INTRODUCTION
Coral reefs face acute deterioration worldwide as a result of the interaction between global stress factors associated to climate change (Hoegh-Guldberg et al., 2007; Ainsworth et al., 2016) and local and regional factors (Zaneveld et al., 2016).This deterioration process threatens the many goods and services derived from tourism, fishing, and coastal protection provided by this ecosystem to coastal communities (Spalding et al., 2017). Reef degradation is particularly critical in the Caribbean, where about half of the live coral cover has already been lost and there is a shift from coral to algae dominance in several reefs (Mumby et al., 2007; Jackson et al., 2014).
Water quality degradation due to anthropogenic perturbations can have severe consequences on coral reefs, negatively affecting trophic interactions, coral cover, and the susceptibility of corals to diseases and massive bleaching events (Rogers, 1990; Koop et al., 2001; Sutherland et al., 2010; Wagner et al., 2010; Fabricius, 2011; Junjie et al., 2014; Pollock et al., 2014; Shantz and Burkepile, 2014; Vega Thurber et al., 2014). Most studies aimed at better understanding the consequences of water quality degradation on corals have focused on the effects of nutrient enrichment, sedimentation, and particle abrasion. The effects on the underwater light environment and its relationship with coral physiology and ecology have been less explored in comparison (Yentsch et al., 2002; Anthony et al., 2004; Canto et al., 2021; López-Londoño et al., 2021). Considering the essential role of light as the main energetic resource for symbiotic corals, offsetting this knowledge gap is fundamental to better understand and predict how coral reefs will respond to future conditions associated to climate change and coastal development.
Coral reefs are exposed to a broad range of light conditions, from very high intensities close to the water surface (~40 mol quanta m-2 day-1, with irradiance peaks close to 1500 μmol quanta m-2 s-1 at noon) to low-light conditions with increasing depth (~1 mol quanta m-2 day-1, with irradiance peaks close to 50 μmol quanta m-2 s-1). As light penetrates into the water column, it is absorbed and dispersed by the water itself and by dissolved organic material and suspended particles, thereby reducing its intensity and changing its spectral composition (Kirk, 2011). The light that reaches the sea floor determines the primary productivity rates of benthic communities, as well as coral growth and calcification, playing an essential role in the energy balance of such communities (Falkowski et al., 1984; Gattuso et al., 2006; Colombo-Pallotta et al., 2010). In addition, light availability, together with local temperature and hydrodynamic conditions, is a major driving force of biodiversity patterns and the structure of coral communities (Kahng et al., 2019; López-Londoño et al., 2022). In this way, the amount of available light, mediated by the water optical properties and depth, is a fundamental factor in symbiotic coral ecology and physiology.
The Varadero Reef is located in the southern end of the Caribbean Sea, close to the southern mouth of the Cartagena Bay in Colombia. This reef is exposed to the turbid plume of Canal del Dique, an artificial arm of the Magdalena River that discharges large amounts of freshwater with high sediment load into the Cartagena Bay (Restrepo et al., 2018; Tosic et al., 2019). The canal-caused turbidity limits light penetration into the water column, conditioning the reef to a low-light environment (López-Londoño et al., 2021). Despite their proximity to the city of Cartagena and the Dique plume influence, some portions of the reef are in good condition in terms of coral cover, mostly toward shallow areas (Pizarro et al., 2017). This study sought to answer the following research questions: 1) Are the survival rates of corals from Varadero different from those of corals from clear water sites? 2) Can corals originating in Varadero acclimate to clear-water conditions, and can corals originating in clear-water sites acclimate to the turbid conditions of Varadero? and 3) How is the temporal variation in physiological responses of corals from Varadero relative to that of corals from clear-water reefs? In previous studies, the structure and composition of the coral community in Varadero has been described (Pizarro et al., 2017), as well as the effects of local conditions associated with the Canal del Dique continental discharges on coral microbiomes (Roitman et al., 2020) and coral physiology and ecology (López-Londoño et al., 2021). By answering the questions raised above, this study seeks to complement and deepen the current knowledge on the Varadero Reef coral community as an input for prioritizing management and conservation strategies for this reef and other marginal reefs exposed to similar conditions.
MATERIALS AND METHODS
Experimental design
To assess the effect of the Canal del Dique turbid plume on the survival and physiological responses of hermatypic corals (i.e., reef-builders), a reciprocal transplant with fragments of the species Orbicella faveolata was conducted between two sites with different levels of exposure to the Dique plume. The Canal del Dique is a man-made distributary channel of the Magdalena River that discharges copious amounts of freshwater (~500 m3 s-1) with high sediment load (~24.000 t d-1) into the Cartagena Bay, which have respectively increased by 30 and 50 % over the last decades (Restrepo et al., 2016). The species O. faveolata was selected as indicator of the effect of perturbations produced by the plume on Varadero Reef corals due to the broad knowledge of physiological responses in this species (Rowan et al., 1997; Warner et al., 2006; Colombo-Pallotta et al., 2010; Kemp et al., 2015; Scheufen et al., 2017a, 2017b), its wide distribution and dominance in Caribbean reefs following consistent vertical zonation patterns (Weil and Knowlton, 1994; Pandolfi and Budd, 2008), and the possibility of measuring reliable photoacclimation responses due to its flat morphology at meso-scale, which prevents the formation of internal light gradients.
The Varadero (VAR) site is within the reef of the same name, which has an approximate area of 1 km2 (López-Victoria et al., 2015; Pizarro et al., 2017) and is located close to the Bocachica Strait toward the south of Cartagena Bay (10° 18’ 23.3” N, 75° 35’ 08.0” W) (Figure 1). In general, the reef is highly turbid due to its closeness (6 km to the east) to the Canal del Dique mouth in the Cartagena Bay and the direct influence of its plume, whose suspended solids are mainly composed of silts and clays (Lonin et al., 2004). Coral cover in Varadero Reef is low below 12 m (1-5 %). Above this depth, massive colonies of some species (e.g., Orbicella faveolata) gradually become more abundant, reaching maximum coral cover between 3 and 8 m. The shallowest portion of the reef is in good conditions in terms of live coral cover (50-60 % at 6 m depth) (Pizarro et al., 2017).
The site Isla Grande Norte (IGN) is located 21 km southwest of Varadero in the National Natural Park Corales del Rosario y San Bernardo (NNPCRSB) (10° 11’ 12.1” N, 75° 44’ 43.0” W), within a network of reticulated reefs that encompasses one of the most developed coral reef areas in Colombia (Díaz et al., 2000). The water in the vicinity of IGN is predominantly clear due to a lower influence of the Dique plume when compared to VAR (Figure 1). However, IGN, as the rest of the reefs within the NNPCRSB, is occasionally subjected to chronic stress by the Dique plume, with a concentration of suspended particles that can exceed 10 mg cm-2 d-1 (reference or threshold value for healthy reef waters) (Rogers, 1990; Henao-Castro, 2013; Restrepo et al., 2016). The coral reef area where IGN is located contains terraces of karstic origin with partially emerged portions and exuberant coral growth, with massive colonies dominating in shallow waters and encrusting or crustose morphologies dominating below 12 m. It is worth mentioning that live coral cover in this coral reef area has drastically decreased over the last decades due to natural and anthropic disturbances, including the reduction of water quality associated to the Canal del Dique continental discharges (Díaz et al., 2000; Restrepo and Alvarado, 2011; Restrepo et al., 2016).
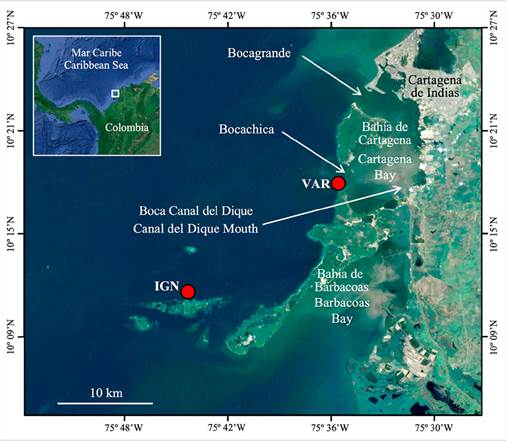
Figure 1 Study area indicating the location of the two transplant sites (red circles). Modified from: Google, Maxar Technologies.
The depths selected for the reciprocal transplantation were 3.5 m in VAR and 12 m in IGN. Both sites were exposed to similar total irradiance as a result of the local optical properties of the water column. Depths were selected from preliminary analyses of the vertical attenuation coefficient for diffuse downwelling irradiance (K d ) at each site, which were obtained after measuring the light intensity variation across the depth gradient using the cosine-corrected PAR sensor of a submersible fluorometer (Diving PAM, Walz). The K d results of the combined effects of light absorption and dispersion associated with the concentration of dissolved organic material and suspended particles, being a good indicator of the water optical properties at each site (Kirk, 2011).
In October 2016, three fragments of approximately 10 cm2 were collected from the free edges of 15 healthy colonies of the species O. faveolata (N = 45) at each site. The donating colonies were randomly selected at a constant depth in each site (3.5 m in VAR and 12 m in IGN). Fragments were glued with non-toxic epoxy (Z-Spar A-788 epoxy) to removable PVC couplings (Figure 2a), which were inserted in PVC pipes anchored to a cement block located at the same depth of the donating colonies (Figure 2b). After a two-week recovery and acclimating period with regard to the local conditions, an even number of fragments (n = 15) was transplanted from its site of origin to the turbid (VAR) and clear-water (IGN) site (i.e., VAR→VAR, VAR→IGN, IGN→IGN, IGN→VAR). A series of physiological analyses were conducted before (T1), and seven months after transplantation, in May 2017 (T2). To conduct these analyses, a variable number of samples was obtained from 15 fragments in T1 and from the surviving fragments in T2 at each site (Table 1). The fragments were visually inspected in T2 to quantify the survival rate of corals at each site.
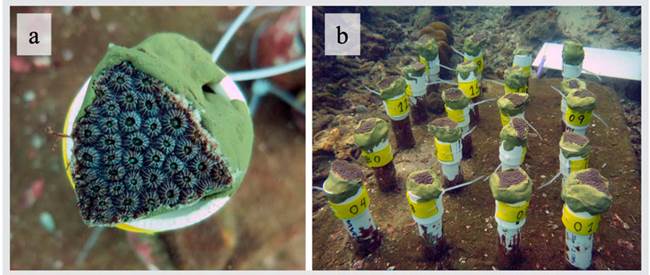
Figure 2 Coral fragments used in the transplant experiment. a) Fragment attached to a removable PVC coupling. b) Cement block where couplings with coral fragments were anchored (several blocks were used to place the 45 fragments initially obtained at each site).
Environmental variables
During the transplant experiment, the temperature was monitored every 30 min with automatic temperature loggers (Hobo UA-002-64, Onset Computer Corporation), and the light intensity every 10 min with cosine-corrected light sensors (Odyssey PAR, Dataflow Systems). Light sensors were previously calibrated against a reference cosine-corrected light sensor (LI-1400, Li-COR). Replicates of K d were obtained both in T1 and T2 at VAR (n = 26 and n =23) and at IGN (n = 13 and n = 14, respectively).
Optical and structural properties of the coral tissue
The chlorophyll a (Chla) content in corals was spectrophotometrically measured with a modular spectrometer (Flame-T-UV-VIS, Ocean Optics Inc.), using Jeffrey and Humphrey (1975) equations. Pigments were extracted using a mixture of acetone / dimethyl sulfoxide (95:5 vol / vol) (Iglesias-Prieto and Trench, 1994), from samples of coral tissue previously extracted with an air gun connected to a scuba tank and homogenized with a tissue homogenizer (Tissue-tearor, BioSpec Inc.).
The in vivo light absorption capacity of the coral tissue was measured using a modular spectrophotometer (Flame-T-UV-VIS, Ocean Optics Inc.), following Enríquez et al. (2005) and Vásquez-Elizondo et al. (2017). The portion of the light spectrum that was reflected (Reflectance, R), was used to calculate the fraction of incident light absorbed by the coral tissue (Absorptance, A), according to the equation A= 1-R. This, while assuming that the light transmitted through the coral skeleton tends to zero. The Chla specific absorption coefficient (a Chla *) was used to characterize the light-absorption efficiency of in vivo photosynthetic pigments. This parameter was calculated according to Enríquez et al. (2005), using the equation a Chla * = (D 675 / ρ) * ln10, where D 675 is the estimated absorbance value of the coral tissue at 675 nm, corresponding to the absorption peak of Chla; and ρ is the pigment content per projected coral tissue area (mg Chla m-2).
Coral photosynthetic properties
Photosynthetic parameters were obtained from photosynthesis vs. irradiance curves (PE curves). PE curves were determined in laboratory-controlled conditions using an acrylic chamber with four hermetic compartments of ~600 ml each, equipped with highly sensitive fiber-optical oxygen sensors (FireSting, Pyroscience GmbH). Orbicella faveolata corals from each site and sampling time were incrementally exposed to ten levels of irradiance between 0 and ~1400 μmol quanta m-2 s-1 at 10 min intervals, previously measured with a Diving-PAM light sensor. Light was provided with four 16 W dimmable LED lamps (UL PAR38, LED Wholesalers Inc.) controlled in continuous mode with a custom-made software that allowed to modulate time and intensity of light exposure. Previously filtered sea water (0.45 μm) used in the incubations was maintained under constant agitation with magnetic stirrers and at a constant temperature of 28 °C with a thermocirculator (Isotemp, Fisher Scientific) connected to the acrylic chamber. The respiration rate (R d) was calculated in darkness from the mean oxygen consumption before and after light exposure during the PE curves. The maximum photosynthesis (P max) was calculated from the average O2 production at light intensities exceeding the saturation point (E k). The photosynthetic efficiency (α) was calculated from the slope of the linear increase in photosynthesis at sub-saturating light intensities. The compensation point (E c) was the light intensity where gross photosynthesis matched respiration (Osinga et al., 2012). The photosynthetic parameters were normalized per unit area, which was calculated with the aluminium foil technique (Marsh, 1970).
Chla florescence measurements were performed in situ on low-cloudiness days, using an underwater pulse amplitude modulated fluorometer (diving-PAM). The photosystem II (PSII) effective photochemical efficiency (ΔF/F m’) was measured at noon during the time of peak irradiance, and the maximum photochemical efficiency of PSII (F v/F m) was recorded at dusk. The maximum excitation pressure over PSII (Q m) was calculated as Q m = 1 - [(ΔF/F m’) / (F v/F m)] (Iglesias-Prieto et al., 2004). Chla fluorescence measurements were performed only at the end of the transplant experiment on surviving O. faveolata corals at each site (T2).
Statistical analysis
To answer the question: Are the survival rates of corals from Varadero different from those of corals from clear-water sites?, the survival rates of O. faveolata were evaluated at the end of the experiment at each transplant site, considering the place of origin of the coral fragments. In addition, to standardize the survival advantage of native corals at a specific site over corals from the opposite site, the differences in survival were divided by the average survival at each transplant site (Hereford, 2009). For example, to compare the survival of native corals of VAR against native corals of IGN transplanted to IGN, the proportion of survivors native to VAR was subtracted from the proportion of survivors native to IGN, and the result was divided by the average survival of survival of corals originating from both sites transplanted to IGN.
To answer the question: Can corals originating in Varadero acclimate to clear-water conditions, and can corals originating in clear-water sites acclimate to the turbid conditions of Varadero?, only physiological data obtained at the end of the transplant experiment (T2) were used, considering the site of origin of coral fragments at each transplant site. All data were tested for normality (Shapiro-Wilks test) and homogeneity of variance (Levene test) with a significance level (alpha) of 0.05 in order to determine the type of inferential statistics of each variable (Sheskin, 2003). For data sets that did not meet the assumptions of parametric statistics (Chla, a Chla *, Pmax, F v/F m, ∆F/F m’, Q m), the non-parametric Kruskal-Wallis was used to evaluate the effect of the site of origin and destination. For data sets that meet the assumptions of parametric statistics (A, α, E c, E k, R d), a two-way analysis of variance (ANOVA2) was used (Sheskin, 2003).
To answer the question How is the temporal variation in physiological responses of corals from Varadero relative to that of corals from clear-water reefs?, the physiological data obtained from native corals of each site at the beginning (T1) and at the end (T2) of the transplant experiment were used. Similar to the statistical procedure to answer the previous question, a non-parametric Kruskal-Wallis test was used in datasets that did not meet the assumptions (Chla and a Chla *), as well as an ANOVA2 in datasets that met the assumptions of parametric statistics (A, α, E c, E k, R d, Pmax).
K ds obtained at each site and sampling time were compared using the non-parametric Kruskal-Wallis test. Between-sites differences in mean daily temperature and daily integrated irradiance were analysed using the Mann-Whitney non-parametric test, considering that these variables did not meet the assumptions of parametric statistics (Sheskin, 2003). Statistical analyses were conducted using the R programming environment, version 3.6.1 (R Core Team, 2015).
RESULTS
Environmental variables
The vertical attenuation coefficient for diffuse downwelling irradiance (K d) varied between 0.26 and 0.80 m-1 in VAR and between 0.15 and 0.20 m-1 in IGN. The estimated K ds were significantly higher in VAR (H(1) = 51.55, p < 0.001), where a significant K d increase was also registered in T2 (0.51 ± 0.13 m-1, average ± SD) relative to T1 (0.35 ± 0.08 m-1) (H(1) = 5.59, p = 0.02). In IGN, the K ds were constant between sampling times (0.17 ± 0.01 m-1 in T1 and T2) (H(1) = 1.85, p = 0.174). A strong stratification of the water column was detected in the two sampling times at VAR, characterized by an increased attenuation of incident light produced by a highly turbid surface water layer of ~1m. It was estimated that this surface layer attenuates up to 90 % of the incident light (Figure 3). The daily integrated irradiance was slightly lower and more temporally variable in VAR (2.03 ± 1.32 mol quanta m-2 d-1) than in IGN (2.17 ± 0.91 mol quanta m-2 d-1) (U = 40976, p < 0.001) (Figure 3b). The mean daily temperature was similar in VAR and IGN (28.77 ± 1.06 °C and 28.62 ± 0.79 °C, respectively) (U = 13244, p = 0.487).
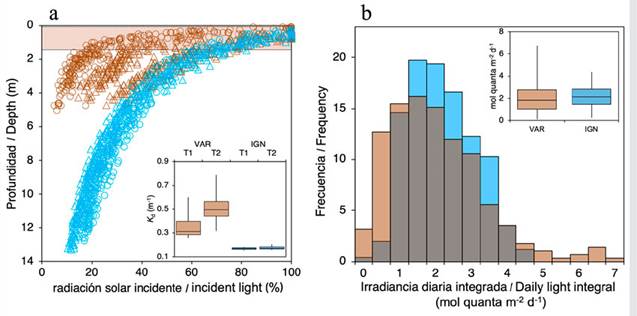
Figure 3 K d and irradiance variation in VAR (brown) and IGN (blue). a) Vertical light gradient measured on T1 (triangles) and T2 (circles), highlighting the strong attenuation produced by the superficial water layer in VAR (brown shading). The inset shows a boxplot with calculated K ds at each site and sampling time. b) Frequency histogram of the daily integrated irradiance. The inset shows a boxplot with the daily integrated irradiance values at each site during the transplant experiment.
Coral survival
Survival rates of both native corals and those originating from IGN were 100 % in VAR. The above suggests that native corals of VAR had no survival advantage with respect to corals originating from IGN. In IGN, the survival rate of native corals was 80 %, while the survival rate of corals originating from VAR was 60 % (Figure 4). It was estimated that, in IGN, native corals had a 29 % survival advantage with respect to corals originating in VAR.
Optical and structural properties of coral tissue
The chlorophyll content varied between 109.33 ± 26.96 and 195.27 ± 37.61 mg Chla m-2 in corals transplanted to VAR and between 91.36 ± 44.15 and 135.78 ± 51.04 mg Chla m-2 in corals transplanted to IGN (H(1) = 8.23, p = 0.004) (Table 1). Corals transplanted to VAR had higher chlorophyll content than corals transplanted to IGN (H(1) = 8.23, p = 0.004) (Figure 5). This difference even increased in T2 (H(1) = 5.29, p = 0.021) (Figure 6). No significant effect of the site of origin was observed on the chlorophyll content of corals at each transplant site (H(1)= 2.96, p = 0.085). The Chla specific absorption coefficient (a Chla *) varied between 0.013 ± 0.002 and 0.020 ± 0.002 m2 mg Chla -1, and between 0.019 ± 0.007 and 0.041 ± 0.016 m2 mg Chla -1 in corals transplanted to VAR and IGN, respectively (Table 1). Differences in a Chla * indicate that corals transplanted to IGN were more efficient at collecting light per unit of pigment than corals transplanted to VAR (H(1) = 8.22, p = 0.004) and that this difference was related to the site of origin of transplants (H(1) = 4.05, p = 0.044) (Figure 5). Additionally, the reduction of a Chla * in corals from both transplant sites detected in T2 (H(1) = 4.00, p = 0.045) (Figure 6) indicates that the light absorption efficiency was temporally variable. Light absorption in in vivo coral tissue, Absorptance (A), varied between 81 and 88 % in VAR and between 85 and 91 % in IGN (Table 1). No significant differences were observed in A between sites at the end of the transplant experiment (F(1, 29) = 1.34, p = 0.256) (Figure 5), nor between sampling times at each site (F(1, 26) = 0.14, p = 0.706).
Photosynthetic properties of transplanted corals
Photosynthetic parameters derived from PE curves showed similar variation among corals at both transplant sites (Figure 5), and no significant differences were detected in the photosynthetic efficiency, α (F(1, 22) = 0.08, p = 0.773), compensation point E c (F(1, 22) = 0.18, p = 0.672), saturation point E k (F(1, 22) = 0.15, p = 0.701), maximum photosynthesis P max (H(1) = 0.002, p = 0.956), and respiration rates R d (F(1, 22)= 0.04, p = 0.833). A significant increase in α (F(1, 24) = 7.38, p = 0.012) and R d (F(1, 27) = 20.59, p < 0.001) was observed in T2 relative to T1, as well as a decrease in E k (F(1, 24)= 46.58, p < 0.001) and P max (F(1, 27) = 5.94, p = 0.022) at both transplant sites (Figure 6). Some parameters derived from Chla fluorescence, which were only evaluated at the end of the transplant experiment, evidenced clear differences between sites (Figure 5). The maximum photochemical efficiency of PSII recorded at dusk (F v/F m) (H(1) = 24.15, p < 0.001) and the effective photochemical efficiency recorded at noon (∆F/F m’) (H(1) = 5.91, p = 0.015) were significantly higher in corals transplanted to IGN. The range of Q m variation was similar at both sites (H(1) = 1.27, p = 0.259) (Figure 5, Table 1).
DISCUSSION
Light attenuation by the Dique’s turbid plume
The strong stratification of the water column observed in Varadero, also recorded in previous studies (Pizarro et al., 2017; López-Londoño et al., 2021), results from the presence of two water masses with different properties (e.g., salinity and temperature), associated with the continuous flow of the freshwater plume from the Canal del Dique at the surface and a subsurface layer of denser oceanic waters (Lonin et al., 2004). Sediments from the Dique turbid plume, mainly silts and clays, tend to remain in the surface layer where turbulence is enough to keep them suspended (Lonin et al., 2004). Moreover, the presence of La Escollera (submerged breakwater) in the northern mouth of the Cartagena Bay (Bocagrande) acts as a wall that prevents the inflow of denser oceanic waters into the bay, favoring the continuous push of turbid surface water out of the bay (Molares y Mestres, 2012). The vertical stratification, together with continuous flow of the turbid surface layer, seems to favor the isolation of the Varadero Reef from the direct influence of Canal del Dique freshwater discharges. However, such stratification has a critical impact on light energy availability for corals, as only the turbid surface layer attenuates up to 90 % of the incident solar radiation. Although the K d in IGN did not show an important temporal variation, it was remarkably superior to the K d ’s recorded in other clear-water reefs (e.g., 0.06 in Curaçao: Vermeij and Bak, 2002), indicating a greater degree of light attenuation in the water column, potentially associated with the Canal del Dique freshwater discharges. The above highlights the potential influence of the Dique turbid plume extending to a considerable distance from its mouth, a condition that can be related to the generalized degradation of coral reefs within the NNPCRSB (Restrepo et al., 2006; Restrepo and Alvarado, 2011).
Varadero Reef conditions favor coral survival in shallow waters
Although environmental conditions in Varadero are typically considered sub-optimal for coral development (e.g., high turbidity, little light availability, and high sedimentation) (Kleypas et al., 1999; Weber et al., 2012), coral fragments of the indicator species O. faveolata transplanted to this site evidenced the lowest mortality (0 %), regardless of the site of origin. This result suggests that the higher survival of corals in VAR relative to that of corals transplanted to IGN does not appear to be related with any adaptive traits of Varadero-native corals, but to local environmental conditions. The high survival of corals in VAR may result from the interaction of two factors: 1) reduction of light stress over the photosynthetic machinery of the symbiotic algae (zooxanthellae) as a result of the light attenuating effect of the Dique turbid plume, which can lead to an increase in the amount of photosynthetic usable energy available for coral hosts in shallow environments (Fisher et al., 2019; López-Londoño et al., 2022); and 2) access to nutrients and energy through heterotrophy, compensating the reduced light availability and autotrophic potential (Anthony and Fabricius, 2000; Hoogenboom et al., 2010; Fabricius, 2011).
The higher coral mortality rates at IGN, which is consistent with the generalized degradation of coral reefs in the NNPCRSB (Díaz et al., 2000; Restrepo and Alvarado, 2011; Restrepo et al., 2016), may be related to a reduced availability of organic material that could be used by the corals as a source of nutrition in order to compensate for the low photosynthesis (Anthony and Fabricius, 2000; Hoogenboom et al., 2010), making them more susceptible to natural and anthropogenic disturbances prevalent in this area (Restrepo and Alvarado, 2011). Differences in hydrodynamic processes linked to the Dique plume between transplant sites could have also influenced the observed mortality rates. As the plume moves away from the Canal mouth, it reduces its speed, which may favor the deposition of sediments and flocs on corals from IGN. The energy required for actively removing such sediments may have been an additional stress factor associated with the coral mortality observed at this site (Anthony and Fabricius, 2000; Junjie et al., 2014).
It has been proposed that the potential benefit of light stress reduction may be responsible for a lower incidence in coral bleaching in turbid reefs during thermal-stress events (van Woesik et al., 2012; Morgan et al., 2017). However, it is necessary to highlight that this potential benefit is limited to the shallower portion of the reef and that, with increasing depth, the lack of energy derived from photosynthesis, added to the energy demands for active sediment rejection (Anthony and Fabricius, 2000; Junjie et al., 2014), may negatively affect the energy balance and survival of corals. Although this study did not assess coral survival rates across depths, previous studies have demonstrated an increase in the partial mortality of coral colonies with increasing depth in Varadero, potentially associated with the acute limitation of light and photosynthetically-derived energy (López-Londoño et al., 2021). Available evidence indicates that a potential beneficial effect of the turbid plume of Dique on Varadero Reef corals is limited only to the shallowest portion of the reef. Moreover, given the intrinsic relationship between light intensity and coral calcification (Colombo-Pallotta et al., 2010), the decreased light availability due to the attenuation effect of the Dique turbid plume potentially impairs the ability of reef-building corals such as O. faveolata to secrete calcium carbonate at a rate sufficient to maintain reef growth and structural complexity in the long term.
Native and foreign corals acclimate in a similar way to local conditions in Varadero
The physiological assessments indicated that corals develop phenotypes acclimated to low light, similar in both sites despite the differences in depth. This highlights the importance of considering the local optical properties of the water column -not only depth- in acclimation and physiological studies involving corals. Comparative analyses of photosynthetic parameters derived from PE curves, which are strongly affected by local environmental factors (mainly light and temperature), showed that these parameters behave similarly at both sites, thereby indicating that corals had similar photosynthetic potential. The maximum excitation pressure over PSII (Q m ) indicated that most of PSII reaction centers of symbiont algae in corals at both sites remain open (i.e., values close to 0), even at noon, when corals are exposed to daily peaks in irradiance (Iglesias-Prieto et al., 2004).
This suggests that the photosynthetic rates of algal symbionts are light-limited, and that the energetic contribution derived from photosynthesis to coral metabolism is low at both transplant sites. The values of F v/F m, however, indicated that coral symbionts at IGN have a higher efficiency to convert sunlight into chemical energy, and that corals from VAR have an increased fraction of photo-inactive PSII reaction centers and greater capacity to dissipate the excessive energy absorbed by the photosynthetic apparatus (Hoegh-Guldberg and Jones, 1999; Warner et al., 2002). Such differences can be the result of the occasional exposure to high irradiance in VAR, although previous studies have also suggested that such events are not long enough to induce important changes in the PSII activity (López-Londoño et al., 2021).
Coral chlorophyll content was one of the physiological parameters that had higher variation between sites. Corals from VAR had more than twice the photosynthetic pigment content than corals from IGN, which inevitably led to important reductions in light absorption efficiency (a Chla *) due to pigment self-shading (Scheufen et al., 2017a). These results indicate that corals from VAR are less efficient at absorbing light per unit of pigment than corals from IGN. It can be speculated that the ample nutrient in the Cartagena Bay, as a result of Dique discharges (Tosic et al., 2019), extends into Varadero and affects the metabolism of corals within this reef. It is well known that nutrient enrichment can affect symbiotic coral metabolism, leading to an increase in symbiotic algae density and/or cellular pigment content (Dubinsky et al., 1990; Shantz y Burkepile, 2014), conditions that, in turn, can affect the coral holobiont photosynthetic performance.
The absence of important differences in the photosynthetic parameters derived from PE curves indicate that corals at both sites have similar photosynthetic potential, and that the solar energy absorbed is used with similar efficiency in algal photosynthesis. The above suggests that corals from both VAR and IGN have a similar acclimation potential to prevailing environmental conditions at each site, and that the potential nutrient enrichment associated with Dique discharges in Varadero does not appear to have a negative impact on coral physiology. It is important to highlight that this study was conducted by comparing corals from two different depths at VAR and IGN, where total light exposure was similar due to the local optical properties of the water. Coral acclimation potential and survival are expected to change when comparing sites with contrasting sunlight exposure. For example, a transplant from VAR to IGN at a same depth of 3 m, could expose corals to acute light stress, considering that light intensity in IGN is expected to be about three times that of VAR at this depth, potentially affecting the acclimation capacity and survival of coral transplants. The above highlights the importance of considering local water optical properties and light climate in experiments and projects involving coral transplantation.
The Dique plume’s influence on coral physiology is temporally variable
The results of this research indicate that the effect of the Dique turbid plume on the water optical properties in VAR is temporally variable, being stronger towards the end of the transplant experiment (May of 2017), when the highest K d values were recorded. In IGN, the K d s were consistently low during the two sampling periods, suggesting a reduced effect, although constant, of the Dique plume on local water optical properties. The temporal variation of the plume effect on the water properties in VAR may arise from changes in the plume hydrodynamics and the amount of Dique freshwater discharges into the Cartagena Bay, conditions that are mostly shaped by local and regional seasonal patterns (Lonin et al., 2004; Restrepo et al., 2006; Tosic et al., 2019). Our findings indicate that the temporal variation of chlorophyll content and light absorption efficiency in corals from Varadero, can be mostly attributed to the seasonal dynamics of the Dique plume, considering the significant effect of the transplant site in the statistical models. These physiological effects associated with the temporal dynamics of the plume seem to be driven by changes in the concentration of nutrients in the water column. The temporal variation of photosynthetic parameters in corals, on the other hand, can be mostly attributed to the seasonal variation of environmental conditions prevalent in the whole study area, considering that the pattern of variation of these parameters was similar at both transplant sites (there was a significant effect of the time variable on the statistical models).
CONCLUSIONS
The strong stratification of the water column in Varadero helps to isolate the reef from the direct influence of the turbid freshwater discharges from the Canal del Dique. The turbid surface layer has an extraordinary attenuating effect on the incident sunlight, limiting the amount of photosynthetic usable energy for reef-building corals such as O. faveolata. The high survival of corals in Varadero is likely to be related to the synergistic effect of light stress reduction and complementary nutrition through heterotrophy. However, such effect is restricted to the shallower portions of the reef. Differences in the Dique plume circulation patterns may be related to higher coral mortality in reef areas adjacent to Varadero. Corals in shallow areas in Varadero develop phenotypes acclimated to low light comparable to those of corals found at greater depths in clear-water reefs. Physiological parameters indicate strong light limitation and limited autotrophic potential, which represents a risk for coral survival with increasing depth and decreasing light availability. Chlorophyll content was the most sensitive coral structural trait influenced by the Dique discharges. The increase in the Dique freshwater discharges into the Cartagena Bay, together with maritime infrastructure works involving dredging operations, can further compromise the water quality, as well as the light energy availability for reef-building corals, and, ultimately, their capacity to maintain the Varadero reef. Effective policies seeking to improve the water optical properties should be a priority objective to preserve Varadero, as well as the ecosystem goods and services that benefit coastal communities.