INTRODUCTION
Cryptobenthic fish can be defined as “adult fishes usually < 5 cm that are visually and/or cryptic in behavior, having a strong relationship with benthos” (Depczynski and Bellwood, 2003: 183). On hard bottoms, such as coral reefs, these can be ~50 % of the abundance of fish, ~40 % of the number of fish species, and ~35 % of the total fish biomass (Ackerman and Bellwood, 2000, 2002). The great abundance of cryptobenthic fish larvae and the analysis of the stomach contents of reef predators suggest that they probably play an essential role in the trophic dynamics of coral reefs, participating in the recycling of biomass-derived from primary production through detrital pathways (Depczynski and Bellwood, 2003; Brandl et al., 2019). On the other hand, analysis of stable isotopes (δ15N) in tropical estuarine zones indicate a medium and high trophic level for this type of organisms, which would indicate that they behave as predators of benthic microfauna (Medina-Contreras et al., 2018).
Commonly used sampling techniques, such as visual censuses, do not allow obtaining ecological information on cryptobenthic fish communities (Willis, 2001) due to the bias towards individuals with a total length > 5 cm. This technique tends to underestimate the contribution of cryptobenthic fish, so adjustments have been made to visual censuses to study this group of organisms (Beldade and Gonçalves, 2007). In turbid waters such as in estuarine areas near mangrove forests, where visibility is low (< 1 m), visual censuses are impractical. Therefore, anesthetic and ichthyocides have been used to sample this community in these environments. (e.g. Barletta et al., 2002). These substances have restrictions in their use due to their environmental impact; however, they can provide accurate information on biodiversity inventories at small sampling scales (Robertson and Smith-Vaniz, 2008). In the case of marine protected areas, the use of these substances is restricted because they could affect other organisms that are not part of the study group, as well as the sampled ecosystems. For this reason, it is necessary to have alternative sampling methods, which include the use of standardized units that mimic the conditions of the substrate (Zimmerman and Martin, 2004; Ransome et al., 2017). These sampling units are located in specific habitats and recovered after a certain period, collecting fish and cryptic invertebrate species in an effective way. These devices can also be used to investigate settlement and recruitment events (Robertson et al., 1988; Glynn, 2006; Valles et al., 2006; Carassou et al., 2009; Mellin and Pontón, 2009).
To date, there are no studies that have documented the relative abundance of cryptic fish assemblages in the estuarine systems of the Tropical Eastern Pacific (TEP) and particularly on the Pacific coast of Colombia. For most of the species that make up this community, the only information available is restricted to their original description, unique records, and lists of species from specific areas. Studies that address the ecology of cryptic fish in the TEP have been carried out in tidepools and coral reefs in Mexico and Colombia (Castellanos-Galindo et al., 2005, 2014; Balart et al., 2006; González-Cabello and Bellwood, 2009; Alzate et al., 2014). The cryptobenthic fish fauna of the TEP has a low species richness compared to other areas, which produces low functional redundancy among the species that make up these communities (González-Cabello and Bellwood, 2009). The above refers to the fact that few species play the same function in the ecosystem (e.g. feeding habits) (Loreau, 2004), so this function is vulnerable to disturbances. These characteristics make this community of organisms vulnerable to both anthropogenic and environmental impacts.
In the present work, the cryptobenthic fish community is documented for the first time in the marine protected area Málaga National Natural Park Bahía Málaga (Uramba NNP). It is also evaluated whether the structure of this community differs temporally and spatially in this place.
STUDY AREA
The Uramba NNP (3,953169 N-77,326456 W; Figure 1) is a marine protected area (MPA) located on the central coast of the Colombian Pacific. This bay was formed during tectonic events in the late Miocene-Holocene (Martínez and López-Ramos, 2011). It has an area of 470.94 km2. Estuarine conditions dominate in this area that has average depths of 12-15 m. Also, it hosts a diversity of habitats that include mangroves, rocky coasts, mudflats, sandy beaches, and both soft and hard bottoms (Cantera-Kintz et al., 2013). Annual precipitation is one of the highest among the coastal areas of the Western Hemisphere, with an average of 7236 ± 2033 mm between 1969 and 2015 (station n.° 5407003-IDEAM), with a period of lower rainfall between January and April (x̃ = 422 mm/month) and higher rainfall between May and December (x̃ = 746 mm/month) (Castellanos-Galindo and Krumme, 2013). Sea surface temperature is stable during the year (27-30 °C), while salinity values vary between 0 and 30 depending on tidal currents and precipitation (Cantera-Kintz et al., 1998). This area is characterized by presenting a semi-diurnal macro-tidal regime (ca. 4 m tidal range).
MATERIALS AND METHODS
Sampling
Hard artificial substrates (SADs) were used as the sampling unit, which contained bricks and fragments of shells collected in the area (shells). These materials were deposited in plastic baskets (35 × 20 × 18 cm) and covered with a mesh with 2 mm openings (Figure 2). Six localities were chosen along the bay as follows: two in the inner zone (Silver: IN1, Coquero: IN2), two in the middle (Curichichi: MD1; The Negros: MD2) and two in the outer zone (Morro Chiquito Islet: OU1; The Negritos: OU2) (Figure 1). The rocky substrate is predominant at these localities and is surrounded by sandy-muddy bottoms. The depths at which the SADs were placed ranged between 3-7 m during low tide.
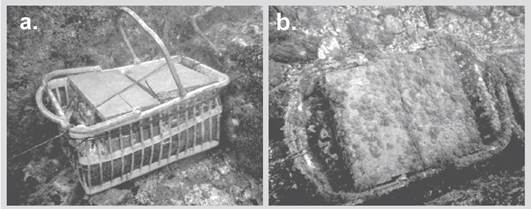
Figure 2 Hard artificial substrates (SADs) used for the collection of cryptobenthic fish in the six locations of the Uramba NNP. A) SAD recently exposed and B) SAD before being recovered, after five months.
The sampling was carried out between June 2008 and August 2009, a period in which seven field trips were carried out approximately every two months. In the first field trip, three SADs were located in each of the localities (3 SADs × 6 localities = 18 SADs). In the following field trips, a similar number of SADs were located in each location. The SADs were recovered after the third field trip when the first set of 18 SADs was retrieved. In the following field trips, the recovery and location of SADs continued. On average, the SADs were submerged for five months (x̃ ± s.d. = 145 ± 19.4 days). In total, 90 SADs were exposed and recovered throughout the sampling. Upon being recovered, each SAD was carefully lifted from the bottom and placed inside larger plastic baskets covered with a mesh with smaller openings while still in the water. Later they were slowly brought to the surface with a recovery bag to prevent some specimens from escaping during the process.
After being extracted from the water, the SADs were placed in plastic trays with seawater. Once on land, they were carefully disarmed, searching for of fish and invertebrates, scraping the substrate by hand, and sifting the sediments accumulated in each unit. All fish were identified to the lowest possible taxonomic level (following Robertson and Allen, 2008), total length (precision = 0.01 mm) and weight (precision = 0.001 g) were measured. Some fish were preserved in formalin (10 %) and transported to be deposited in the Ichthyology Reference Collection of the Universidad del Valle (CIRUV). The abundance data of the three SADs for each locality were grouped due to the low number of fish collected in each experimental unit. Fish density was calculated by considering the volume of each SAD (35 × 20 × 18 cm = 0.0126 m3 or 0.07 m2). Information on feeding habits (HA) was obtained for each species recorded in Robertson and Allen (2008) and Froese and Pauly (2009).
Data analyses
Non-metric multidimensional scaling (nMDS) was used to explore whether clustering patterns existed between locations. A permutational multivariate analysis (PERMANOVA) was used to statistically test whether the cryptobenthic fish community differed spatially (between zones) and temporally (between months). Also, a percentage similarity analysis (SIMPER) was used to identify the species responsible for the differences between zones and months when significant differences were found in the PERMANOVA analysis. All the analyzes were carried out after transforming of the abundance data to the square root (one month of sampling was excluded for the IN1 locality due to the absence of fish). In all cases, the Bray-Curtis similarity index was used. The analyzes were performed with the vegan package (Oksanen et al., 2019) of the R statistical program (R Core Team, 2019).
RESULTS
A total of 197 individuals belonging to 26 species and 11 fish families were collected during the entire sampling period. Of the species found, 50 % correspond to new records for the Uramba NNP (Figure 3, Table 1). Gobiidae, Labrisomidae, and Gobiesocidae dominated the community in number of species. Gobiidae (43.7 % of all individuals) was the most representative family in terms of abundance, followed by Labrisomidae (22.3 %) and Muraenidae (11.7 %). The families with the least representativeness in the samples (less than three species and few individuals) were Balistidae, Serranidae, Pomacentridae, and Antennariidae. The yellow stalk Starksia fulva was the most abundant species, representing 16 % of all individuals. The freckled worm goby Cerdale ionthas and the goby Gobulus hancocki accounted for 10 % and 15 % of the abundance, respectively. The remaining species presented abundances less than 10 % each.
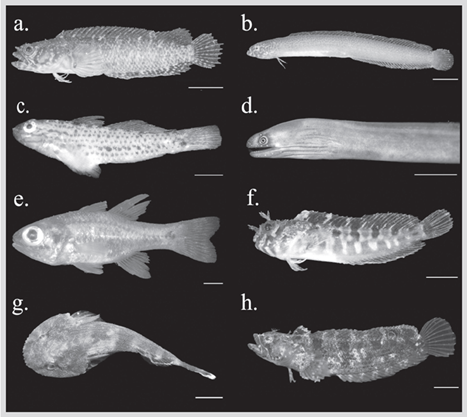
Figure 3 Some cryptobenthic fish from the Uramba NNP. A) Starksia fulva. B) Cerdale ionthas. C) Coryphopterus urospilus. D) Gymnothorax flavimarginatus. E) Apogon dovii. F) Hypsoblennius caulopus. G) Gobiesox adustus. H) Paraclinus mexicanus. Scale bar = 5 mm. Photographs: Arthur Anker.
Table 1 Cryptobenthic fishes collected in SADs (90) were exposed in the six locations of the Uramba NNP in the five sampling events (2008-2009). Total number of individuals for each species (n) together with the number of individuals measured (in parentheses). x̃ ± d.e. = Average ± standard deviation. HA = Feeding habits. C = Carnivore. P = Planktivorous. O = Omnivore (Robertson and Allen, 2008). FO: Frequency of occurrence. *New records for the Uramba NNP. The list is presented according to the classification proposed by Betancur et al. (2017).
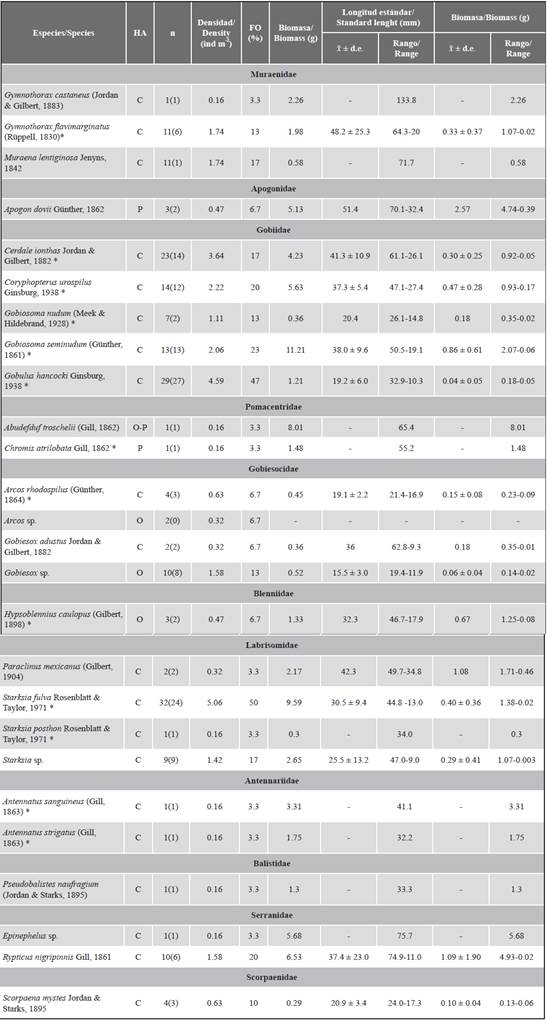
The average fish density was 31.2 ± 27.7 ind m-2. For S. fulva and G. hanckoki, it was 5 ind m-2, while species with a single individual presented densities of 0.16 ind m-2 (Table 1). The average abundance was higher for OU2 (12.8 ± 10.5 ind m-2) and lower for OU1 (2.6 ± 2.3 ind m-2) and the average richness observed was higher for OU2 (5.4 ± 2.7 ind m-2) and lower for IN1 (1.6 ± 1.1 ind m-2).
In the nMDS, the separation of IN1 and OU2 from the other localities (Figure 4) and from the internal zone of the other zones was observed. The two-way PERMANOVA analysis (zones and months) showed that only the zones presented significant differences, and there was no interaction between the factors (Table 2).
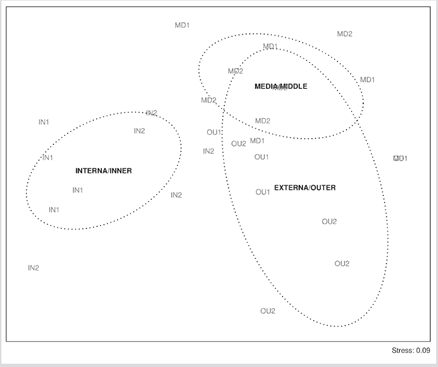
Figure 4 Non-metric multidimensional scaling (nMDS) of the cryptobenthic fish localities and sampling areas in the Uramba NNP (Tropical Eastern Pacific).
Table 2 Results of the two-way permutational multivariate analysis (Permanova ) evaluating the effects of the zones and the months in the cryptobenthic fish community in the Uramba NNP (Tropical Eastern Pacific). gl: Degrees of freedom. SC: Sum of squares. MC: Mean of squares. P: Level of significance. **: Significant (P < 0.05).
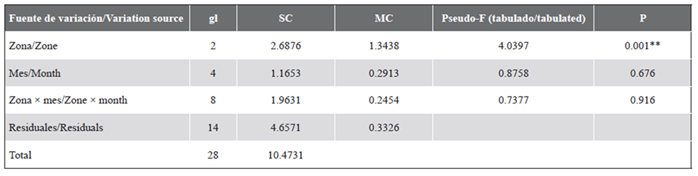
For the IN1 locality, the gobies Gobiosoma seminudum and C. ionthas accounted for 68.8 % and 31.2 % of the average Bray-Curtis similarities. For IN2 S. fulva was the most representative species, contributing 57.4 % of the average Bray-Curtis similarity. Gobulus hancocki was the species that contributed the most to MD1 with 65 % of the average similarity. Similarly, Coryphopterus urospilus was the most representative species for MD2 with a contribution of 41.6 % of the similarity of this locality. The differences found in the cryptobenthic fish community between the internal zone and the other two zones are due to C. ionthas, G. seminudum, S. fulva, and G. hancocki. Finally, the most representative species for the OU1 and OU2 localities of the external zone were S. fulva and the brunette Gymnothorax flavimarginatus, respectively. The mentioned species were responsible for the greatest contributions in the differences between the pairs of zones when evaluated utilizing the SIMPER analysis (Table 3).
DISCUSSION
The cryptobenthic fish community of the Uramba NNP presents similarities with the composition of fish families that have been found in the coral reefs of the Gulf of California (Balart et al., 2006; González-Cabello and Bellwood, 2009) and of the Panamanian Pacific (Enochs et al., 2011). In the Gulf of California, Tripterygiidae, Gobiidae, Chaenopsidae, and Labrisomidae are the families with the highest number of species, while Chaenopsidae, Tripterygiidae, and Gobiidae are those with the highest abundances. In the Uramba NNP, Gobiidae and Labrisomidae are the dominant families in the cryptobenthic fish community both in richness and abundance, while Tripterygiidae and Chaenopsidae were not recorded. The absence of these families can be explained by the fact that many of the three-finned fish species (Tripterygiidae) and tube blennies (Chaenopsidae) have specialized ecological niches not present in SADs. For example, species of Chaenopsidae such as the barnacle blenny Acanthemblemaria hancocki Myers and Reid, 1936, can be observed in shallow environments of the middle and inner areas of the bay. Similarly, individuals of the three-finned fish Axoclinus lucillae Fowler, 1944 (Tripterygiidae) are found in rocky tidepools of the outermost station (OU2) (unpublished information from the authors). Although there are no consolidated coral reefs in the Uramba NNP as in the Gulf of California or the Panamanian Pacific, the presence of similar fish communities indicates that the occurrence of some rocky reefs in this protected area can serve as habitat for these species, as these reefs are an ecological equivalent to coral reefs, with a heterogeneous structure where organisms can find shelter, food and nursery areas. Also, most of the species found are highly tolerant to fluctuations in salinity typical of this locality in the Colombian Pacific.
On the Pacific coast of Panama, reef matrices have been simulated using skeletal remains of pocilloporid corals, in which Glynn (2006) found Gobulus hancocki among the most abundant species. However, the most common cryptic fish in this study was the black-spot reef-bass Pseudogramma thaumasia (Gilbert, 1900), which was not found in the Uramba NNP, probably because this species prefers hard substrates in low turbidity areas, a situation that is rare in this MPA.
From functional groups’ perspective, the cryptobenthic fish community of the Uramba NNP differs from that described for the Great Barrier Reef by Depczynski and Bellwood (2003). In Uramba NNP the cryptobenthic fish community is dominated by carnivores (ca. 70% of the species and 85% of the individuals found in this study). In contrast, in the Great Barrier Reef, both carnivores and detritivores are dominant. In the TEP, Glynn (2006) and González-Cabello and Bellwood (2009) found that carnivores and planktivores dominate, while detritivores are a minority component of this community. The differences in these trophic groups of cryptobenthic fish from these regions (Eastern Indo-Pacific and Tropical Eastern Pacific) may be due to the disparity in both areas’ geological and environmental histories.
Los Negritos, the outermost locality in this study (OU2), is the one with the least impacted due to the drainage of fresh water and terrigenous sediments. The predominant substrate there is rocky and usually presents less turbidity compared to the other areas. Furthermore, it is considered one of the most biodiverse areas (Cantera-Kintz, 1991; Guevara-Fletcher et al., 2011) of the Uramba NNP. The composition of families in Los Negritos is similar to that observed in the tidalpools of the Gorgona National Natural Park (Castellanos-Galindo et al., 2014), where coral reefs can be found adjacent to the intertidal zone. Muraenidae is a dominant family in the tidalpools in Gorgona, with six species that contribute significantly to the fish densities and biomass of these coastal environments. In NPP Uramba, G. flavimarginatus (Muraenidae) was one of the species that differentiated OU2 from the other localities in this protected area. Similarly, species of Gobiesocidae and Antennariidae were represented, being exclusive to this sampling station.
In contrast, IN1 was dominated by C. ionthas and E. seminudus and was characterized by the lowest species richness among all localities. These species are commonly observed in mudflats in mangrove areas close to the sampling location, where they play an essential role as predators of the food web of mangrove ecosystems. This was evidenced using stable isotopes (Medina-Contreras et al., 2018). The location of IN1 in the main channel of the Uramba NNP is characterized by strong tidal currents and by a greater influence of estuarine conditions, where the presence of terrigenous sediments is high and limits the settlement of cryptobenthic fishes. Concerning the above, it is observed that OU2 and IN1 have the greatest environmental and species composition differences. However, species such as S. fulva and G. hancocki are found in most localities, suggesting that they are tolerant to the dynamic conditions typical of estuarine areas.
The proportion of new records for the study area indicates that SADs are an adequate tool for collecting organisms such as fishes that are not easily observed in visual censuses, and they also highlight the importance of the Uramba NNP as a biodiverse area. In the last fish species checklist in this area, 237 were recorded (Castellanos-Galindo et al., 2006), these new records increase the total number of species diversity for the park by 5 % (reaching 250 species). It is very likely that when sampling in other habitats of the Uramba NNP (e.g. mangroves) using alternative sampling methods that focus on the species that make up these cryptobenthic communities, the number of fish species registered in this protected area will increase. Preliminary work that has focused on the mangrove endofauna in different localities of the central Colombian Pacific coast has revealed that the diversity of both fish and other organisms such as crustaceans are more significant than what has traditionally been believed (J.F. Lazarus, pers. obs.) and have allowed finding new species for science (Anker and Lazarus, 2015a, 2015b, 2017). This opens a new line of research in which the diversity of these systems can be known. The contribution of this component of fauna to the food webs of estuarine ecosystems can be evaluated.
On the other hand, larger demersal fish could have escaped from the SADs during the recovery process, but most cryptobenthic species are probably represented by this sampling method. Glynn (2006) found in Panama that the number of common fish species between visual censuses and artificial substrates is low. Some studies in the Great Barrier Reef and off the coast of New Zealand have also shown a little overlap between visual censuses and surveys in which rotenone has been used (Ackerman and Bellwood, 2000; Willis, 2001; Smith-Vaniz et al., 2006). Structures similar to SADs have been used frequently to investigate the settlement patterns of fish in various of natural habitats and at different time scales (e.g. Lozano and Zapata, 2003; Mellin and Pontón, 2009). Zimmerman and Martin (2004) used a similar method that made it possible to collect reef invertebrates in the Caribbean effectively. These results allow demonstrating that the use of SADs is an economical, non-invasive, and non-destructive alternative that allows having a representative sample of cryptobenthic fauna, which facilitates the study of different ecological processes that occur in benthic communities. However, care must be taken when extrapolating the fish densities of the SADs to the fish densities observed in nature. This method can constitute an aggregation site for individuals in the natural environment dispersed due to the limited availability of suitable substrates.
The density of cryptobenthic fishes observed in the Uramba NNP (31.2 ind m-2) is in the upper range compared to other fish communities studied with different sampling techniques (e.g. rotenone, visual censuses) in other regions of the world. For example, Depczynski and Bellwood (2003) used rotenone to study cryptobenthic fish in the Great Barrier Reef, finding densities of 11.43 ind m-2 (384 individuals in 33.6 m2). A similar situation occurred with González-Cabello and Bellwood (2009), who found that the density of cryptobenthic fish in the Gulf of California was 20.9 ind m-2. Therefore, it can be considered that the use of SADs is a simple and reliable method to carry out studies on this group of organisms.
The present study is one of the first attempts to describe the structure of cryptobenthic fish communities in estuarine areas in the Tropical Eastern Pacific. Preliminary observations on the abundance of these species and the short life cycles of many of them indicate that they play an essential role in the ecosystems’ trophic dynamics where they are found (Brandl et al., 2019). Therefore, the trophic interactions with other components of the ecosystems present in the Uramba NNP deserve to be investigated in-depth (e.g. metabarcoding of stomach content; Casey et al., 2019). In particular, the presence of a high number of carnivores in the SADs indicates that these heterogeneous benthic substrates in the Uramba NNP also provide a habitat for a great variety of prey (invertebrates) on which fishes can feed.
The low potential of functional redundancy, the high endemism, and the increase in the frequency of ENSO events and their ecological impacts are the main causes why some marine taxa in the TEP are threatened with extinction (Polidoro et al., 2012; Chasqui et al., 2017). Therefore, it is necessary to understand how this community of cryptobenthic fishes reacts spatially and temporally to changes in environmental conditions that occur in the coastal habitats of the region (e.g. pollution and infrastructure development). Comparing this group’s response with others with greater functional redundancy (e.g. crustaceans, mollusks) could help understand the main mechanisms that keep marine ecosystems’ function. Cryptobenthic fishes could then be used as a model to test how different anthropogenic and natural disturbances (e.g. ENSO) affect the functioning and resilience of ecosystems in this MPA. This study shows that the Uramba NNP is vital for the conservation of little-known marine fauna in Colombia.