INTRODUCTION
In aquaculture, probiotics are defined as "live microorganisms that, when administered in appropriate amounts, provide benefits to the health of the hosts" (FAO, 2001; Bajagai et al., 2016). These microorganisms are capable of secreting antagonistic substances, growth factors, essential nutrients, and digestive enzymes, which promote and improve digestion, growth, immune response, and the stress response (Nimrat et al., 2013; Hoseinifar et al., 2017). For their application in cultured aquatic organisms, they must meet certain characteristics such as a high tolerance to bile salts, growth at wide ranges of temperature, salinity, and pH, as well as the ability to adhere to mucus in the intestinal tract and not show factors of virulence (Kazuñ and Kazuñ, 2014; Dawood et al., 2018).
Currently, numerous bacterial strains of the Bacillus genus are used as probiotics to promote growth in cultured aquatic organisms (Kumar et al., 2016; Ringo and Song, 2016), especially in larval stages (Newaj-Fyzul et al., 2014; Vieira et al., 2016; Zorriehzahra et al., 2016). In this way, the effect of B. firmus strains as growth and survival promoters has been previously described in white shrimp (Litopenaeus vannamei) (Yuniarti et al. , 2013; Barman et al. , 2017), a species of commercial interest that is currently affected by bacterial diseases, especially during the larval stages (Flores-Miranda et al., 2012; López León et al., 2016).
Likewise, probiotic bacteria have been shown to have a positive effect on the productivity, population growth and fertility of organisms such as rotifers of the genus Brachionus sp. (Najmi et al., 2018), which are used as live food in the cultivation of aquatic organisms in larval stages (Gatesoupe, 1991; Hirata et al., 1998; Zink et al., 2013). Consequently, the growing demand for biosecurity in the production chain has required the use of biotechnological strategies that avoid the inappropriate use of antibiotics, and that allow improving the physiological response in the larval and post-larval stages of species such as white shrimp, as well as the quality and parameters of rotifer cultures (Vieira et al., 2016; Li et al., 2017). Therefore, the present study presents the characteristics of B. firmus C101 as a growth promoter and possible probiotic candidate for postlarvae of white shrimp (L. vannamei), and in the culture of rotifers (B. plicatilis s.s.).
MATERIALS AND METHODS
Obtaining bacterial strains and maintenance in culture media
C101 was isolated from the gills of healthy Cobia larvae (Rachycentron canadum). For the assays, C101 was grown on trypticase soy broth and agar (TSA and TSB, Becton Dickinson™) with 1 % NaCl and on a marine agar (Zobell™). Vibrio alginolyticus was obtained from a mortality episode of Horse mackerel (Caranx hippos) from a local aquarium and V. harveyi BB120 (Bassler et al., 1997), both were grown on thiosulfate-citrate-bile-sucrose agar (Becton Dickinson™), blood-lamb agar (Becton Dickinson™) and nutrient agar (Sigma-Aldrich). Aeromonas hydrophila, Edwardsiella tard, and Streptococcus agalactiae were isolated from mortalities of Tilapia (Oreochromis spp.) and were obtained from the collection of microorganisms of the Veterinary Faculty of the National University of Colombia and cultured in TSA, TSB, and sheep-blood media. For long-term preservation, the bacteria were cultivated in TSB broth and stored at -80 °C with 20 % glycerol (Murillo and Villamil, 2011).
C101 Characterization
Morphology was described through light microscopy (Olympus CX22) and the Gram character through staining (Claus, 1992). Catalase and oxidase (tetramethyl-para-phenylenediamine) tests were performed and the enzyme profile was obtained with the API-ZYM® kit (Biomérieux). C101 growth was evaluated at different temperatures (4, 28, 36, and 40 °C), salinities (0, 1, and 6.5 % NaCl), pH (2, 4, 6, 7, and 8), and bile salts (0.5, 1, 3 and 5 %). Identity was determined from partial sequencing of the 16S rRNA gene with primers 63f (5'-CAGGCCTAACACATGCAAGTC-3') and 1387r (5'-GGGCGGWGTGTACAAGGC-3 '). The readings were analyzed with the BLASTn algorithm, and a minimum of 98.65 % similarity was taken into account for the species assignment (Valenzuela-González et al., 2015).
Growth was evaluated according to Villamil and Esguerra (2017). In 50-well plates. 50 μl of the fresh culture (106 CFU mL-1) and 50 μl of the liquid medium were mixed in triplicate and incubated at 28 °C. Growth was monitored for 72 h and the optical density (OD) (absorbance or Abs at 600 nm) was read on the Modulus Microplate Reader spectrophotometer. Also, the viability and survival in seawater were estimated by re-suspending 106 CFU mL-1, incubating for 24 h at 28 °C, and performing a plate count (CFU mL-1).
Antimicrobial activity assay
The C101 extracellular products (ECPs) were extracted following the methodology described by Cabo et al. (1999) and Villamil et al. (2010) with slight modifications. For this, C101 was cultivated in broth for 24 h, the culture was centrifuged in three cycles of 3000 rpm x 15 minutes, and the supernatant (containing the ECPs) was recovered and then filtered at 0.2 μm. Subsequently, in a 96-well plate, 50 μl of the filtrate and 50 μl of the fresh pathogen culture (106 CFU mL-1) were added in triplicate. The plate was incubated at 28 °C for 24 h and the OD reading was performed. 50 μl of the culture medium was included as control with the ECPs. The change in the OD of the cultures of the pathogenic strains was expressed as a percentage of growth inhibition.
Obtaining and maintaining shrimp and rotifers
The postlarvae (PLs) of white shrimp (L. vannamei) in stage PL-21, and the rotifers (B. plicatilis s.s.) were provided by CENIACUA (Cartagena, Colombia), transported to the facilities of the Jorge Tadeo Lozano University (Santa Marta, Colombia), and acclimatized in ponds with continuous aeration. The postlarvae were placed in a glass aquarium with 32 L of filtered seawater (35 salinity and 28 °C), at a density of 16 PLs L-1, and were fed with ground Nicovita concentrate (size 500 μm and donated by CENIACUA) in three daily rations (10 % of body weight) (Membreño et al., 2014). The conditions were stable, with continuous aeration, 80 % water changes day-1, and photo-period of 12 h light and 12 h darkness. The massive culture of rotifers (B. plicatilis s.s.) was kept in filtered and sterile seawater (35 salinity and 28 °C), with stable conditions of aeration, continuous lighting, and 100 % water changes day-1, sieving them per 100 and 60 μm, and feeding them twice a day with Nanochloropsis sp. (106 microalgae mL-1).
Evaluation of B. firmus C101 as a probiotic for shrimp and rotifers
C101 was grown in TSB broth for 24 hr at 28 °C. The culture was centrifuged at 6000 rpm for 15 min at 4 °C, the pellet was washed three times with sterile saline solution (0.9 % NaCl), and resuspended in 15 ml of sterile Phosphate Buffer (1X PBS). The initial concentration of the liquid suspension was estimated by spectrophotometry (Abs at 600 nm), and verified by direct plate count and reported as the number of colony-forming units per unit volume (CFU mL-1).
Evaluation for shrimp postlarvae
Three plastic containers were placed for the treatment with C101 and three for the control (sterile PBS). In each container with 2 L of ultra-filtered seawater, 30 PLs of shrimp were sown (for a total of 180 PLs). All the culture and feeding conditions were kept stable, making 100 % water changes day-1. The C101 liquid suspension was added daily in the container water (Luis-Villaseñor et al., 2011) at a final dose of 106 CFU mL-1 * day (Zhou et al., 2009). PBS was added as a control, without the presence of C101. The experiment lasted three weeks.
At the beginning of the experiment, data on dry weight (samples incubated at 65 °C for 48 h) and fresh length of shrimp PLs (n = 10) were taken. At the end of the experiment, these same data were taken from both the controls (n = 30, that is, 10 from each replica) and the postlarvae treated with C101 (n = 30). The fresh length was determined by stereoscopy, while dry weight was measured on a digital scale (Sartorius, Cubis®). Additionally, at the end of the experiment, the number of live PLs was also determined before taking samples for analysis. The following indices were calculated with the obtained data: Weight gain (GP) (%) = (Wf - Wi)/Wi * 100, Height gain (GT) (%) = (Lf - Li)/Li * 100, Specific Growth Rate (TEC) (% day-1) = (LnWf - LnWi)/t * 100, Average Weight Increase per Day (ADG) (mg day-1) = (Wf - Wi)/t, and the Food Conversion Rate (TCA) = R/(Wf - Wi); where W is average weight, L is the average length, t is the duration time (in days) of the experiment, and R is the daily ration of the concentrated food (Abd El-Rhman et al., 2009; Nimrat et al. , 2013). The survival rate of the organisms was calculated as follows: Survival (%) = (nf/ni) * 100, where ni is the total number of individuals in each container at the beginning of the experiment and nf at the end (Nimrat et al., 2012).
Evaluation for rotifers
The rotifers were placed in Erlenmeyer with 80 ml of ultra-filtered and sterile seawater, at an initial density of 65 ± 4.2 mL-1 rotifers. The addition of B. firmus C101 was carried out for two days (one added daily, that is, every 24 h), to a final concentration of 106 CFU mL-1 * day. Both the C101 treatment and the control (sterile culture medium) were performed in triplicate. Also, all the culture and feeding conditions were constant with 100 % day-1 water replacements.
The effect of C101 administration on rotifers was determined by population growth, as measured by the change in the density of individuals, females, males, and eggs. For this, 1.0 ml of each culture was taken as a sampling unit and a count was made by light microscopy using the Edmonson method (count/volume) on a Sedgewick Rafter plate (Biologik®). Also three aliquots were taken per treatment to take into account the variability of the aliquots. All measurements were made in triplicate, and the following indices were calculated: Population growth rate (TC) (% day-1) = (Ln Nt - Ln No/t * 100), Fertility (F) (individual eggs.-1) = (# Eggs/# Females) and Productivity (R) (indiv. ML-1 * day) = (Nt - No/t * 100); where Nt is the density of rotifers at time t, No is the initial density and t is the supply time in the crop (Cisneros, 2011, 2012).
Evaluation of the number of total cultivable bacteria associated with shrimp and rotifers
After the addition of C101, a count of total heterotrophic bacteria (BT) was performed from the tissues of the post-larvae of shrimp and rotifers. For this, an individual (1.0 ml in the case of rotifers) was taken from each replicate of both the treatment and the control. The samples were washed with abundant sterile seawater and were macerated under sterile conditions. This macerate was resuspended in 0.5 ml of 1X PBS and then serially diluted to 10-6 according to Villamil et al. (2010) and Murillo and Villamil (2011). Then 0.1 ml of dilutions 10-3 to 10-6 were plated in triplicate on marine agar (Zobell™). The plates were incubated at 28 °C for 24 h, and at the end, the number of colonies forming units per milligram of tissue (CFU mg-1) and rotifer (CFU rotifer-1) was determined. All counts were carried out in triplicate.
Statistic analysis
Data were expressed as average ± standard error (ES). Assumptions of normality were tested with the Shapiro-Wilk test and homogeneity of variances (homoscedasticity) with Levene's test. One-way analysis of variance (ANOVA) was performed to test statistical differences (p < 0.05). Also, a Duncan multi-range test was used to compare the means when the differences were detected with the ANOVA. All statistical analyzes were carried out in SPSS 15.0 and Statgraphics Centurion XV for Windows (California, USA).
RESULTS
C101 characterization
The morphological and physiological characterization of C101 is described in Table 1. This isolate was identified as Bacillus firmus (98.8 % identity), and showed growth at different temperatures (between 4 and 40 °C), salt concentrations (between 0 and 6.5 % NaCl), and concentrations of bile salts (between 0.5 and 5 %), but no growth was observed at extreme pH (< 6 and > 8), or below 4 °C. The ideal growth of C101 was observed at 28 °C, 1 % NaCl and pH 7.4, conditions that were used in all subsequent tests for its cultivation. Regarding growth, it was observed that it continues to grow after 24 h and up to 72 h of incubation at a rate of 0.0191 ± 0.003 abs h-1, and also, it can survive in seawater (28 °C and 35 of salinity) with counts of 4.2 ± 1.4 x 106 CFU mL-1 of water after 24 h incubation.
Enzymatic and antimicrobial activity of C101
The enzymatic activity of B. firmus C101 is shown in Table 2. In summary, B. firmus C101 is catalase positive, oxidase negative, showed intense acid and alkaline phosphatase activity, moderate lipase/esterase (C4 - C8) and trypsin/α-chymotrypsin, and shows no activity in the hydrolysis of disaccharides. Additionally, the ECPs of B. firmus C101 showed growth inhibitory activity of different pathogens, turning out to be more active against V. alginolyticus (49.7 %), A. hydrophila (47.9 %) and S. agalactie (46.8 %) and to a lesser extent vs. E. tarda (39.6 %) and V. harveyi BB120 (12.3 % inhibition).
Evaluation for shrimp postlarvae
The initial length and average weight of the postlarvae (PL-21) of white shrimp (L. vannamei) were 70.2 ± 2.1 mm and 12.3 ± 0.02 mg respectively. After the administration of B. firmus C101 (Figure 1), a significant increase (p < 0.05) was evident for all growth indicators concerning the control treatment (Table 3).
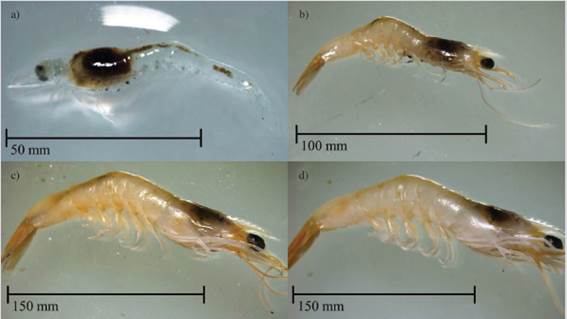
Figure 1 Postlarvae (PLs) of shrimp Litopenaeus vannamei administered with Bacillus firmus C101. a) PLs before addition, b) PLs from control (no addition), c) y d). PLs administered with B. firmus C101 (Replica 1 and 2).
Table 3 Growth and survival indicators of white shrimp (Litopenaeus vannamei) postlarvae administered with Bacillus firmus C101.

Data were expressed as average ± ES. a) There were significant differences (p < 0.05) in the averages of the treatment with C101 for the control. b) There were no significant differences (p > 0.05) for the control. Length: length, GT: height gain, TEC: specific growth rate, GP: weight gain, ADG: average daily weight increase, TCA: feed conversion rate, S: survival.
In general, an increase was observed in the length and weight of the postlarvae with the B. firmus C101 treatment, compared to those of the control. There was a marked effect on the specific growth rate (TEC = 3.8 ± 0.7 % day-1), the increase in daily weight (ADG = 1.5 ± 0.1 mg day-1) and the feed conversion rate (TCA = 1.5 ± 0.1 %), which was significantly lower (p = 0.0011) in relation to the control. Furthermore, no negative effects were observed in the survival rate (S > 90 %) of the postlarvae after the addition of B. firmus C101, nor significant differences concerning the control (p > 0.05; p = 0.3793).
Evaluation for rotifers
The initial densities in the cultures were 65.0 ± 4.2 indiv. mL-1, 44.7 ± 3.8 females mL-1, 20.3 ± 2.3 males mL-1, and 25.0 ± 3.2 eggs mL-1, and the indicators of population growth are described in Table 4. It was observed that for the number of total individuals and of females there were no differences (p > 0.05) between the initial time and the final time in the control. However, in the treatment with B. firmus C101, differences were found (p < 0.05). A significant decrease (p < 0.05) in the number of eggs in the control was also observed in the final time. The number of males was significantly reduced at the end of the experiment in control and treatment. On the other hand, a significant increase was observed in the number of individuals (p = 0.0101), females (p = 0.0009), and eggs (p = 0.00001) after the administration of B. firmus C101 concerning the control. Thus, it was observed that the effect of B. firmus C101 was more evident for the total number of rotifers (97.0 ± 3.4 indiv. mL-1) and for that of females (87.7 ± 1.9 females mL-1) and concerning the control. Population growth rates (TC), fertility (F), and productivity (R) were also significantly higher (p < 0.05) after the administration of B. firmus C101, and compared to that obtained for the control.
Table 4 Population growth parameters of cultures of rotifers (Brachionus plicatilis s.s.), administered without probiotic (control) and with Bacillus firmus C101, cultivated for 48 h.

Data were expressed as average ± ES. a) There were significant differences between the initial and final time (p < 0.05). b) There were no significant differences between the initial and final time (p > 0.05). c) There were significant differences in the means of the parameters/indices concerning the control (p < 0.05). d) There were no significant differences in the mean of the parameters concerning the control ♀ females, ♂ males, TC: population growth rate, F: fertility (egg-ratio), and R: productivity.
Evaluation of the number of total cultivable bacteria associated with shrimp and rotifers
The values of total cultivable bacteria (BT) counts from the tissues of post-larvae of white shrimp and rotifers are shown in Figure 2. In both cases, a significant increase (p < 0.05) was observed in the count in BT plate after administration of B. firmus C101, concerning those obtained for the control treatment. Regarding shrimp postlarvae, average counts of 4.9 ± 0.4 x 107 CFU mg-1 tissue were obtained with the addition of B. firmus C101. For rotifers, the average count was 2.9 ± 0.3 x 105 UCF rotifer-1 after treatment with B. firmus C101.
DISCUSSION
Evaluation of B. firmus C101 as a probiotic for shrimp postlarvae
Bacillus genus bacteria have been successful as additives in the diet of cultured aquatic organisms (Kumar et al., 2016; Ringe and Song, 2016), improving water quality (Zokaeifar et al., 2014; Tang et al., 2016) and evidencing a potential effect on the growth and survival in larval stages of different marine species of commercial interest (Vieira et al., 2016; Zorriehzahra et al., 2016). In white shrimp (Litopenaeus vannamei) culture, the positive effect of different species of this genus (ie Bacillus cereus, B. subtilis, B. pumilus, B. megaterium, B. coagulans and B. licheniformis) has been demonstrated when administered at different doses, and in freeze-dried food (108 CFU g-1) (Wang, 2007), micro-encapsulated (109 CFU g-1) (Nimrat et al., 2012), pellets (1010 CFU g1) (Zokaeifar et al., 2012; Sadat Hoseini Madani et al., 2018), liquid suspensions (106 CFU mL-1 directly in water) (Wang et al., 2016), bio-flocs (109 CFU mL-1) (Ferreira et al., 2015; Pacheco-Vega et al., 2018) and in mixture with other bacteria (107 and 109 CFU g-1) (Bernal et al., 2017; Bachruddin et al., 2018).
Furthermore, the effect of B. firmus strains on growth and resistance in tiger shrimp (Penaeus monodon) (Raghu et al., 2016; Kolanchina et al., 2017) and in L. vannamei (Yuniarti et al., 2013; Barman et al., 2017) has been previously demonstrated. In this study, it is reported that at a dose of 106 CFU mL-1 of B. firmus C101, administered directly in the water and without mixing with other strains, a significant increase in the growth and yield of the PLs of white shrimp is obtained (L. vannamei).
Compared to other studies carried out in different stages of L. vannamei (from nauplius to juveniles), the addition of Bacillus sp. strains has a marked effect on the feed conversion rate (TCA %), showing values below 1.8 % and even some equal to or less than 1.5 % (Table 4). These low values indicate rapid growth and good use of food (Membreño et al., 2014), which in turn corresponds to the height and weight gain obtained in this study. However, for direct comparisons of the effect of probiotics, the form of addition (individual or in a mixture), dose, route, and duration of administration should be considered. Thus, with the addition of B. firmus C101 directly in the culture water of the shrimp postlarvae, and with a duration of three weeks, the effect on specific growth rates, daily weight increase, and feed conversion is highly promising if it is compared with that obtained in other studies according to the type of probiotic (simple or mixed), the dose, the route of administration, and its duration (Table 5).
Table 5 Some studies of the effect of the administration of bacteria of the genus Bacillus sp. in white shrimp (Litopenaeus vannamei) of different stages.
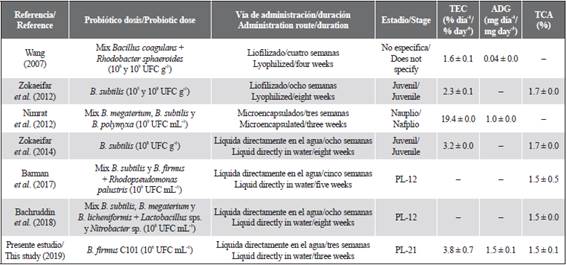
Data are expressed as mean ± SD (standard deviation). TEC = specific growth rate, ADG = average daily weight gain, and TCA = feed conversion rate.
Growth, resistance to changes in temperature, salinity, and the physiological conditions of the gastrointestinal tract (TGI) of organisms, which is slightly alkaline (pH ~ 8.0) and with a high concentration of bile salts (Heisterkamp et al., 2016), are characteristics that a good probiotic candidate must meet (Balcázar et al., 2006; Reddy et al., 2018). Following the above, the physiological profile of B. firmus C101 and the fact that some strains of B. firmus can adhere and form biofilms in the intestinal tract of aquatic organisms (Kesarcodi-Watson et al., 2008) suggest that there is a high probability of TGI colonization of white shrimp postlarvae and a high probability of survival under these conditions, therefore it would be pertinent in future researches to carry out analyzes of intestinal microbial diversity with independent culture techniques.
Additionally, the biochemical profile of B. firmus C101 suggests a high enzymatic activity of hydrolases such as lipases/esterases, chymotrypsins and phosphatases, which may be beneficial for the host, since several studies suggest that once these bacteria establish themselves in the TGI can secrete these enzymes, and in this way contribute to the degradation and absorption of essential nutrients that improve digestion and the use of food (Castex et al., 2008; Angelakis, 2017; Gobi et al., 2018). Other research indicates that the production of phosphatases by probiotics such as B. subtilis increases the absorption of elements such as calcium, iron, and inorganic phosphorus in fish species such as Epinephelus coioides and Silurus soldatovi (Liu et al. , 2010), and facilitates the elimination of phosphate esters and intestinal detoxification, which avoids inflammation problems and infections in the TGI that can lead to stress and death of the host (Lallès and Suescún, 2014).
On the other hand, it is known that some strains of Bacillus sp. show significant antagonistic activity against potential pathogens such as A. hydrophila (Yi et al., 2018), Edwarsiella tard (Ran et al., 2012), V. harveyi (Zokaeifar et al., 2012), V. parahaemolyticus (Bernal et al., 2017) and Streptococcus sp. (Liu et al., 2012), which affect the larviculture of aquatic animals (López León et al., 2016). This study highlights the inhibitory activity of the B. firmus C101 ECPs against some of these potential pathogens. However, subsequent studies on this mode of action are required as some authors suggest that this activity may be due to the secretion of compounds such as antibiotics, siderophores, bacteriocins, proteases, reactive forms of oxygen (i.e. H2O2) and lysozymes (Khochamit et al., 2015; Newaj-Fyzul and Austin, 2015; Wang et al., 2015), which could be present in the ECPs of this candidate.
Based on all of the above, B. firmus C101 is proposed as a potential probiotic candidate and growth promoter in the larviculture of white shrimp (L. vannamei), especially in post-larval stages and in closed culture systems. However, studies are required on the possible mechanisms of action of this candidate, the ability to adhere to TGI, and the effect on crop water quality.
Evaluation of B. firmus C101 as a probiotic for rotifers
In recent decades, several studies have indicated that lactic acid bacteria (i.e.: LactoBacillus plantarum, L. delbrueckii, L. acidophilus, L. rhamnosus) and of the genus Bacillus (i.e.: B. subtilis, B. megaterium, B. licheniformis, B. pumilus) have a positive effect on the yields of rotifer crops. Therefore, it has been observed that mixed cultures of these bacteria increase their density (rotifers mL-1), beneficial bacterial load (CFU rotifer-1), and nutritional composition (Hirata et al., 1998; Douillet, 2000; Najmi et al., 2018). In a previous publication (Murillo and Villamil, 2011), a remarkable effect on the growth and regulation of the B. plicatilis microbiota treated with B. subtilis CCBM-64 (107 CFU mL-1) was reported. Another study showed that mixtures of B. subtilis, B. licheniformis, B. megaterium and B. laterosporous (108 CFU L-1 each) have a direct effect on the fecundity of rotifers (egg-ratios from 0.30 to 48 h of treatment) (Zink et al., 2013). In agreement, the data of the present study suggest a remarkable effect of Bacillus firmus C101 on population growth, fertility, and productivity of B. plicatilis s.s., when administered at a dose of 106 UCF mL-1, after 48 h. However, direct comparisons of population dynamics between studies of rotifers can be complicated due to the use of different strains, mixtures, delivery mechanisms, and/or preconditions or during cultivation as the type, and quantity of microalgae supplied as a primary food (Snell et al., 1983; Abd Rahman et al., 2018).
Among the benefits of enriching rotifer cultures with this type of probiotics is modulation of the endogenous microbiota of the digestive tract, which includes increasing the load of beneficial bacteria, decreasing the load of pathogenic bacteria (Makridis et al., 2000; Planas et al., 2004); and the secretion of metabolites and essential nutrients such as vitamins (biotin and VitB12), amino acids, and growth factors (Yoshimatsu and Hossain, 2014). This contributes to the improvement of the nutritional composition (Rollo et al., 2006), reproduction rate (Qi et al., 2009), and growth rate (Rombaut et al., 1999) of rotifers. In the present study, it was observed that the administration of B. firmus C101 in crops resulted in an increase in population densities, which in turn evidences a positive effect of this probiotic candidate. However, future research on its nutritional profile is required, and an analysis of the bacterial community that in turn allows determining whether the increase in the number of total bacteria is due to the incorporation of this candidate in the native microbiota, and not to an increase in the bacterial load of possible pathogens (i.e. species of the genus Vibrio) in the tissues of the rotifers.
The importance of the present contribution in this study is that the improvement of rotifer cultures through these strategies has turned out to be an important tool for the transfer of probiotic candidates to culture organisms in larval stages (Jamali et al., 2015). Thus, these organisms can take advantage of the multiple benefits provided by probiotic bacteria, such as the decrease in diseases that affect larvae (Jeeja et al., 2011; Loka et al., 2016). For this reason, future studies are necessary to prove that the enriched rotifers can serve as a vehicle in the transfer of this probiotic candidate to the larvae of culture organisms such as shrimp, as some studies suggest that once these probiotic candidates establish themselves in the tissues and the digestive tract of rotifers (or any other type of live food) (Kostopoulou and Center, 2012), these candidates and their products can effectively reach the TGI of the larvae, which feed on these enriched live prey (Ringe et al., 2003, 2007).
CONCLUSIONS
It is possible to confirm the important role of Bacillus firmus C101 as a growth promoter in post-larval stages of white shrimp (L. vannamei) and in the improvement of rotifers population parameters (B. plicatilis s.s.), as the results presented here indicate a positive effect of the direct addition of this strain in experimental cultures. Although there are currently numerous reports on the probiotic effect of different Bacillus sp., the present study can be considered as the first report of the effect of individual administration of B. firmus in rotifers. However, additional studies are required on the possible mechanisms of action of this probiotic candidate, and pilot and commercial-scale tests to validate these results and their possible transfer to aquaculture companies.