INTRODUCTION
Chondracanthus chamissoi, or “yuyo”, is a red alga belonging to the phylum Rhodophyta, the taxonomic group with the largest number of species recorded in Peru (185 out of the 260 species in recent reports) (Ávila-Peltroche and Padilla-Vallejos, 2020). This seaweed is distributed from Piura, Paita, Peru (5° S), to Acnud, Chile (42° S) (Ramírez and Santelices, 1991; Calderón et al., 2010; Macchiavello et al., 2017). It grows while attached to hard substrate (Otaíza and Cáceres, 2015) in the inferior intertidal and subtidal zones down to 15 m deep (Bulboa and Macchiavello, 2006). In Peru, it is considered to be one of the most abundant red seaweeds in the littoral, and it is in high demand in local markets, mainly for direct human consumption and, to a lesser extent, for the extraction of carrageenans; these seaweeds can be commercialized in both fresh (4 %) and cured (96 %) states (Pariona and Gil-Kodaka, 2011; Castañeda et al., 2018; Espi et al., 2019; Ávila-Peltroche and Padilla-Vallejos, 2020; Basaure et al., 2021; Produce, 2021). It is worth indicating that the volumes of seaweeds landings have increased over the years, i.e., between 2010 (4368 ton) and 2021 (50480), which amounts to 12 times the landing volumes of the last 11 years (Produce, 2019, 2021).
Given its commercial importance, C. chamissoi has been studied for more than 20 years with the purpose of making its cultivation at sea feasible, wherein the advances made in vegetative propagation stand out (Macchiavello et al., 2003, 2017; Bulboa and Macchiavello, 2006; Pariona and Gil-Kodaka, 2011; Arbaiza, 2016; Colque, 2017; Zapata-Rojas et al., 2020; Basaure et al., 2021; Oyarzo et al., 2021). This cultivation technique involves the formation of new shoots from an initial inoculum or mother plant, which is achieved by fixating the seaweed to a natural and/or artificial substrate. This substrate is subsequently placed in either bottom or suspended marine cultivation systems (Sáez et al., 2008; Arbaiza, 2016; Basaure et al., 2021). One of the advantages of cultivation by vegetative propagation is that it can be performed throughout the year, as it does not depend on individuals with reproductive structures. This makes it a simple methodology that requires lower investments in comparison with spore-based cultivation (Macchiavello et al., 2012). One of its main strengths is also the production of biomass in short periods of time, in addition to the fact that it utilizes low-complexity techniques and technologies (Bulboa et al., 2013; Saez and Macchiavello, 2018; Basaure et al., 2021).
Among the disadvantages and/or difficulties of this form of cultivation is the fact that it requires abundant biomass from natural beds (Bulboa et al., 2013), considering the biomass losses caused by the presence of epiphytes and/or sediment in the samples collected (Arbaiza et al., 2016). These losses may entail the reduction of the initial inoculum, leading to a greater extraction of biomass from natural beds (Oyarzo et al., 2021). This, in combination with the fishing extraction of the resource, reduces the availability and productivity of beds over time (Flores et al., 2015).
In this context, several authors have pointed out that, in order to optimize the formation of secondary attachment discs (SADs) in a vegetative propagation culture, one must consider the previous treatment of the initial inoculum (cleaning with freshwater and elimination of epiphytes or sediment), as well as that of the water (filtration, coloration, and sterilization) and the substrate (formation or cleaning of the biofilm) (Icochea, 2008; Arbaiza, 2016; Colque, 2017; Macchiavello et al., 2017; Arbaiza et al., 2019; Suárez, 2019; Zapata-Rojas et al., 2020; Oyarzo et al., 2021). For productive purposes, the effect of these processes is unclear, and protocols related to water cleaning and treatment with chlorine are scarce. Therefore, this work aims to evaluate the formation of SADs as a function of substrate handling and inoculum and seawater treatment, with the purpose of contributing to the optimization of the initial stages of cultivation via vegetative propagation.
MATERIALS AND METHODS
Study area
C. chamissoi samples were collected at Mendieta beach, located in the Paracas National Reserve (Paracas district, Pisco province, department of Ica, Peru), on July 18th, 2020, by means of autonomous diving (14°3’38.54” S; 76 ° 15’ 54.72” O) (Figure 1). After collection, the seaweeds were transported in isolated thermal containers at 10 °C to Universidad Científica del Sur’s Laboratorio de Investigación en Cultivos Marinos (Licma), located in the district of San Andrés, Pisco province, department of Ica, Peru. Upon arriving at the laboratory, the algae in vegetative state were selected (individuals with no visible reproductive structures), as suggested by Macchiavello et al. (2012) .
Experimental design
Eight treatments (with four repetitions each) were employed, evaluating the substrate treatment (biologized and non-biologized), the previous algae treatment (washed and unwashed), and the culture medium (treated and untreated water) (Table 1).
Substrate treatment
Shells of Argopecten purpuratus (natural substrate) were used, which were sterilized with 1 % sodium hypochlorite for 10 min (Arbaiza, 2016) and subsequently processed in two different ways: a) biologized substrate: 16 shells were vertically submerged in 3.2 m3 incubation tanks with seawater for 15 days in order to promote the formation of biofilms (Figure 2). The water in the tanks was maintained at a temperature of 20 - 21 °C, a salinity of 35 - 36 ups, a photoperiod of 8:12 (light:darkness), and abundant aeration; b) non-biologized substrate: the 16 shells were stored in hermetic bags (Ziplock) directly after the disinfection process for later use.
Water treatment
Seawater was collected from San Andrés beach and conditioned at Licma at a temperature of 16 - 17 °C, a salinity 35 - 36 ups, a photoperiod of 8:16 (light:darkness), and a light intensity of 2300 lux. Afterwards, the water was treated in two different ways. a) The water was treated by adding sodium hypochlorite at a concentration of 2 % for every 1000 mL of seawater. It was then dechlorinated with sodium thiosulfate, using 1 mL for every 4 mL of Cl (Murray et al., 2018), from a mother solution of 25 % thiosulfate. b) The water was left untreated, and no procedures were carried out.
Algae treatment
In the laboratory, the algae samples underwent a mechanical cleaning process aimed at eliminating epiphytes or sediment residues adhered to the individuals’ structures, and they were then processed in two different ways: a) unwashed algae: the specimens were briefly washed with a 1 % sodium hypochlorite solution for 30 s, and then with freshwater for 1 min to eliminate chemical residues; b) unwashed algae: no procedure was carried out.
Fixating the algae to the substrate and incubation
The individuals in vegetative state were weighted in an analytical balance, adding 7 g of C. chamissoi (wet weight) to each experimental unit, considering the morphological characteristics described by Arbaiza et al. (2023). Next, they were attached the substrate (A. purpuratus shell) using a rubber band to promote contact between the seaweed and the surface, ensuring proper DFS generation. Then, the substrates with the fixated algae were placed in cultivation systems for 38 days (1 L flasks with 800 mL of seawater) and maintained under the following conditions: a temperature of 17 - 19 °C, a photoperiod of 12:12 (light:darkness, 2300 lux), constant aeration, and two water replacements on a weekly basis. In addition, a commercial foliar nutrient (Bayfolan ®) was added, at a ratio of 0.2 mL for every 1 L of seawater (Castañeda et al., 2018). Once the experimentation phase was complete, the inocula were removed from the shells using a scalpel, and the vegetative individuals were then weighted (wet weight). Finally, the total number of SADs per shell was determined by direct counting, employing a Leica EZ4E stereoscope. It is worth noting that only the SADs that were attached to the shells were counted, and higher magnification photos allowed confirming that no SAD was lost during the count while also allowing to observe the formation of these structures on the shells in detail (Figure 3).
Statistical analysis
Hypothesis tests were performed using a negative binomial regression model, as we were dealing with a count variable, in order to analyze the formation of secondary attachment discs. By means of a Shapiro-Wilk test, the data were found to exhibit a normal distribution (p>0.05) and variance homogeneity. The analysis of the information and the elaboration of graphs were carried out in the R4.2.0 software (copyright © 2022) and Excel 2016 (Microsoft, under license).
RESULTS
Through the statistical tests, it was evidenced that the SAD values are significantly different for unwashed and washed algae (p=0.03921), which is not the case for the substrate treatments and the water used as culture medium. However, interaction between the algae treatment and the substrate can be observed (p=0.03079) and between the substrate treatment and the water (p=0.01559). On the other hand, a deviation quotient of 1.406 over the degrees of freedom (24) was obtained, indicating a good model fit (Table 2).
Figure 4 shows that, depending on the treatment, the washed algae exhibited a greater formation of SADs in comparison with the treatments involving unwashed algae. The means of the unwashed algae were 0.584 ± 0.343, and that of the washed ones was 1.282 ± 0.382.
Figure 5 shows that there was interaction between the algae treatment and the substrate, wherein washed algae and biologized substrates exhibited similar means (1.903 ± 0.395) to those of unwashed algae and non-biologized substrates (1.313 ± 0.412). On the other hand, the interaction between washed algae and non-biologized substrates reported lower means (0.661 ± 0.519), similar to those of unwashed algae and biologized substrates (-0.144 ± 0.550).
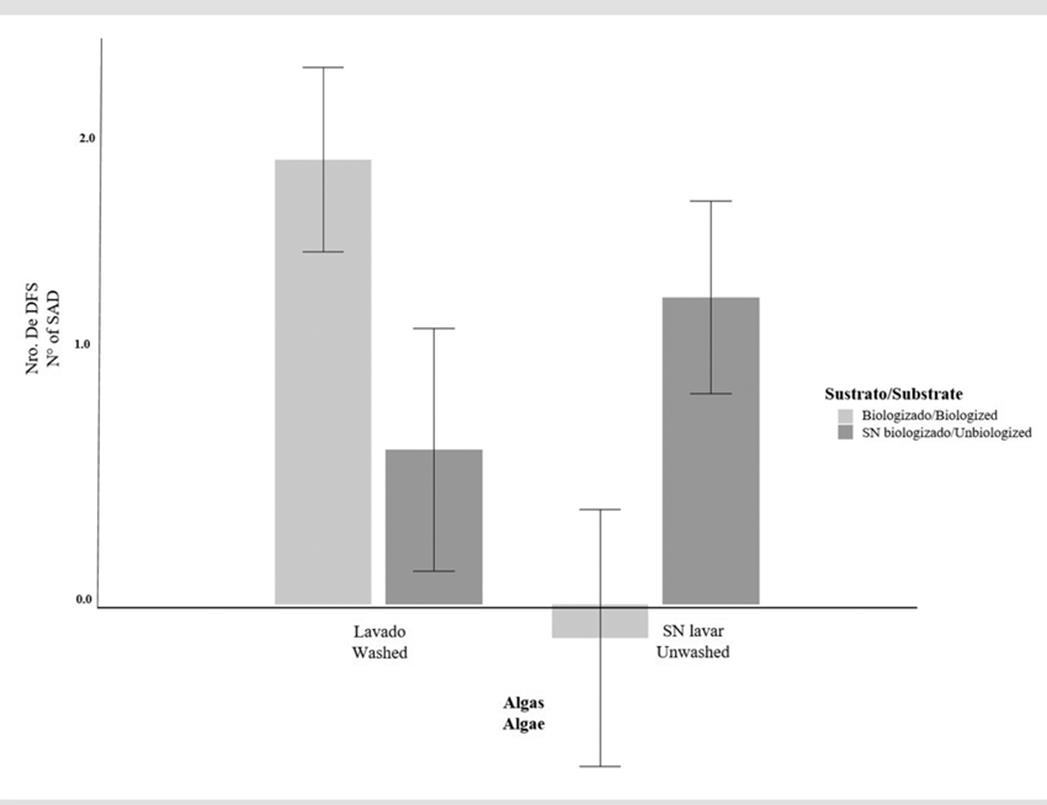
Figure 5 Number of SADs as a function of the interaction between the algae treatment (washed and unwashed) and the substrate (biologized and non-biologized)
Figure 6 shows the interaction between water treatment and substrate. Here, the chlorinated water treatment the non-biologized substrate exhibited similar means (1.597 ± 0.404) to those of unchlorinated water and the biologized substrate (1.354 ± 0.404). It can also be observed that the chlorinated water and the biologized substrate reported similar means (0.405 ± 0.524) to those of unchlorinated water and the non-biologized substrate (0.377 ± 0.525).
DISCUSSION
Chondracanthus chamissoi exhibits diverse reproduction strategies, such as spore-based propagation and reattachment by fragmentation of the thallus, allowing it to remain in its natural environment throughout the year (Acleto, 1986; Alveal, 2001; Vásquez and Vega, 2001; Riofrío, 2003; Fonck et al., 2008). Not only does SAD formation allow for the anchoring of drifting fronds of C. chamissoi, but it also plays an important role in the ability to propagate to new areas in the face of stressful environmental conditions for red algae cultivation (Pacheco-Ruiz et al., 2005; Sáez et al., 2008). In this study, all treatments formed SADs (1 - 21 SADs / shell) after 38 days of cultivation. These data are similar to those obtained by other researchers, who reported between 2 ± 1 and 20 ± 8; 1 and 27; and 32 ± 15 and 27 ± 7 SADs / shell over 20 - 40 days of cultivation (Bulboa et al., 2013; Arbaiza, 2016; Sáez and Macchiavello, 2018; Zapata-Rojas et al., 2020).
The results obtained indicate that algae cleaning process favor a greater SAD formation, as shown in Table 2 and Figure 4. This may be due to the fact that, with prior algae cleaning at the initial stages of cultivation, the sediments or epiphyte algae attached to C. chamissoi were eliminated, restricting competition for substrate and nutrients in the medium (Vásquez and Vega, 2001). These results agree with the previous procedures (washed algae) performed by Arbaiza (2016) for C. chamissoi. It can be stated that a greater amount of SADs can be obtained via prior algae cleaning.
On the other hand, in this study, the water treatment alone exhibited no significant differences. However, it could be observed that there was indeed interaction between water treatments and substrates, with slight differences. Previous research indicates that some bacteria present in the medium are involved in the production and degradation of algae phytohormones and biostimulants of cell growth and development for the formation of SADs (Meusnier et al., 2001; Singh et al., 2011; Singh and Reddy, 2014; Alsufyani et al., 2020). Nevertheless, it has been observed that the use of chlorinated and unchlorinated seawater entails SAD formation (Bulboa et al., 2013; Arbaiza, 2016, 2023). Therefore, in order to favor SAD formation, it may be unnecessary to use filtrated or chlorinated water, since this process implies extended times for chlorination or dechlorination, leading to logistic delays and the elimination of bacteria that could favor growth or SAD formation.
Likewise, the substrate treatment (biologized and non-biologized) exhibited interaction with algae treatments, but it was not significant in the formation of SADs, even though previous studies state that washing and disinfecting the substrate with sodium hypochlorite allows eliminating attached microorganisms that can affect the process (Macchiavello et al., 2012; Zapata-Rojas et al., 2020). Nevertheless, some studies refute the above, implying that the presence of biofilms (bacteria and mucilage) may increase the surface energy of the substrate, thus favoring the settling of seaweeds (Lobban and Harrison, 1990; Hurd et al., 2014). This study, however, showed the formation of SADs with biologized and non-biologized substrates.
In this research, SAD formation was observed in almost all treatments with chlorinated and unchlorinated water, biologized and non-biologized substrates, and washed and unwashed algae. An increase in biomass was also observed in some treatments. However, despite the controlled conditions of the culture, the presence of epiphytes and diatoms could be observed, which may have altered the formation of SADs and caused the depigmentation or necrosis of the algae. These microorganisms have effects on the culture, notably through competition for light and nutrients in the medium and by increasing density and/or weight on the host algae, leading to detachment from the substrate (Fletcher, 1995; Bulboa, 2006). In this study, the presence of epiphytes persisted in the algae despite being washed, which is probably due to the fact that they developed in untreated seawater or that, during the experimentation phase, no GeO2 or penicillin was added. According to Arbaiza (2016), these additives help to control the presence of diatoms and cyanobacteria, which is why it is recommended that a rigorous cleaning is carried out and that the culture is provided with a continuous flow system. A continuous water flow system enables the capture of nutrients in the marine medium, as suggested by Balboa et al. (2013).
CONCLUSIONS
This study provides an idea of the initial processes required by C. chamissoi for cultivation by vegetative propagation. In this case, it is shown that the prior washing of seaweeds may favor a greater production of SADs in short periods of time. However, in the interaction of variables, it could be observed that using chlorinated and unchlorinated water and biologized and non-biologized substrate also allows for the formation of SADs. It is suggested that, in addition to having a controlled environment, additives could be used to prevent the growth of diatoms or other microorganisms.