Introduction
Sustainability assessment is one of the most relevant approaches to mitigate the impacts of inadequate crop management on the environment, society, and the economy. It involves three pillars or the triple bottom line (Elkington, 1998): economic viability, social equity, and ecological integrity (De Luca et al., 2017). However, agricultural sustainability is not easily measured (Pollesch & Dale, 2015). In addition to being multidimensional, it can be carried out at different geographic scales: global (e.g., Kanter et al., 2018; Repar et al., 2017; Rockström et al., 2017; Soussana, 2014; Tittonell, 2014), national (e.g., Ghisellini et al., 2014; Kucukvar et al., 2014; Rinne et al., 2013; Roy & Chan, 2012), regional (e.g., Gaudino et al., 2014; Gerdessen & Pascucci, 2013; Milder et al., 2014; Triste et al., 2014; Walraevens et al., 2015), and farm or production unit (e.g., Bockstaller et al., 2015; Marchand et al., 2014; Peano et al., 2014; Schader et al., 2016).
A series of methods, methodological approaches, frameworks, or protocols have been developed for agricultural sustainability assessments (De Olde, Oudshoorn, Sørensen, et al., 2016; Marchand et al., 2014; Schader et al., 2014; Schindler et al., 2015), whose objective is to quantitatively compare systems, methods, alternatives, or trends in agricultural production (De Olde, Oudshoorn, Sørensen, et al., 2016; Dizdaroglu & Yigitcanlar, 2014; Paracchini et al., 2015; Peano et al., 2014; Schindler et al., 2015). These tools are typically built from indicators that systematically monitor the system and its modifications, thus identifying processes that may be out of threshold limits (De Olde, Oudshoorn, Sørensen, et al., 2016). Within the environmental dimension of agricultural sustainability, there are usually indicators associated with soil properties, whose choice depends on the nature and purpose of the analysis to be carried out.
Agricultural activities involving soil could have the following impacts: accumulation of toxic substances in harvested products, pollution of water or atmosphere (if the amount of applied fertilizer exceeds the soil’s buffer capacity), wind or water erosion, compaction due to machinery traffic and overgrazing, salinization (by irrigation, especially in arid regions or soils with poor drainage), nutrient depletion, structure alteration, and soil biodiversity reduction. These impacts endanger agricultural production and, therefore, food security (Blume et al., 2016). Sustainable use of soil depends on environmental conditions, soil characteristics, and its management. These factors interact under the system principle, where changing one factor alters the others.
This review aims to i) assess the relationship between agricultural sustainability and soil properties, processes, and management and ii) review the indicators used in agricultural sustainability assessments, which derive from physical, chemical, and biological soil properties and associated processes.
Materials and methods
A preliminary review was made in Google Scholar to obtain the desired information, downloading the relevant publications from the journals’ websites. The search included all terms —soil, agriculture, and sustainability—, mainly in titles and anywhere in the article. From this search, we selected the publications that reported on the effects of agricultural practices associated with soil management on the three dimensions of sustainability (environmental, economic, and social). A time scale of 20 years until 2019 was established for the literature search. Subsequently, the search focused on the agricultural sustainability indicators associated with soil management. For this, we considered publications that addressed the three dimensions of sustainability of agricultural production systems only. The cited literature in this review includes scientific articles, academic books and book chapters, academic conference proceedings, and public reports from recognized international agricultural research organizations.
This review included 127 references describing or linking agricultural sustainability to soil management. Three classes of publications were identified: i) publications that define agricultural sustainability and showed information regarding its assessment (40), ii) publications that contextualize soil management in agriculture (63), and iii) publications that evaluated agricultural sustainability through indicators under field conditions (37). Note that some publications are grouped into more than one class. The following criteria were used to include the publications in this review:
Accepted by academic peers
Oriented to evaluate the performance of agricultural sustainability, i.e., including and harmonizing the environmental, social, and economic dimensions of the system (classes i and iii)
Assessed sustainability based on indicators (class iii)
Included indicators for the three dimensions of sustainability (environmental, social, and economic) (class iii)
Soil and agricultural sustainability
In addition to being modified depending on its management, the method of production, and the study region, the soil is subject to erosion, acidification, alkalization, salinization, structure destruction, compaction, loss of biodiversity, nutritional imbalances, decreased damping capacity, and pollution from natural or anthropogenic sources. All the above leads to its degradation (Bone et al., 2010), making agricultural sustainability assessments involving soil even more complex (Keesstra et al., 2016). A sample of this is sustainable development goals (SDGs) 2, 3, 6, 11, 13, 14, and 15, in which soil properties and functions are relevant to food security (SDGs 2 and 6), food safety (SDG 3), pollution of water sources (SDG 14), urban development (SDG 11), and sustainability of terrestrial ecosystem services (SDG 15) (Bouma et al., 2019; Tóth et al., 2018).
Soil management practices with the highest impact on agricultural sustainability
The agricultural activities associated with the soil that directly influence agricultural production system sustainability include fertilization, tillage, and irrigation, which impact the soil, water sources, and the atmosphere.
Fertilization
Fertilization is one of the processes with the highest environmental (Bojacá et al., 2014; Cellura et al., 2012) and economic impact on agricultural production mainly because the loss of soil fertility, which results in reduced productivity, is commonly compensated for by increasing the amount of fertilizer applied (Tilman et al., 2002). The use of nitrogen fertilizers has increased about seven times, while crop yields without N fertilization have been reduced 2.4 times since the 1960s (Hirel et al., 2011; Spiertz, 2010; Tilman et al., 2002). Something similar occurs with phosphorus since its consumption has increased six to seven times since 1960, with about 85 % of this element used for fertilizer production (Cordell et al., 2009). P fertilizers are manufactured from phosphate rock, a non-renewable resource that may be depleted in 50 to 100 years (Therond et al., 2017). Unlike phosphorus, potassium fertilizer reserves are high, although it has been reported that large areas of agricultural soils around the world (e.g., Australia and China) have deficiencies (Römheld & Kirkby, 2010). More than 90 % of the mined potassium is used to manufacture potash fertilizers (Rawashdeh & Maxwell, 2014; Rawashdeh et al., 2016), whose global consumption has increased from 8.8 million Mg in 1961 to 29.1 million in 2008 (Rawashdeh et al., 2016). Potassium fertilizers are typically applied in smaller amounts than N or P, so less than 50 % of the K uptake by crops is replenished (Zörb et al., 2014).
Tillage
The intensive and long-term practice of tillage has led to significant soil degradation in many parts of the world (Loaiza et al., 2018). During tillage, the mechanical forces cause soil macro-aggregate alteration due to fracturing or compaction (Acar et al., 2018; Blanco-Canqui & Lal, 2004). This practice not only alters the soil structure but also influences organic carbon dynamics (oxidizing organic matter faster than its replacement rate) (Barto et al., 2010; Loaiza et al., 2018; Usman et al., 2018), microbial activity (Lemtiri et al., 2018; Lori et al., 2017; Van Capelle et al., 2012), and greenhouse gas emissions (Stavi & Lal, 2013). Additionally, due to the alteration of soil structure by tillage, it has been established that this cultivation practice is one of the leading causes of erosion in cultivated areas, as it accelerates surface runoff and soil loss (Wang et al., 2018).
Irrigation
Because irrigated areas have tripled in the last 50 years (Food and Agriculture Organization [FAO], 2011), 70 % of water extractions and 80-90 % of the world’s fresh water consumption are dedicated to irrigation (Drechsel et al., 2015; Therond et al., 2017). On average, two liters of water per person are enough for daily hydration, but around 3,000 liters are needed to meet their daily food needs (agricultural and agroindustrial production) (Drechsel et al., 2015). In many irrigated regions, water scarcity and salinization problems have increased sharply (Foley et al., 2011; Gomiero et al., 2011). Irrigated agriculture only accounts for 20 % of the total cultivated land area but produces 40 % of the world’s food (FAO, 2011).
Effects of soil management practices on agricultural sustainability
Soil degradation
Soil degradation is associated with a decrease in soil quality, which, in agricultural terms, implies the reduction of its production capacity (Lal, 2015; Usman et al., 2018). This degradation is a function of the relief (geomorphology), soil characteristics (physical, chemical, and biological properties), climate (temperature and precipitation), and management practices (intensive or conservation). These factors act as catalysts since soils are highly resistant or vulnerable to degradation depending on their features and interaction. This phenomenon is particularly problematic in arid and sub-arid zones, where evapotranspiration exceeds precipitation (Usman et al., 2018) (table 1).
Table 1. General description of soil degradation processes associated with agricultural activities
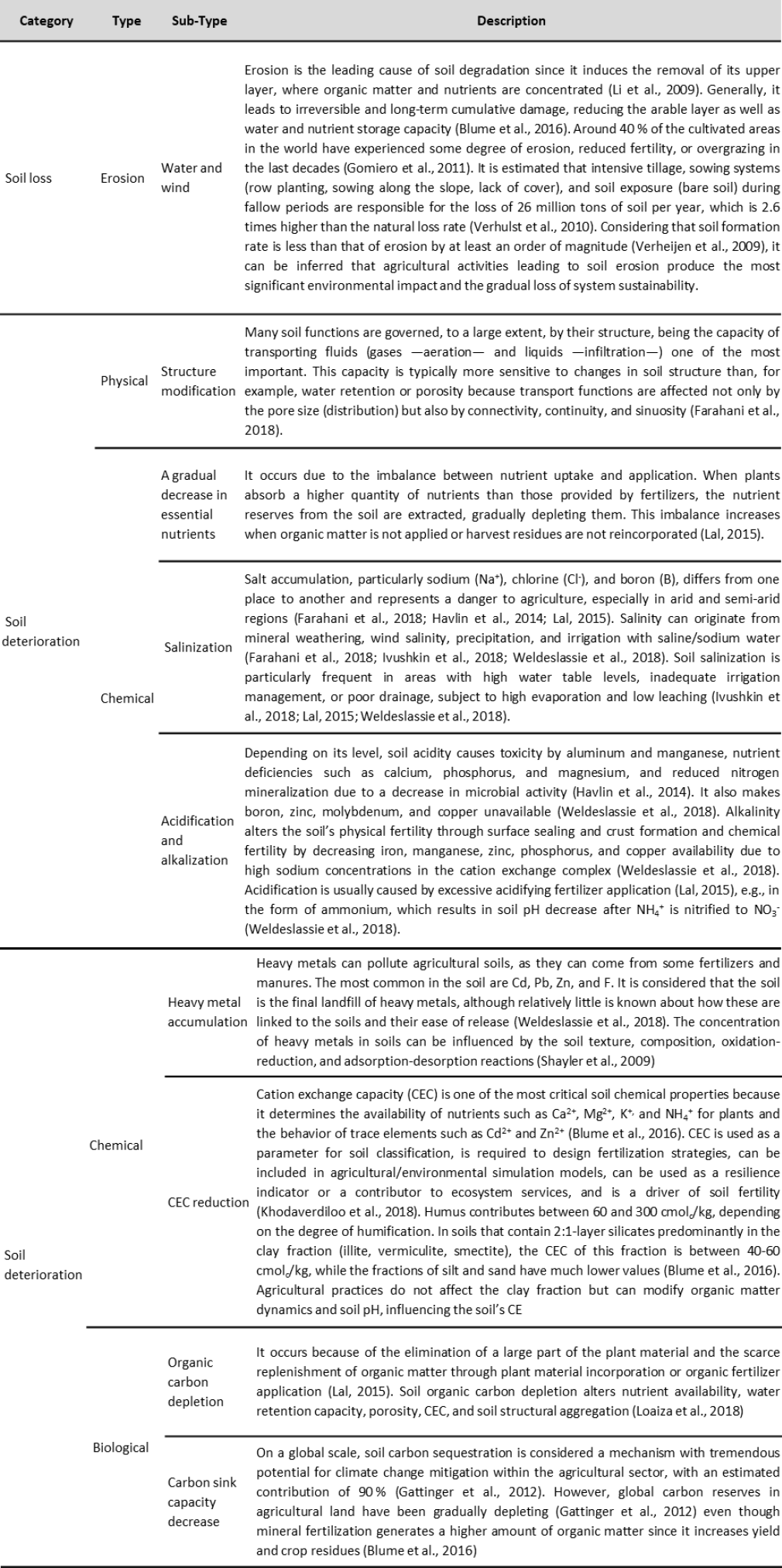
Source: Adapted from Lal (2015) and Oldeman (1994)
Greenhouse gas (GHG) emissions from soil to atmosphere
Lithosphere contains the largest reserve of carbon and nitrogen (without considering atmospheric N) on the planet, with 108 Pg (1015 g) and 1.64E1011 Tg (1012 g), respectively, stored mainly on the surface (1 m deep) (Nieder & Benbi, 2008; Schaufler et al., 2010). Soils naturally emit carbon dioxide (CO2), methane (CH4), and nitrous oxide (N2O), which are essential GHGs (Oertel et al., 2016). However, agricultural systems intensify biochemical processes in the soil, increasing N2O (1.7–4.8 Tg N2O/yr) (Baggs, 2011; Boeckx & Van Cleemput, 2001; Bouwman et al., 1995; Ciais et al., 2013; Intergovernmental Panel on Climate Change [IPCC], 2013), CO2 (180 Pg CO2 accumulated during 1750–2011) (Ciais et al., 2013), and CH4 emissions (up to 6,950 μmol CH4 m-2/h) (Oertel et al., 2016). Agriculture generates 52 and 84 % of the CH4 and N2O global emissions, respectively (Smith et al., 2008), and at the same time, these two gases have 25 and 298 times more global warming potential than CO2, respectively (IPCC, 2013). It is necessary to consider that CO2 (30–95 years) has a longer half-life than CH4 (12 years) but shorter than N2O (121 years) (IPCC, 2013). The net flow of CO2 from agricultural soils is small compared to industry emissions (Smith et al., 2008). However, emissions from industrial manufacture of agricultural inputs using fossil fuels (e.g., plastic and fertilizers) must be added to this flow, which results from soil alteration by tillage and fertilization activities (Arizpe et al., 2011; Smith et al., 2008; West & Marland, 2002). Properties and processes in the soil, such as humidity, pH, nutrient concentration, temperature, exposure, air pressure, wildfires, type of coverage, and change in land use are the main drivers of GHG emissions from the soil to the atmosphere (Oertel et al., 2016).
Nutrient leaching
Under natural conditions, ions are constantly leached from the soil surface into groundwater, varying in intensity according to the magnitude and frequency of rainfall and soil characteristics (Laird et al., 2010). Under agricultural conditions, the leaching of nutrients, such as nitrogen and phosphorus, into aquatic systems is greatly exacerbated (Yao et al., 2012), generating eutrophication because of the excessive production of photosynthetic aquatic microorganisms in marine life and freshwater ecosystems (Karaca et al., 2004). These microorganisms, typically adapted to environments with low nutrient content, rapidly reproduce in the presence of high nutrient concentrations, thus consuming oxygen from the aquatic environment and causing the death of other oxygen-dependent organisms (Dempster et al., 2012).
On average, plants do not take up 50 % of the nitrogen applied through fertilizers (Drechsel et al., 2015; Hoang & Allaudin 2010). This nitrogen is leached into water sources or volatilized into the atmosphere (Galloway et al., 2003), with consequent adverse environmental effects on aquatic ecosystems, climate change, and human health (Bodirsky et al., 2014; Camargo & Alonso, 2006; Giles, 2005; Umar & Iqbal, 2007).
About 25 % of the phosphorus extracted since 1950 has accumulated in landfills or ended up in water sources, increasing eutrophication problems (Cordell et al., 2009). Unlike nitrogen, phosphorus leaching varies substantially in time and space, and, in general, agricultural practices have a more negligible effect on phosphorus leaching than environmental conditions and soil characteristics (Ulén et al., 2018).
Not only soluble nitrogen and phosphorus (anionic form) are liable to leach. When there is an alteration in soil conditions (e.g., the addition of organic matter or mineral fertilizers), metal ions such as iron and manganese, which are usually precipitated and immobilized, can be solubilized due to fluctuations in pH and soil redox potential (Aharonov-Nadborny et al., 2018).
Increase in production costs
The way the soil is handled in agriculture not only impacts the environmental dimension of sustainability but also influences its social and economic dimensions. Agricultural practices associated with soil management, such as fertilization, tillage, and irrigation, require hiring labor, renting or purchasing agricultural machinery/equipment, and acquiring agricultural inputs. Each of these tasks creates wages or jobs and becomes an item in the list of production costs (FAO, 2016).
In general, labor is the item that represents the highest cost in agricultural production. Of the agricultural activities, soil preparation demands much labor, especially in developing countries, where specialized machinery for these tasks is not widespread. Manual labor has a high impact on the social dimension of sustainability since it creates a higher number of jobs than the mechanized option. An increase in social sustainability implies a reduction of economic sustainability and vice versa, which generates a dichotomy in sustainability analysis.
Fertilization places second in production costs since it includes fertilizers and labor costs required to obtain, mix, and apply the fertilizers (FAO, 2016). Additionally, this activity produces the most variability in production costs since it depends on the application method (manual, foliar, or fertigation), dosing (estimation method), timing (weather), and type (compound or simple, soluble or insoluble).
Indicators associated with soil properties, processes, and management
Some of the indicators used in agricultural sustainability assessments are associated with soil properties, processes, composition, and management. Indicators consider aspects inherent to the soil and those associated with soil-water, soil-atmosphere, and soil-plant systems. The indicators included in this review were grouped according to these factors and extracted from international scientific publications referencing agricultural sustainability assessments, i.e., those considering the three sustainability dimensions (environmental, economic, and social).
Soil inherent indicators
This group of indicators refers to the impact that agricultural production systems exert on the soil. The most common indicators in this group are erosion rate and soil organic matter concentration, while properties such as electrical conductivity, cation exchange capacity, exchangeable acidity, clay content, water retention capacity, apparent density, effective depth, and permeability are the least used (table 2).
Although several works reference soil quality (e.g., Rodrigues et al., 2010), we did not find an index or aggregation function to estimate it. This indicator was addressed from the individual determination of soil properties such as soil organic matter (SOM), electrical conductivity (EC), pH, cation exchange capacity (CEC), and Bi (table 2). It should be noted that soil quality is commonly estimated by integrating properties such as texture, effective depth, apparent density, water retention capacity, SOM, nitrogen, phosphorus, exchangeable bases, pH, EC, microbial biomass, potentially mineralizable C and N, and soil respiration (Garrigues et al., 2012; Hayati et al., 2010). Except for SOM, these properties are not commonly considered in agricultural sustainability assessments (table 2).
Soil-water relationship indicators
This group of indicators considers soil management’s impact on the hydrological cycle. The movement of nitrates (NO3-) from soil to surface and groundwater due to fertilizer application and organic amendments stands out within this group. This indicator is closely related to potential eutrophication (PE), an environmental impact resulting from nitrate and phosphate emissions from soil to water bodies. Aquatic eco-toxicity (AEt) is included in Life Cycle Sustainability Assessments (LCSAs), which are still under development (table 3).
Soil-atmosphere relationship indicators
This group of indicators measures soil management’s effects on the atmosphere. Some of them are the emissions of carbon dioxide (CO2 - global warming potential (GWP)), ammonia (NH3), nitrous oxide (N2O), and oxides of nitrogen (NOx) from the soil to the atmosphere, and potential acidification (PA) (table 4). Despite the evident effects of management practices on pollutants, these indicators are commonly estimated through models, conversion factors, or equivalence tables (table 4) due to the methodological difficulties and high assessment costs. In most cases, they are not considered in sustainability assessments.
Soil-plant relationship indicators
The effect of agricultural systems management on the soil-plant relationship is evaluated in two ways: from soil to plant (e.g., element consumption per kilogram produced [Elto-kg]) and from plant to soil (e.g., soil cover [SC]) (table 5). The nitrogen, phosphorus, or potassium balance indicator (NPKBal) stands out in this group and is assessed in many reviewed publications, followed by SC. Despite the information provided on the performance and efficiency of production system management, indicators such as soil-production ratio (S-Pr) and Elto-kg are not considered, even when estimating S-Pr, for example, which can be done without investing resources in laboratory analysis or expensive methodologies.
Conclusions
Practices such as fertilization, tillage, and irrigation directly impact soil, water bodies, and the atmosphere, so variations in the production system will modify the state of the agroecosystem. This fact has been known for decades, raising questions such as how to assess these variations’ impact on the production system or what to do if the impact is adverse. Regarding the first question, sustainability assessment is the most effective mechanism to determine if a crop production method, alternative, or system is environmentally, economically, and socially viable. Some of the indicators used in these assessments are associated with soil properties, processes, composition, and management. Due to its complexity, it is not easy to define which indicators best represent the agricultural production system, giving rise to a list of selection criteria whose application would help define which indicators may be the most suitable.
Agricultural sustainability assessment compares existing production systems, for instance, organic versus conventional or irrigated versus rainfed production systems. It is not common to analyze the sustainability of new alternatives for agricultural production, such as those developed from scientific experimentation, which would help answer the second question (what to do if the impact is adverse).
Sustainability assessments should evaluate at least one indicator per system (soil, soil-water, soilatmosphere, and soil-plant) to cover all the soil interactions with the environment. In this way, a broader picture of the agricultural production system’s impact on the ecosystem can be obtained.
Very few studies refer to land use and crop productivity indicators, even though this relationship reflects the overall system efficiency. Remember that one of the premises of sustainable intensification is to produce more and higher quality crops in smaller areas, which has repercussions not only on soil cover but also on the efficient management of water, inputs, human resources, food security, and the ecosystem in general.