (Murphy & Riley, 1962, as cited in Ortiz et al., 2016). From each strain seeded in SMRS, we took 10 mL of the medium at a concentration of 108 and diluted it in 90 mL of saline solution at 0.85% (w/v). Subsequently, 2 mL of each dilution was inoculated in triplicate in 100 mL of the liquid culture medium NBRIP (National Botanical Research Institute Phosphate): glucose, 10 g/L; Ca3(PO4)2, 5 g/L; MgCl26H2O, 5 g/L; (NH4)2SO4, 0.1 g/L; NaCl, 0.2 g/L; MgSO47H2O, 0.25 g/L; KCL, 0.2 g/L; pH: 7.0 ± 0.2, described by Nautiyal (1999), supplemented with TP (5 g/L).
We used an inoculum of Penicillium janthinellum (strain: FP010 - Collection of the Bio-inputs Laboratory, Biotechnology Institute of the Universidad Nacional de Colombia [IBUN]) as a positive control, a phosphate solubilizing fungus as the active ingredient of a bioproduct for rice crops (Pineda, 2014), and the culture medium without inoculation as a negative control in triplicate. They were incubated under constant shaking at 150 rpm for ten days at 28 ± 2 °C. After the incubation time, we homogenized the fermentation broth and took 2 mL of sample centrifuged at 11,200 xg for 10 min at 20 ± 0.5 °C until the soluble fraction was wholly separated. This translucent supernatant was refrigerated at 4 ± 2 °C until reading the samples.
For determining the quantitative test, we dispensed 250 µL of the supernatant (previously obtained) into an Eppendorf tube and added 750 µL of distilled water and 240 µL of the Spectro Quant® Phosphorus reagent (Merck, Germany). Samples were vortexed (Genie®2 G560, Scientific Industries Inc., USA) and allowed to stand for 10 min; the absorbance was determined in a spectrophotometer (Optizen POP UV- Vis, Mecasys, South Korea) at 450 nm. We determined the amount of phosphate solubilized by the microorganisms, expressed in mg/L, and the percentage of solubilization with the following equations:
We determined the solubilization percentage upon obtaining the pure colonies of the isolated microorganisms (time 0) and evaluated this activity six months after storing biological material at -20 °C (time 1).
Determining indole compounds
We used the colorimetric method based on the Salkowsky reagent reaction, described by Glickmann and Deessaux (1995), with modifications. The strains were cultured in NBRIP supplemented with tryptophan (2 mg/L); we observed the color change in the culture medium under in vitro conditions after 30 min and took the absorbance reading at 450 nm in the spectrophotometer (Optizen POP UV-Vis, Mecasys, South Korea). The determinations were made in triplicate for each strain tested.
Molecular identification
The strains’ DNA was obtained with an extraction kit (GenElute™ Bacterial Genomic DNA Kits, Sigma Aldrich Co. LLC). Purity and quantification were determined in a NanoDrop® spectrophotometer (ND- 1000 UV-Vis Thermo, Fisher Scientific, Germany). With the DNA extracted from strains M15 and M18, we performed the PCR in a thermal cycler (PTC100 MJ Research®, Global Medical Instrumentation, Inc., Minnesota, USA) at a concentration of 25 µg, using the universal primers 27F (5’ AGAGTTTGATCCTGGCTCAG 3’) and 1492R (5’ AGAAAGGAGGTGATCCAG CC 3’) for amplifying the 16S rRNA gene. The amplified product was made visible on a 2% agarose gel before sequencing and sent to Macrogen Inc. (Seoul, Korea). The sequences obtained were edited and assembled using Geneious®, version 9.8 (Biomatters Ltda, Auckland, New Zealand). Subsequently, we analyzed them with the BLAST algorithm (http://blast.ncbi.nlm.nih.gov/) and compared the taxonomic analysis of the assembled sequences with the databases of the RefSeq Genomic GenBank (National Center for Biotechnology Information [NCBI]), Grengenes (Laurence Berkeley National Laboratory, http://greengenes.lbl.gov/cgi-bin/nph-index.cgi) and RDP, version 11 (Ribosomal Database Project, http://rdp.cme.msu.edu).
Virulence tests of bacterial strains
The virulence tests of the identified bacterial strains were carried out on King B agar and plant tissue of onion Allium cepa L. (Amaryllidaceae), used as a model plant (Leitão et al., 2010; Sinha et al., 2017), adapting Karki’s (2010) method. The strains were seeded in King B culture medium: meat peptone, 10 g/L; casein peptone, 10 g/L; K2HPO4, 1.5 g/L; MgSO47H2O, 1.5 g/L; agar-agar, 15 g/L, pH: 7.0 ± 0.2 (King et al., 1954), to determine the production of diffusible pigment in the medium related to phytotoxin production (Sinha et al., 2017). Then, we cut onion tissue sections of approximately 2 × 3 cm, previously disinfected with 1% sodium hypochlorite, and inoculated them with 10 µL of each strain at concentrations of 103, 105, and 108 CFU/mL. They were incubated at 30 ± 1 °C with monitoring from 24 h to 72 h. The test was considered positive when there were symptoms of tissue maceration and color changes, from pale yellow to brown, at the edge of the lesion, indicating the presence of degrading enzymes.
As a positive control, we used three strains from the Agrosavia La Libertad Research Center’s working collection, molecularly characterized as Burkholderia glumae (Kurita & Tabei, 1967), isolated from symptomatic panicles of a rice crop established in the high plains. Strain B02 was used as a negative control, molecularly identified with 99% similarity as a Rhizobium sp. (GenBank accession number MK559029) (Amaya et al., 2020), belonging to the Agrosavia Germplasm Bank.
Evaluating growth promotion in rice plants under greenhouse conditions
For evaluating the effect of phosphate solubilizing activity in the selected strains and its relationship with plant growth promotion, we conducted a pot trial with 3 kg of unsterilized soil (Oxisol) from a lot with no previous processing, low P content (2.52 mg/kg), pH 4.9, and 6,800 mg/kg of organic matter. Two Oryza sativa L. (Poaceae) rice seeds were sown in each pot, previously disinfected with 2% sodium hypochlorite for 3 min and rinsed with sterile distilled water. The seeds before sowing were soaked with an inoculum of M15 and M18 individually and co-inoculated at a cell concentration of 108 CFU/mL for 30 min. Fifteen days after the material germinated, we re-inoculated the rhizosphere of each plant by applying 2 mL of PVK with each strain at a concentration of 108 CFU/mL using a sterile syringe. We inoculated control plants with a sterile culture medium.
We evaluated nine treatments distributed in complete random blocks with six repetitions (T1: Control plants not inoculated and without P fertilization; T2: Plants inoculated with M15 without P; T3: Plants inoculated with M18 without P; T4: Plants co-inoculated with M15 and M18 without P; T5: Plants fertilized with 50% P (60 kg/ha); T6: Plants inoculated with M15 plus 50% P; T7: Plants inoculated with M18 plus 50% P; T8: Plants co-inoculated with M15 and M18 plus 50% P; T9: Plants fertilized with 100% P). M15 and M18 were previously evaluated in compatibility tests. Fertilization with P2O5 was carried out at the time of sowing using triple superphosphate, and the amount of fertilizer was defined according to the crop requirements, being 100% the recommended dose (120 kg/ha) for rice established in acid soils of the high plains (Aristizábal et al., 2000).
Destructive sampling was carried out at the crop flowering stage (BBCH 69 scale) (Enz et al., 1998), taking variables associated with growth such as dry leaf and root biomass and number and weight of panicles. The data were statistically analyzed with SAS, version 9.3 (SAS Institute, USA), through ANOVA with Tukey’s test (α = 0.05) to determine statistically significant differences between the treatments.
Results
Isolating phosphate solubilizing microorganisms
The Oxisols had pH ranges between 4.40 and 5.90, with an available P content of less than 3.51 mg/kg and organic matter between 13,600 and 21,000 mg/kg. Moreover, when counting the CFU of PSB in agricultural soils and the savanna, we obtained a count of 4.3 × 106 CFU/g of soil in the rhizosphere of soybean, followed by corn, rice, and Brachiaria sp. grass, with values of 4.0, 2.6 and 1.2 × 106 CFU/g of soil, respectively. We got 42 isolates microscopically described as Gram-negative bacteria.
Determining the SI
Of the 42 bacterial strains isolated from high plains soils, we selected 14 for having SIs between 1.2 and 2.4, solubilizing the PR and TP in SMRS. Three strains were chosen from the soybean rhizosphere, four from the corn rhizosphere, five from the rice rhizosphere, and two from the Brachiaria sp. grass rhizosphere present in the savanna.
The highest values for the SI were observed in strains M1, M15, M20, M22, M27, M3, M8, M13, M7, showing statistically significant differences in relation to M18, M23, M25, M26, and M28 (F13, 41 = 9.68; p < 0.001; Tukey’s α = 0.05) (table 1). When the strains were inoculated into SMRS with TP, we observed that M23 and M27 did not form a solubilization halo after 24 h of incubation, compared to the other strains that solubilized this source of P in the medium.
Table 1. Solubilization indices (SI) determined in the selected strains in SMRS supplemented with phosphorite (PR) and tricalcium phosphate (TP)
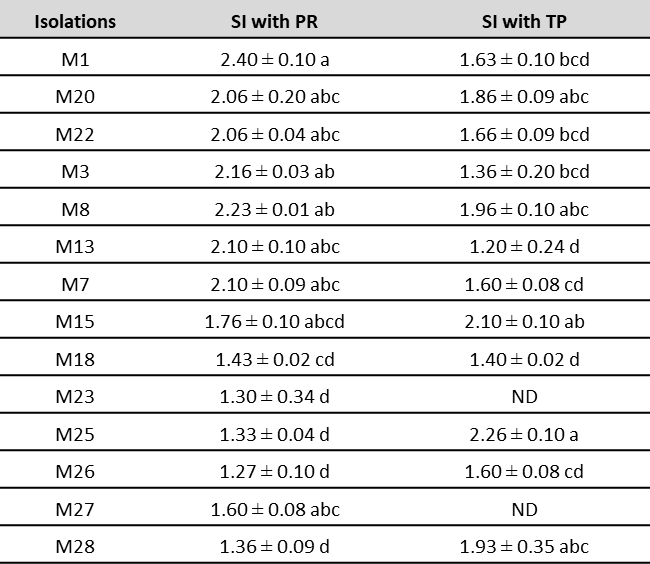
Note.Means with different letters indicate statistically significant differences according to Tukey’s test (α = 0.05); ND: not determined; ± standard error
Source: Elaborated by the authors
Determining acid phosphatase activity
A significant effect related to the sampling day (F2, 9 = 38; p < 0.001) and strain identity (F2, 98 = 46.95; p < 0.001) was found. On the third and sixth day of incubation, M15, M18, and M23 showed the highest acid phosphatase activity with values of 297.7 ± 89.6, 638.3 ± 31.2, and 666.7 ± 26.9 µg of PNPP/mL/h, respectively (Chi2= 31.20; df = 10; p = 0.00054), observing statistically significant differences compared with the other strains studied (Chi2= 30.36; df = 10; p < 0.001). At nine days of evaluation, the three strains decreased their ability to produce acid phosphatases (Chi2 = 31.09; df = 10; p = 0.00056). M1, M7, M25, and M26 did not exhibit acid phosphatases activity in the culture medium (figure 1).
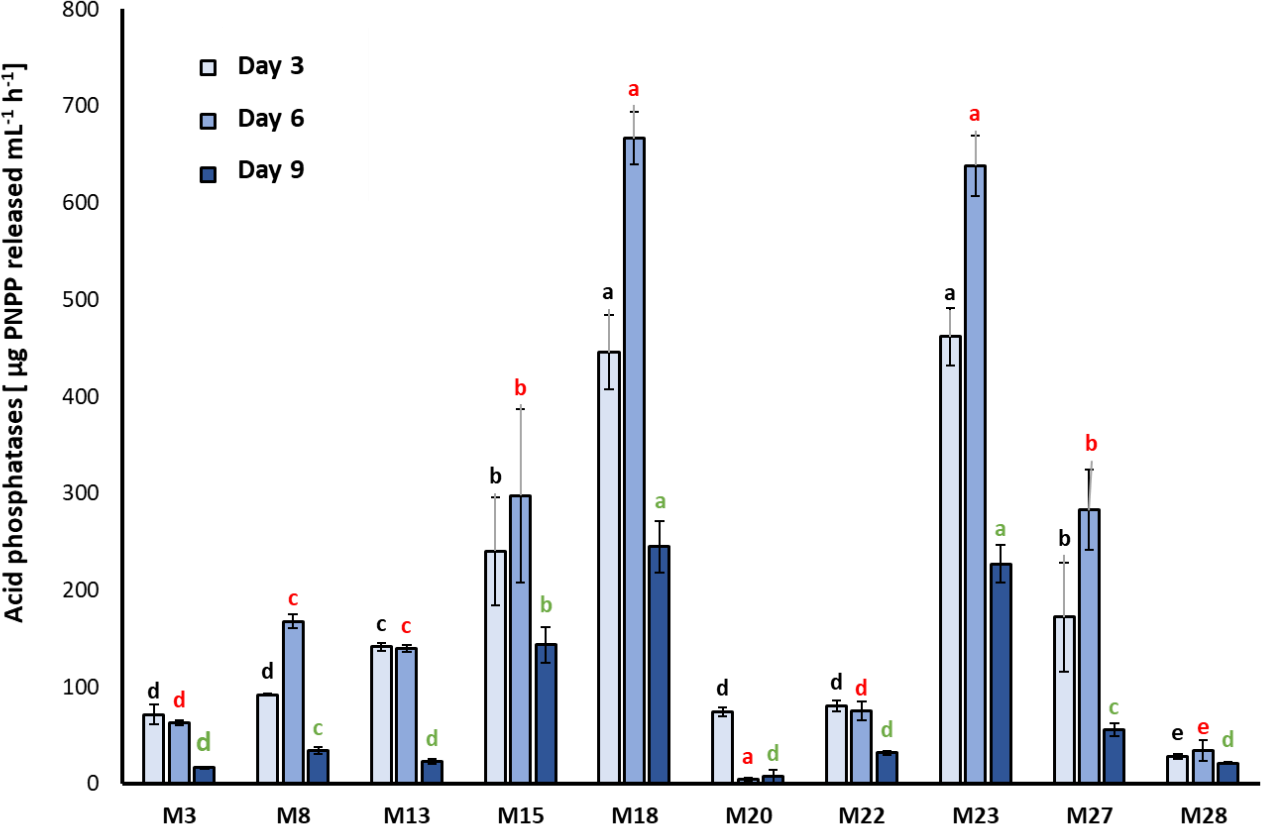
Figure 1. Activity of the acid phosphatase enzyme determined in bacterial strains seeded in Pikovskaya (PVK) (mean ± standard deviation, n = 3); means with different letters on the bars indicate statistically significant differences according to Tukey’s test (α = 0.05). Isolates were compared by grouping sampling times
The pH in PVK decreased along with the incubation time of the culture (F2, 98 = 1.049; p = 0.0002) is shown in figure 2. After three days of incubation, M15, M20, and M22 showed significant acidification in the culture medium, going from pH 7.0 (initial) to 3.90 ± 0.09, 3.85 ± 0.19, and 3.61 ± 0.32, respectively (Chi2= 30.131; df = 10; p = 0.00081).
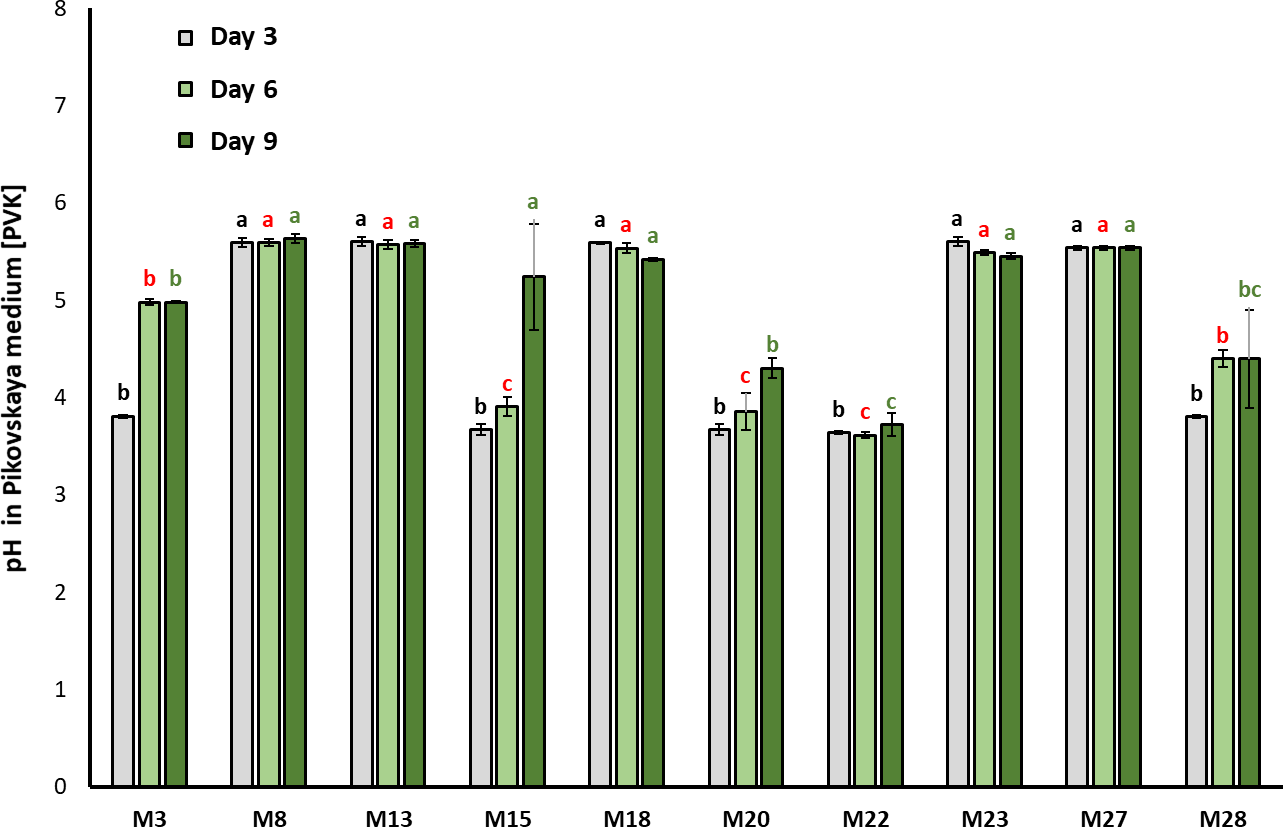
Source: Elaborated by the authors
Figure 2. Evolution of the pH in PVK during bacteria growth (mean ± standard deviation, n = 3); means with different letters on the bars indicate statistically significant differences according to Tukey’s test (α = 0.05). The isolates were compared by grouping sampling times
Determining the phosphate solubilization percentage
M15 and M18 showed greater significance in their ability to release PO43- in the medium (F 13, 41 = 58.04; p < 0.001; Tukey’s HSD α = 0.05), with an efficiency of 47% in phosphate solubilization under in vitro conditions and values of 1,460 and 1,458 mg/L, respectively, similar to the positive control (Penicillium jantinellum) (figure 3). The phosphate solubilizing activity observed in M15 and M18 remained after six months of storage at -20 °C, with values similar to those obtained at time 0; we observed the contrary in the other isolates whose capacity was not detectable after this storage time. For M28, we noticed low production of acid phosphatases in the evaluated times, for which it was excluded from subsequent tests.
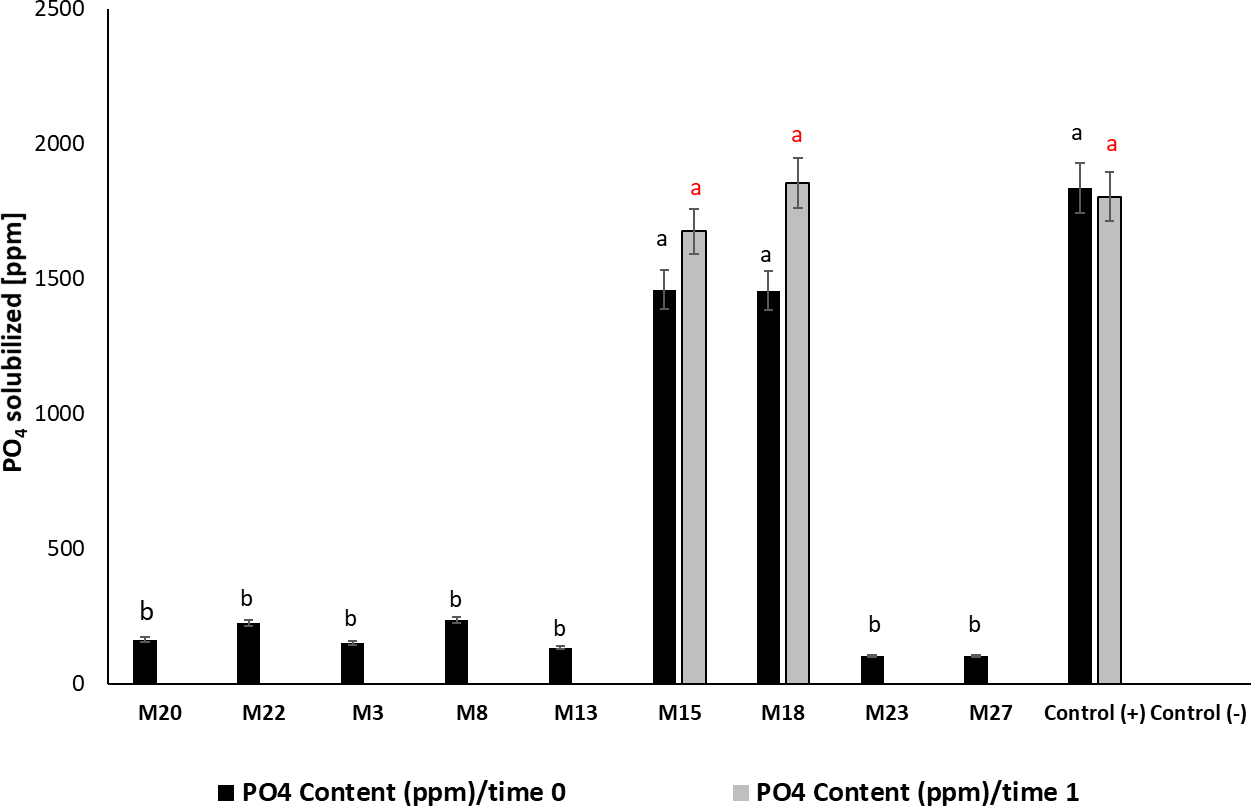
Source: Elaborated by the authors
Figure 3. PO43-content solubilized by the strains selected as potential phosphate solubilizers. Time 0: pure colonies of the isolated microorganisms; Time 1: evaluation of the activity six months after storing the biological material at -20 °C; (mean ± standard deviation, n = 3); means with different letters on the bars indicate statistically significant differences according to Tukey’s test (α = 0.05).
Determining indole compounds
Regarding the determination of indole compounds, M15 and M18 showed productions of 0.229 and 0.05 mg/L, respectively.
Molecular identification of the strains
Considering the previous results, the research continued with the identification of the promising strains M15 and M18 selected due to the production of acid phosphatases and solubilization of P in the in vitro culture medium. Taxonomic analyses of the assembled 16S rRNA sequence, compared with the NCBI and Greengenes nr/nt database, indicated that M15 displayed 99.1% identity at 100% of its length, with sequences of ribosomal gene 16S, belonging to Burkholderia ubonensis (GenBank accession number MN935186.1); in the case of M18, it could be identified as belonging to the Burkholderia cepacia (Bcc) complex (GenBank accession number MN935187.1).
Virulence tests of bacterial strains
The results of the molecular identification of M15 (Burkholderia ubonensis) and M18 (Burkholderia cepacia) made it possible to evaluate two virulence factors at the in vitro level. In the tests carried out with the two strains mentioned, the presence of diffusible pigments associated with the production of toxoflavin was not observed in King B during the evaluated times compared with the positive controls (figure 4).
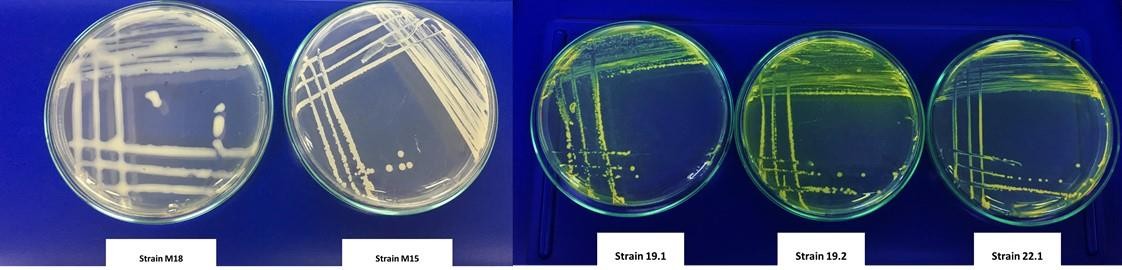
Source: Elaborated by the authors
Figure 4. Evaluation of diffusible pigment production in King B. From left to right: M18 (B. cepacia) and M15 (B. ubonensis). Positive controls of B. glumae: 19.1; 19.2; 22.1.
The onion bulbs showed that the concentrations of 103 and 105 CFU/mL were used in the inoculations with M15 (B. ubonensis) and M18 (B. cepacia) did not cause damage to plant tissue. However, M15 (B. ubonensis), inoculated at a concentration of 108 CFU/mL, caused a lesion at the puncture site, evident by a color change in the tissue, indicating the presence of degrading enzymes. Likewise, we observed this change in the bulbs inoculated with the positive controls (B. glumae) at the same concentration. We also noted no symptoms in onion tissue inoculated with M18 (B. cepacia) at the three cell concentrations tested. Although the lesions in onion bulbs with M15 (B. ubonensis) were recognized, it should be mentioned that, at the maximum concentration evaluated in the different tests carried out in this study, it showed greater capacity for producing acid phosphatases, enzymes in charge of mineralizing organic P in the soil.
Evaluating growth promotion in rice plants under greenhouse conditions
When analyzing the dry leaf biomass in the rice plants sown in soil with low P content, we observed no statistically significant differences between fertilized and individually inoculated/co-inoculated treatments, but we did for the control treatment (T1), whose plants were neither inoculated nor fertilized, providing the lowest values for this variable (Tukey: p < 0.05) (figure 5).
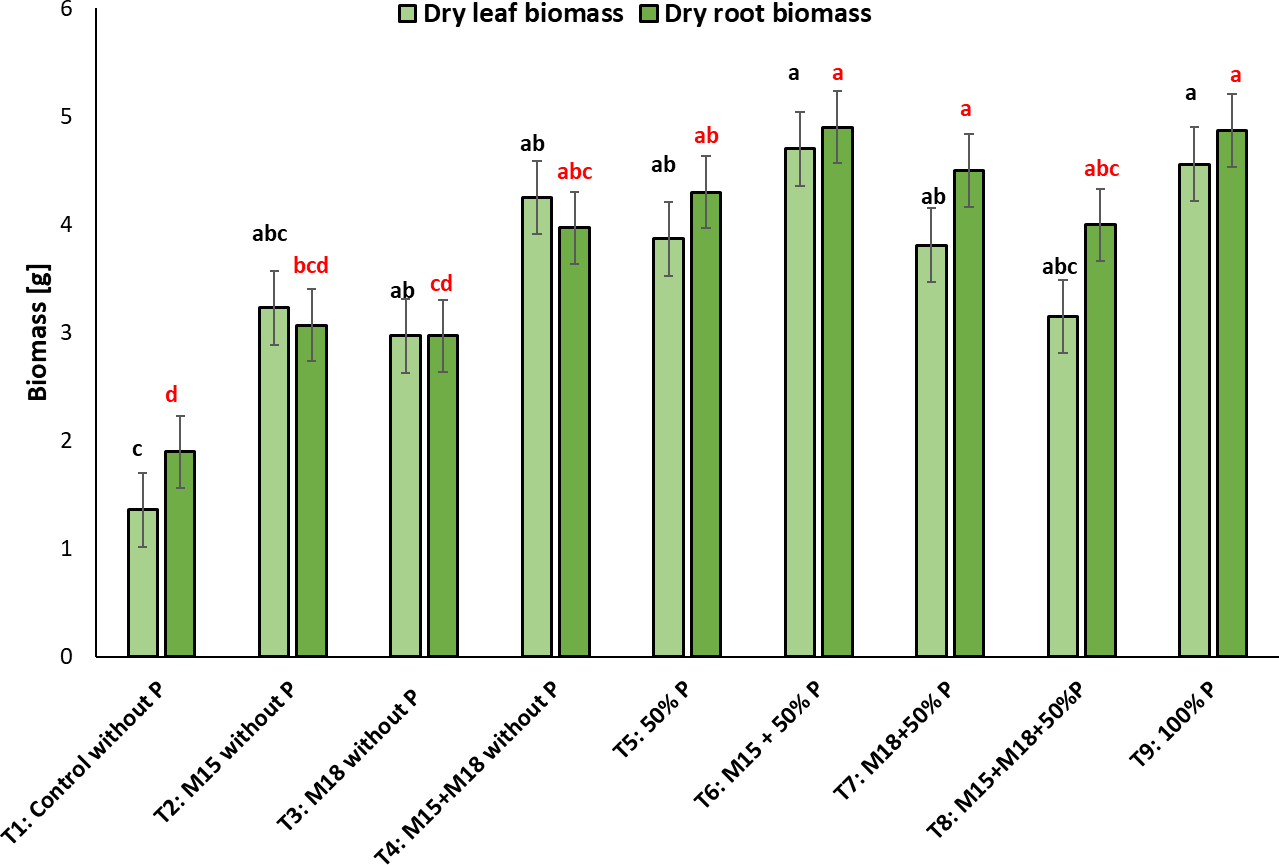
Source: Elaborated by the authors
Figure 5. Dry leaf and root biomass (g) taken from rice plants inoculated with M15 (B. ubonensis) and M18 (B. cepacia) in the flowering stage (mean ± standard deviation, n = 6); means with different letters on the bars indicate statistically significant differences according to Tukey’s test (α = 0.05).
It is worth highlighting the trend observed in the treatments inoculated with the strains that were not fertilized, showing no statistically significant differences compared to the chemical treatment fertilized with the 100% P dose. In T4, in which the rice was co-inoculated with M15-M18 and not fertilized, the plants reached a biomass production statistically similar to T9, in which the plants were fertilized with 100% of the recommended P dose for this plant species, demonstrating the role of solubilizing bacteria in the soil with low availability of this element and making its acquisition more efficient.
Regarding dry root biomass, we noticed that T1 (control) showed the lowest value for this variable with 2.0 ± 0.1 g, associated with limited root development of the plants as a result of the low P availability in the soil. There were statistically significant differences in relation to T4, T5, T6, T7, T8, and T9, where the biomass weight was similar, with values between 4.0 ± 0.3 g and 5.0 ± 0.3 g.
About the number of panicles, we observed a more significant number of structures in T4, where the plants were co-inoculated with bacteria and not fertilized with P, followed by T6, T7, T8, and T9. Regarding the weight of panicles, we noted that T6, T7, T8, and T9 showed the highest values for this variable with 2.23 ± 0.2 g, 2.27 ± 0.03 g, 2.10 ± 0.02 g, and 2.10 ± 0.1 g, respectively, compared to the other treatments, where the range was between 0.95 ± 0.05 g and 1.9 ± 0.1 g (figure 6).
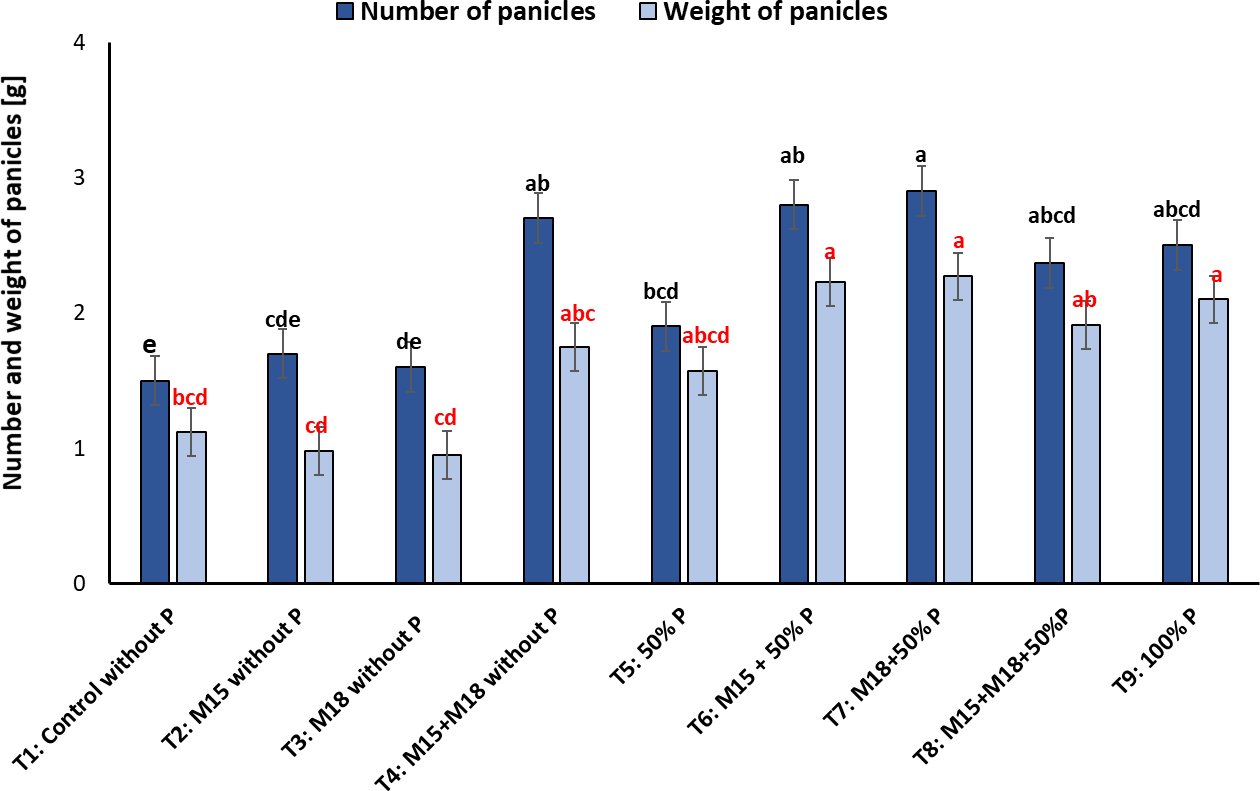
Source: Elaborated by the authors
Figure 6. Number and weight of panicles in rice plants inoculated with M15 (B. ubonensis) and M18 (B. cepacia) sampled in the flowering stage (mean ± standard deviation, n = 6); means with different letters on the bars indicate statistically significant differences according to Tukey’s test (α = 0.05).
Discussion
P deficiency is the major limiting factor for crop growth in tropical Oxisols (Fageria & Baligar, 2008). PSB in the rhizosphere that makes this nutrient available to plants would reduce the use of chemically synthesized fertilizers in crops of importance for high plains, such as corn, soybean, and rice. It is noteworthy that PSB population concentration is influenced by factors such as type of vegetation/cultivar, pH, root exudates, management practices, microbial activity, fertilizer application, and local environmental conditions, including temperature and humidity (Rooney & Clipson, 2009). Thus, in this study, 14 bacterial strains were selected from Oxisols from various locations that, when evaluated under in vitro conditions, showed potential for phosphate solubilization.
The amount of PSB found in this study was in the range reported by Caballero et al. (2007), who showed that, in savanna, weed, and soybean cultivated soils in the same study area, the PSB counts varied between 9.6 × 105, 2.5 × 105, and 7.6 × 106 CFU/g soil, respectively, and as described by Bhardwaj et al. (2017) in soils cultivated with cauliflower (Brassica oleracea var. botrytis L.) with populations of 1.3 × 106 and 2.2 × 106 CFU/g soil. In contrast, counts higher than those reported by Becerra et al. (2011) for soils in cape gooseberry producing areas (2.9 × 104 and 3.6 × 105 CFU/g soil) were found in Cundinamarca (Colombia).
Regarding the SI values, the indicators for the strains selected in this study are similar to those presented by Collavino et al. (2010). For rhizosphere bacteria isolated from Ultisols in Argentina of the Acinetobacter, Burkholderia, Enterobacter, Exiguobacterium, Pantoea, and Pseudomonas genera, they determined an SI between 1.1 and 2.7, using TP as a source of P. The results are also comparable with those reported by Motamedi et al. (2016) with Gram-negative bacteria from soils cultivated with cabbage, with SI between 1.3 and 4.7. Furthermore, Alam et al. (2002) evaluated bacteria isolated from the corn rhizosphere under in vitro conditions and found that the SI ranged between 1.2 and 6.3 at 24 h of incubation, increasing the solubilization halo with the expansion of the colony diameter after seven days of testing. Other studies selected phosphate solubilizing microorganisms from the rice rhizosphere based on SI and changes in the medium pH, obtaining values that ranged from 2.1 to 6.2 at 24 h of incubation and a pH that went from 5.96 to 2.75 (Rashid et al., 2004).
The halo around the ring formed by the bacteria evaluated under in vitro conditions would be mainly associated with organic acid production. It was evident by the color change in the culture medium supplemented with the source of insoluble P, which went from purple to yellow due to acidification (Bashan et al., 2013). This condition can vary over time and is considered a criterion for selecting the PSB (Chung et al., 2005; Puente et al., 2004).
Regarding the production of acid phosphatases, M15 (B. ubonensis) and M18 (B. cepacia) showed high activities, compared to those reported by Oliveira et al. (2009) for Burkholderia and Pantoea, isolated from the corn rhizosphere and producing 3.57 and 50.2 µg of PNPP/mL/h, respectively. They are the same as those reported by Acosta-Martínez et al. (2007) for bacteria that had values of 498.6 µg of PNPP/g/h. Other studies carried out with Oxisols from the Brazilian Amazon proved that phosphatase activity in soils could reach values close to 700 µg of PNPP/g/h (Soltangheisi et al., 2019).
PO43− values greater than 1,400 mg/L and an efficiency of 47% in phosphate solubilization indicate that the isolated strains of Colombian Oxisols have a high potential for phosphate solubilization. Contrasting results were presented by Pérez et al. (2007), who obtained bacteria of the Burkholderia, Serratia, Ralstonia, and Pantoea genera from Venezuelan Ultisols that exhibited solubilization ranges between 188 and 375 mg/L under in vitro conditions, using TP as a source of P. In particular, other studies determined Burkholderia anthinaen and Pantoea agglomerans bacteria with solubilization capacities of 600 mg/L at 48 h of incubation (Walpola & Yoon, 2013). Likewise, Oliveira et al. (2009) isolated potential phosphate solubilizing microorganisms from the corn rhizosphere in Brazil and noticed that Bacillus and Burkholderia were the most predominant and effective genera, solubilizing 67% and 59% of the total P, respectively, after ten days of testing. Bacteria of the Klebsiella and Burkholderia genera, isolated from the sugarcane rhizosphere, displayed solubilization percentages that varied between 41% and 63% sown in NBRIP supplemented with TP (Dar et al., 2014).
M15 (B. ubonensis) and M18 (B. cepacia) selected as PSB produced low amounts of indole compounds compared to other Burkholderia strains, which have been reported as producers of this type of compound using the same method for this determination. Such is the case of Burkholderia heleia PAK1-2, with yields close to 100 µM and the ability to inhibit pathogens such as Burkholderia plantarii (Wang et al., 2016), and Burkholderia pyrrocinia, with a maximum indole compound production of 6.9 mg/L after 36 hours of in vitro culture (Liu et al., 2019). In other studies with bacteria isolated from the mango Mangifera indica L. (Anacardiaceae) rhizosphere reported the production of 2 ± 0.2 mg/L of indole compounds for Burkholderia caribensis (De los Santos-Villalobos et al., 2015). Panhwar et al. (2012) found that PSB in soils cultivated with dry rice in Malaysia ranged between 0.74 and 4.34 mg/L of indole compound production. Moreover, Dawwam et al. (2013), in a similar study, found indole compound concentrations between 0.6 and 10.73 mg/L for bacteria from the sweet potato (Ipomoea batatas) rhizosphere in the culture medium supplemented with tryptophan. Among the indole compounds, the leading phytohormone is indole acetic acid (IAA), which can be produced by rhizosphere microorganisms and is responsible for regulating processes such as cell division, elongation, differentiation, and pattern formation in plants (Sahasrabudhe, 2011).
The presence of Burkholderia sp. in tropical Oxisols has been scarcely documented; however, Oliveira et al. (2009) identified the species B. cepacia in the Sete Lagoas region in Minas Gerais, Brazil, with potential for phosphate solubilization. The genus Burkholderia, comprising more than 60 species, inhabits a wide range of ecological niches, given its versatile metabolism (Coenye & Vandamme, 2003; Sousa et al., 2011; Suárez-Moreno et al., 2012), being widely distributed in the rhizosphere of various crops and establishing different interactions with plants by colonizing the roots, stems, or leaves.
This genus of Gram-negative bacteria can carry out processes associated with promoting plant growth, such as converting atmospheric nitrogen to ammonia through biological nitrogen fixation, producing phytohormones such as IAA, solubilizing phosphates, and controlling pathogens (Caballero-Mellado et al., 2004; Estrada-De los Santos et al., 2001; Suárez-Moreno et al., 2012; Zhao et al., 2014). Thus, the bacteria in this group have been classified as potential promoters of plant growth and bioinoculants for agriculture in crops such as corn (Zhao et al., 2014), pineapple (Borges-Baldotto et al., 2010), tomato (Gao et al., 2015), banana (Weber et al., 2007), among others.
Authors such as Mora and Toro (2007) and Useche et al. (2004) reported species of the B. cepacia (Bcc) complex for their ability to colonize the rhizosphere of rice and corn, crops of economic interest in Venezuelan acid soils, as well as in Colombian Amazonian Oxisols with demonstrable phosphate solubilizing activity. Besides, Ahmed and Shahab (2015) and Sashidhar and Podile (2010) found that bacteria belonging to the genus Burkholderia are more efficient in solubilizing mineral phosphates due to the secretion of organic acids into the extracellular medium as solubilization mechanisms.
Although several species of the genus Burkholderia are known for their participation in plant growth promotion processes, they have also been reported as causal agents of diseases in humans, animals, and plants (Li et al., 2013; Rojas-Rojas et al., 2019; Sinha et al., 2017; Stoyanova et al., 2007). The positive result observed with M15 (B. ubonensis) makes clear the phenomenon associated with the quorum sensing mechanism (Kwak et al., 2020; Venturi et al., 2004), indicating that temperature and population density were optimal under the evaluated conditions. It points to the phenotypic expression of the strain’s virulence genes in onion plant tissue, possibly due to lipase-like enzyme action produced extracellularly, with heat-soluble characteristics whose action is unknown in plants (Yang et al., 2016).
Additionally, Stone et al. (2012) reported B. ubonensis strains isolated in Australia with the unusual type B lipopolysaccharide from Burkholderia pseudomallei. This finding is supported by molecular studies suggesting a high diversity of this virulence factor in B. ubonensis and showing that it is independent of the geographical area of isolation and that the same factor could be present in strains of different origin (Price et al., 2017). Therefore, we could infer that, for the results found with M15, it would be due to the effect of the expression and regulation of various virulence factors (Leitão et al., 2010).
In the present study, the application of B. ubonensis and B. cepacia individually and co-inoculated significantly promoted growth in rice plants under greenhouse conditions, hence demonstrating the beneficial effect of these microorganisms, either by making the use of low amounts of phosphorus in the soil more efficient or by incorporating it through fertilization (Bhattacharyya et al., 2016). Similar results reported by Pande et al. (2019) proved that B. cepaciaand B. contaminansinoculated in sweet corn increased the dry leaf biomass of the plants with values of 0.48 and 0.32 g, respectively, compared with the control treatments whose value was 0.20 g. Estrada et al. (2013) tested the inoculation of rice with B. vietnamiensis and Herbaspirillum seropedicae as PSB in the vegetative stage, showing a significant increase in the total biomass of the plants with values of 15.25 and 11.22 g, respectively, compared with the uninoculated control, whose value was 5.86 g. These results illustrate the advantages of Burkholderia sp. as a phosphate- solubilizing bacterium and its effect on increasing crop yield.
Conclusions
From the Oxisols of the high plains in Meta, Colombia, we isolated, characterized, and identified two strains of the genus Burkholderia .i.e., B. ubonensis [M15] and B. cepacia [M18]), which showed a remarkable capacity for phosphate solubilization. This study revealed that inoculation of B. ubonensis (M15) and B. cepacia (M18) in rice significantly increased growth in terms of dry leaf and root biomass, under greenhouse conditions, in soil with low P availability. M15 and M18 would have the potential to be incorporated as active ingredients in inoculants, with capacities to promote plant growth and favoring P availability in the soil, and could even be compatible with phosphoric fertilization.