INTRODUCTION
Mangroves are intertidal ecosystems distributed along the tropical and subtropical coasts of the world, providing a wide range of ecosystem services (Giri et al., 2011; Bunting et al., 2022). These ecosystems are not isolated; they are strongly linked to the social and cultural dynamics of local communities, and are therefore regarded as socio-ecological systems, or socioecosystems (Dahdouh-Guebas et al., 2021). In addition to being important settings with high levels of biodiversity, mangroves stabilize the coastline, reduce erosion, aid in maintaining biodiversity, capture carbon, and protect coastal communities, increasing their adaptive capacity and making them more resilient to the impacts of climate change (Spalding et al., 2014). Mangroves also help to maintain the food security of local communities, as they provide living and breeding sites for marine organisms such as fish, mollusks, crabs, and shrimp (Sandilyan and Kathiresan, 2012). The complex three-dimensional habitat created by mangroves supports the production of both artisanal and industrial fisheries, which are sources of protein and income for coastal communities (Unep-Nairobi Convention/Usaid/Wiomsa, 2020).
In addition to the anthropic threats faced by mangrove socioecosystems (which include forest overexploitation; land cover conversion for large-scale activities such as agriculture, forestry, human development, and infrastructure; water diversion for irrigation; the establishment of aquaculture ponds; and urban expansion), mangroves are also threatened by the impact of climate change (Ward et al., 2016; Worthington et al., 2020). Among the impacts of climate change that affect mangroves and exacerbate other direct impacts are changes in temperature and precipitation; increased storm frequency, sedimentation rates, and coastal erosion; water supply issues; increased pathogens; and rising sea levels (Alongi, 2015; Leal and Spalding, 2022).
Colombia’s mangrove forests contain significant carbon reserves (Leal and Spalding, 2022), with extensive coverages in the Sanquianga National Natural Park (80000 ha of mangrove), which is located in the Pacific region and features the largest mangrove area in the country and South America (Parques Nacionales Naturales, 2018). In addition to the extensive coverage and good conservation status of the mangroves of the Colombian Pacific (Spalding et al., 2007), which have been described as the most luxuriant and wet on the continent (West, 1956), the tallest mangroves in America (Castellanos et al., 2021) and the world (Simard et al., 2023) can be found in this region. In the Colombian Caribbean, the largest mangrove extension is associated with the Magdalena River delta system and the Ciénaga Grande de Santa Marta (39899 ha) (Rodríguez-Rodríguez et al., 2018). It is worth highlighting the marked contrast between Colombia’s Pacific and Caribbean biogeographical regions in terms of pressures and threats, mainly regarding those associated with changes in land use, resource extraction, coastal erosion, and urbanization (Rodríguez-Rodríguez et al., 2018; Blanco-Libreros and Ramírez-Ruíz, 2021), with the Caribbean region facing greater threats.
According to Murillo-Sandoval et al. (2022) , over the 1984 - 2000 period, mangrove cover in Colombia decreased by 14 % (48000 ha). In the Pacific, the decline started in 2004, while, in the Caribbean, mangrove cover decreased between 1984 and 1988 and after 2012. However, the only drivers of change identified in this work were associated with human activities such as agricultural expansion and road construction, leaving aside factors related to the impacts of climate change or climate variability, such as coastal erosion generated by rising sea levels.
In Colombia, mangroves are not isolated systems, as they are home to Agrocolombian and Amerindian, to fishermen without a specific ethnic association, and to a large number of residents associated with urban mangroves (Blanco-Libreros and Ramírez-Ruíz, 2021), whose food security largely depends on these systems, since a low income level and a limited capacity to acquire goods and services are among the common characteristics of these communities. These conditions can be exacerbated by the impacts associated with climate change. Although some studies have addressed the issue of mangrove socioecosystem degradation due to anthropogenic effects in Colombia (Botero and Salzwedel, 1999; Garcés-Ordoñez and Bayona, 2019; Garcés-Ordoñez et al., 2023), assuming that such pressures can exacerbate the adverse effects of climate change, they do not focus on the impacts of climate change on mangrove socioecosystems.
The character and severity of climate change impacts depend not only on the threats, which are expected to intensify in future scenarios, but also involve exposure variables and the system’s vulnerability to climate change (IPCC, 2022). Generally, exposure is a determining factor of risk, specific to the threat of climate change, whereas vulnerability results from a broad range of factors, such as socioeconomic conditions in the case of human systems, where the variables of sensitivity and adaptive capacity are related. Since the fifth assessment report of the Intergovernmental Panel on Climate Change (IPCC, 2014), climate risk management has been emphasized in order to facilitate the identification of necessary management strategies within a context of uncertainty intensified by climate change. This approach has the virtue of facilitating the understanding of the public and decision-makers about the measures to be adopted regarding climate change (Ideam et al., 2017).
It is necessary for the country’s mangrove socioecosystems to remain in a healthy state that allows contributing to global and national climate strategies and the sustainable development goals, so that they play a fundamental role in mitigating climate change through carbon storage while safeguarding biodiversity, reducing the risk of disasters, and maintaining optimal resilience levels to face rapid global warming. The country’s commitments in terms of climate (mitigation and adaptation) do not exclude mangroves, as the goals of the Nationally Determined Contribution (NDC) include nature-based solutions for the protection of these ecosystems and their restoration, allowing the country to advance in climate change management.
Given that the impacts of climate change and climate variability can endanger mangrove socioecosystems and the ecosystem services and benefits they provide to communities, which is exacerbated by socioeconomic pressures, this work seeks to conduct a vulnerability and climate risk analysis for the mangroves of Colombia. The objective is to identify climate threats and vulnerability conditions to define the risk level of the mangrove socioecosystem under changing climate conditions in the Colombian Pacific and Caribbean, in order to identify adequate adaptation and management strategies. These results constitute a baseline for the country’s public policy instruments and, among others, for the territorial climate change adaptation plans of departments and municipalities.
STUDY AREA
Colombia is located in the north of South America and has coasts along the Pacific Ocean and the Caribbean Sea. In the country, there are approximately 280 000 ha of mangroves, out of which 71 % are in the Pacific and 29 % are along the Caribbean coast, including those in the oceanic insular region of San Andrés and Providencia (https://geovisorsigma.invemar.org.co/) (Figure 1). The Colombian Pacific includes the moist lowland forests of the Chocó-Darién Ecoregional Complex (CECH) (Olson and Dinerstein, 2002), one of the regions with the highest precipitation in the world (Poveda and Mesa, 2000). Due to the double passage of the Inter Tropical Convergence Zone (ITCZ), a bimodal annual precipitation cycle predominates in the central and northern areas (Poveda et al., 2007). Meanwhile, towards the latitudinal extremes (Ecuador and Panama), this cycle is unimodal. In the bimodal cycle, maximum precipitation occurs between April and May and between October and November, and the minimum values are observed between December and February and between June and August (Poveda et al., 2006). The interannual variability associated with the El Niño Southern Oscillation (ENSO) is another significant cycle in the region, exhibiting an amplitude similar to that of the annual cycle (Fiedler and Talley, 2006). In the Colombian Caribbean, the climate regime is distinctly bimodal, with rainy (August-November) and dry periods (December-February) interrupted by a transition period (March-July). Unlike the Pacific, where the tidal range can reach up to four meters, in the Caribbean, there are microtides with an amplitude of less than 0.5 m. Moreover, there are marked differences in mangrove structure between the Pacific and the Caribbean, as the latter are less developed than those on the Pacific coast (Invemar, 2013).
MATERIALS AND METHODS
The analysis followed the Intergovernmental Panel on Climate Change’s definition of vulnerability and risk (IPCC, 2022), where the former is a function of sensitivity and adaptive capacity, and the latter is a function of threat and vulnerability. Given that mangrove socioecosystems in Colombia are not isolated but strongly connected with the social and cultural dynamics of local communities, they were considered as a socioecosystem with two components, for which different indicators were identified. These components are the mangrove ecosystem and its biodiversity, and the human communities associated with mangroves and their livelihoods.
The vulnerability and risk analysis was conducted for 46 coastal municipalities with mangrove cover in the two littorals of Colombia, according to the Information System for the Management of Mangroves in Colombia (SigmaV8) (https://geovisorsigma.invemar.org.co/). In total, 30 municipalities in the Caribbean and 16 in the Pacific were included in the analysis. Although there are seven municipalities in the Colombian Caribbean with mangrove coverage but no coastal zone, these were not included in the analysis because they did not report the indicators associated with the coastal and oceanic zone of all analysis units (e.g., changes in the coastline and changes in sea surface temperature, among others). The mangroves in San Andrés and Providencia were also excluded, because these municipalities did not have many of the socioeconomic indicators of the other units.
After an interdisciplinary analysis (involving governance, biodiversity, and climate change teams at WWF Colombia), different indicators regarding threats, sensitivity, and adaptive capacity were prioritized. In all cases, official information sources and/or data from state entities were used. 22 threat indicators (Table 1), eight sensitivity indicators (Table 2), and 18 adaptive capacity indicators (Table 3) were employed. The indicators in each dimension (threat, sensitivity and adaptive capacity) were assigned according to the Third National Communication on Climate Change (TCNCC) (Ideam et al., 2017).
Table 1 Threat indicators used in the vulnerability and climate risk analysis of the mangrove socioecosystem
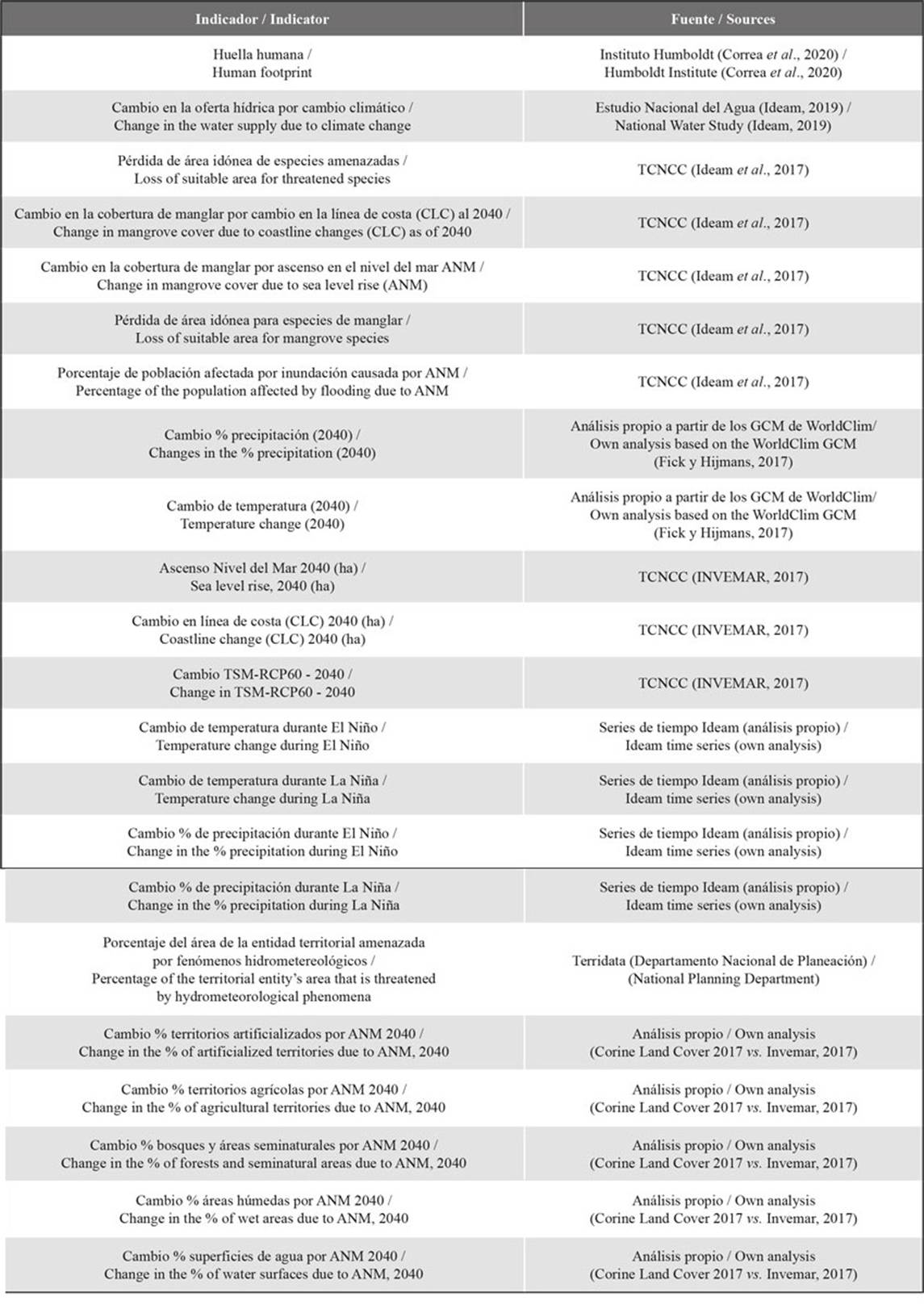
Table 2 Sensitivity indicators used in the vulnerability and climate risk analysis of the mangrove socioecosystem
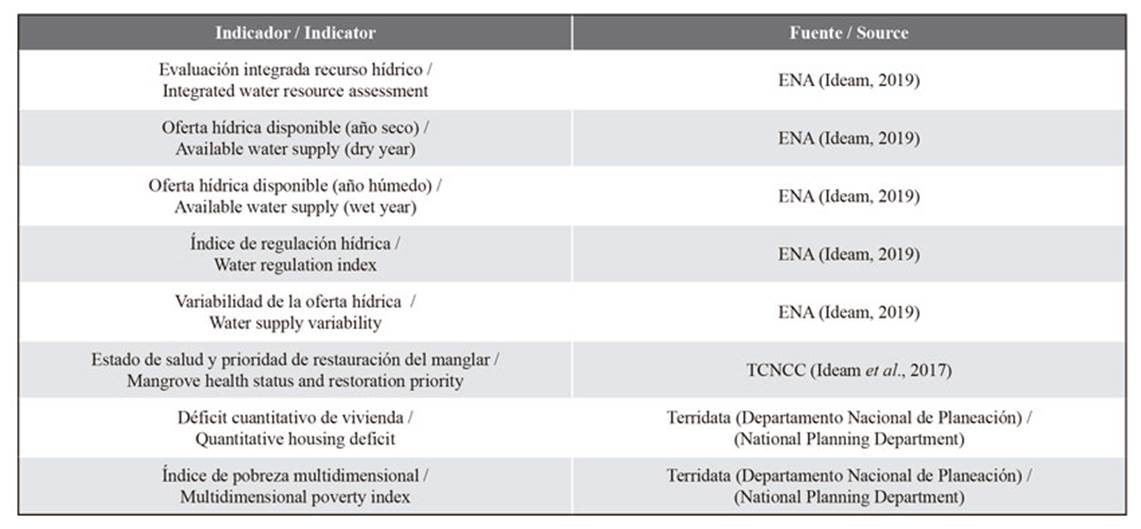
Table 3 Adaptive capacity indicators used in the vulnerability and climate risk analysis of the mangrove socioecosystem
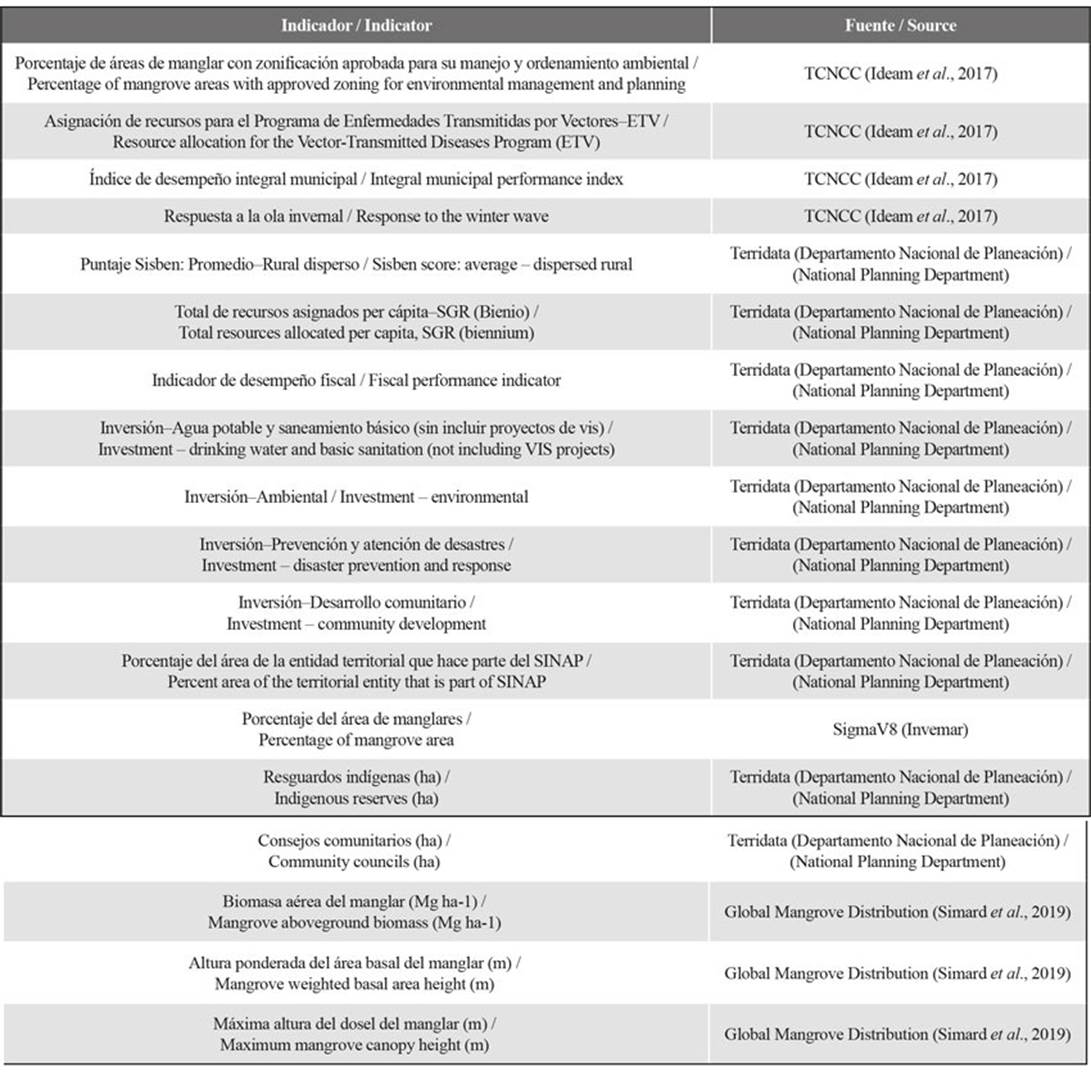
Given that the scenarios of the Third National Communication on Climate Change (TCNCC) (Ideam et al., 2017) are available in vector format and are categorized by ranges, they do not adequately capture the variability of temperature and precipitation changes in future scenarios. Additionally, the TCNCC generated scenarios using multi-model ensembles of RCP (representative concentration pathways) and GCM (global circulation models), which is not suitable since, in addition to mixing the uncertainty associated with the GCM, it does not allow differentiating between the various greenhouse gas concentration trajectories. Therefore, it was necessary to model future scenarios regarding precipitation and temperature in Colombia by using the GCM of the CMIP5 project, which are available on the WorldClim platform (https://www.worldclim.org/) (Fick and Hijmans, 2017). The CMIP5 project GCM used were those best fitted for Colombia according to Arias et al. (2021). The scenario employed was RCP 6.0 for the 2040 period. The percent changes in precipitation and temperature delta were calculated relative to the 1970 - 2000 reference period (Fick and Hijmans, 2017) (Table 1).
The percent average precipitation and temperature changes during El Niño and La Niña events (Table 1) were calculated using the climatological normal time series from Ideam 1980 - 2010 for 307 stations across the national territory. The time series were obtained from the Ideam website (http://dhime.Ideam.gov.co). The definition of an ENSO (El Niño Southern Oscillation) period was adjusted to the operational designation of El Niño/La Niña under the ONI index by the National Oceanic and Atmospheric Administration (NOAA), which defined these events as “a phenomenon in the equatorial Pacific characterized by an increase/decrease in Sea Surface Temperature (SST) with respect to the base period, in the Niño 3.4 region, and equal or greater in magnitude to (+/-) 0.5 °C, averaged over a period of three consecutive months, and sustained for five periods” (Larkin and Harrison, 2005). When the ONI index was in its warm phase, an El Niño (EN) period was identified, while, when the ONI index was in its cold phase, a La Niña (LN) period was identified. The remaining periods were considered neutral and employed as the baseline reference to calculate the percent changes in precipitation and temperature during each event (EN/LN).
The percent change in land cover (artificialized and agricultural territories, forests and seminatural areas, wet areas, and water surfaces) (Table 1) was estimated by crossing layers of the 2017 land cover of Colombia at a 1:25.000 scale (https://siac-datosabiertos-mads.hub.arcgis.com), with the projected increase in sea level rise by 2040 (Invemar, 2017). The human footprint index (Table 1) was used as an indicator of anthropogenic impact. This index was calculated by Correa et al. (2020) while considering land use, rural population density, distance to roads, distance to urban settlements, the fragmentation index, the biomass index relative to the original biomass, and the time of intervention. The remaining threat indicators (Table 1) were obtained from the TCNCC (Ideam et al., 2017), from the National Water Study (ENA) (Ideam, 2019), from the TCNCC’s indicators of the marine-coastal and insular vulnerability analysis regarding climate change (Invemar, 2017), and from the Terridata database of the National Planning Department (https://terridata.dnp.gov.co/). The sensitivity indicators (Table 2) were obtained from the National Water Study (ENA) (Ideam, 2019), from the TCNCC (Ideam et al., 2017), and from the Terridata database. Finally, the adaptive capacity indicators were obtained from the TCNCC (Ideam et al., 2017), from the Terridata database, from the information system for the management of mangroves (Sigma, http://sigma.invemar.org.co/), and from the layer Global Mangrove Distribution, Aboveground Biomass, and Canopy Height layer of the ORNL DAAC (Simard et al., 2019).
Regarding each dimension (threats, sensitivity, and adaptive capacity), a principal components analysis (PCA) was conducted for each coast (Pacific and Caribbean). Initially, a PCA that included both coasts was conducted, but, due to the biogeographical and socioeconomic differences between these two regions of Colombia, we evidenced the need for a separate analysis of each coast, allowing to corroborate these significant differences. In a PCA, the components or linear combinations of the original variables are obtained, enabling the simplification of the study universe, focusing on the components that synthesize the maximum residual variance. The objectives of a PCA can be summarized in three points: 1) generating new variables that can express the information contained in the original dataset, 2) reducing the dimensionality of the handled data to perform future analyses, and 3) eliminating some variables that contribute little information to the analysis (Legendre and Legendre, 1998; Emery and Thompson, 2014). This last point was one of the criteria used to prioritize the indicators of each dimension (22 for threats, 8 for sensitivity, and 18 for adaptive capacity). Based on the proportion of variance explained by each principal component (PC), the components that cumulatively explained at least 60 % of the total variance of the data were selected. In all cases (threat, sensitivity, and adaptive capacity), this occurred with only two PC. The threat, sensitivity, and adaptive capacity indices were obtained by weighting the subindices of the sampling units (‘scores’ of the PCA) by the variability contribution of each PC. Vulnerability was calculated as sensitivity over adaptive capacity (V=S/CA), and risk as threat by vulnerability (R=A*V) (Ideam et al., 2017; IPCC, 2022). Finally, to facilitate the interpretation of vulnerability and risk, the obtained indices were standardized to values between 0 and 1 for each coast and placed in four categories using the low, medium, high, and extreme quartiles.
RESULTS
The PCA, which included both coasts, allowed identifying that the average vulnerability and risk index was higher for mangroves located in the municipalities of the Caribbean when compared to those in the Pacific. On average, in the Caribbean mangroves, a higher sensitivity, a lower adaptive capacity, and a higher threat index were found (Table 4).
Table 4 Average sensitivity, adaptive capacity, vulnerability, threat, and risk indices for the Pacific and Caribbean mangroves of Colombia

In the PCA for the Colombian Caribbean, the first two PCs extracted to calculate the threat, sensitivity, and adaptive capacity indices, from which the vulnerability and risk indices were derived, captured 72.10 %, 85.19 %, and 76.57 % of the variability, respectively. The threat indicators that showed the highest correlation (> 0.60) with the PCs were the changes in water supply due to climate change, the changes in sea surface temperature (SST) in the future scenario (2040), the sea level rise in the future scenario (2040), the changes in temperature and precipitation in the future scenario (2040), the changes in temperature during El Niño events, the loss of suitable area for threatened species, the percentage of the territorial entity’s area that is threatened by hydrometeorological phenomena, and the human footprint. The sensitivity indicators that had the highest correlation (> 0.60) with the PCs were the water supply available in a dry year, the water regulation index, and the health status and restoration priority of the mangrove. The adaptive capacity indicators that showed the highest correlation (> 0.60) with the PCs were the percentage of mangrove areas with approved zoning for environmental management and planning, the total resources per capita allocated by the General System of Royalties (SGR), the percentage of mangrove area, the mangrove aboveground biomass, and the mangrove weighted basal area. The highest threat indices for the Caribbean mangroves (extreme category) were found between the center and south of the littoral, in the municipalities of Puerto Colombia, Juan de Acosta, and Piojó (Atlántico); in Tolú and Coveñas (Sucre); and in Puerto Escondido and Los Córdobas (Córdoba) (Figure 2, Table 4).
The mangroves exhibiting the highest vulnerability (extreme category) were associated with the municipalities of Manaure, Uribia, and Riohacha (La Guajira); Tubará, Juan de Acosta, Barranquilla, and Puerto Colombia (Atlántico); and Puerto Escondido and Los Córdobas (Córdoba). In addition, the mangroves in Dibulla, Ciénaga, Piojó, Santa Catalina, Cartagena de Indias, Moñitos, Arboletes, San Juan de Urabá, and Necoclí reported a vulnerability index in the high category (Figure 3, Table 4). In total, 60 % of the municipalities exhibited mangroves with extreme and high vulnerability. On the other hand, mangroves with the highest risk index were found in the municipalities of Manaure (La Guajira); Barranquilla, Puerto Colombia, Tubará, and Juan de Acosta (Atlántico); and Puerto Escondido and Los Córdobas (Córdoba) (Figure 4, Table 4). Seven additional municipalities exhibited a high risk index, i.e., Uribia, Riohacha, Piojó, Santa Catalina, Moñitos, Arboletes, and San Juan de Urabá (Figure 4, Table 4). In total, 47 % of the municipalities showed mangroves with extreme and high risk.
Table 5 Threat, vulnerability, and risk indices for the mangrove socioecosystem in the Colombian Caribbean.
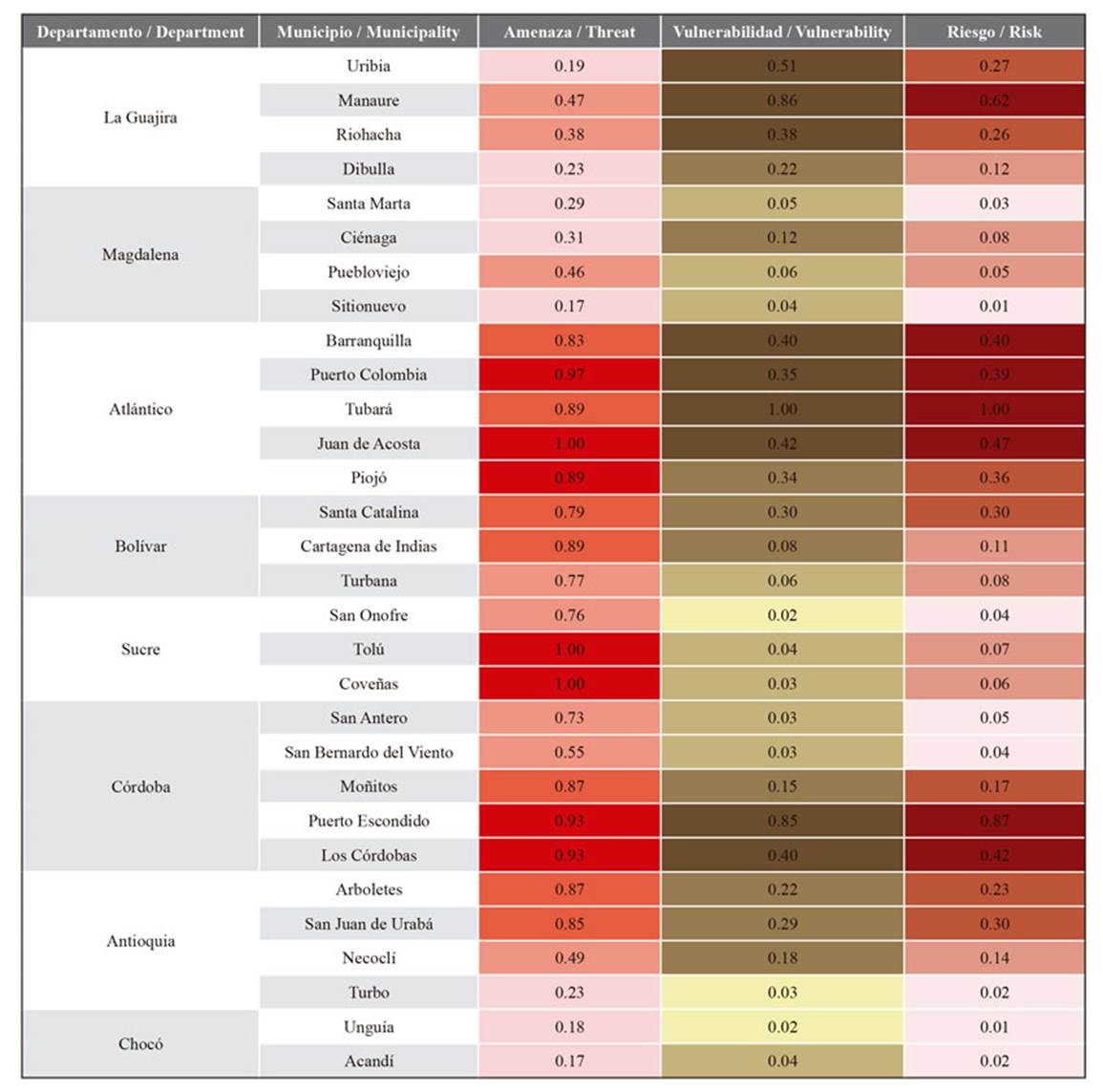
In the PCA for the Pacific region of Colombia, the first two PCs extracted to calculate the threat, sensitivity, and adaptive capacity indices captured 77.55 %, 80.05 %, and 71.17 % of the variability, respectively. The threat indicators that showed the highest correlation (> 0.60) with the PCs were the changes in water supply due to climate change, the changes in precipitation in the future scenario (2050), the changes in temperature during El Niño events, the changes in precipitation during La Niña events, and the changes in mangrove cover due to coastline changes by 2040. The sensitivity indicators that had the highest correlation (> 0.60) with the PCs were the integrated water resource assessment, the water supply available in a dry year, the water regulation index, and the mangrove health status and restoration priority. The adaptive capacity indicators that exhibited the highest correlation (> 0.60) with the PCs were the integral municipal performance index, the fiscal performance indicator, the percentage of mangrove area, and the mangrove aboveground biomass. The highest threat indices for the Pacific mangroves (extreme category) were mainly found in the southern part of the littoral, in the municipalities of Santa Bárbara, Olaya Herrera, and Francisco Pizarro (Nariño); and also in the north, in the municipality of Bahía Solano (Chocó) (Figure 5, Table 6).
The mangroves that exhibited the highest vulnerability (extreme category) were associated with the municipalities of Bahía Solano (Chocó); Buenaventura (Valle del Cauca); and La Tola and Olaya Herrera (Nariño). In addition, the mangroves of López, El Charco, Francisco Pizarro, and Tumaco had a vulnerability index in the high category (Figure 6, Table 5). In total, 50 % of the municipalities exhibited mangroves with vulnerability in the extreme and high categories. The mangroves with the highest risk were found in the municipalities of Bahía Solano (Chocó) and La Tola, Olaya Herrera, and Francisco Pizarro (Nariño). Five additional municipalities exhibited a high risk index, i.e., Juradó, López, Santa Bárbara, El Charco, and Tumaco (Figure 6, Table 5). In total, 56 % of the municipalities reported mangroves with risk indices in the extreme and high categories.
DISCUSSION
The mangroves in the Colombian Caribbean exhibited a higher risk index than those in the Pacific and a greater number of climatic threats that explained the increased risk, in addition to the human footprint index, which quantifies anthropogenic effects. Among the climatic threats, some were found on a seasonal scale (e.g., the percentage of the territorial entity’s area that is threatened by hydrometeorological phenomena), others on an interannual scale (e.g., the change in temperature during El Niño events), and others associated with climate change (e.g., sea level rise and changes in water supply due to climate change), as well as anthropogenic threats that can exacerbate climatic ones and increase the socioecosystem’s vulnerability levels.
Over the last two decades, the coastal wetlands of the Colombian Caribbean have experienced a reduction in runoff, and, consequently, they have exhibited a decrease in the water supply to ecosystems such as mangroves (e.g., Ciénaga Grande de Santa Marta, or CGSM; León-Atrato) (Ahlén et al., 2021). According to the change in water supply due to climate change, this trend will mainly intensify in the municipalities of Puerto Colombia, Tubará, Juan de Acosta, and Piojó, where the reduction will be between 38 and 45 %. These municipalities of the department of Atlántico, along with Barranquilla, reported the mangroves with the highest risk index. Although the analysis unit was municipal, the effects on the basin scale must also be considered. For example, the Magdalena River, the main contributor of freshwater to the CGSM, exhibits significant flow variability during ENSO events, which in turn affects the salinity of the system. The two phases (the warm El Niño and the cold La Niña) can create extreme conditions that deteriorate the mangrove. During El Niño, there is an increase in salinity due to the reduction of freshwater flows entering the lagoon system, which decreases the density of seedlings and propagules (Invemar, 2018). On the other hand, during periods of lower salinity, associated, for example, with La Niña, the dispersal of freshwater species and the deterioration of mangrove forests are favored (Invemar, 2000). Although these changes may be induced by a changing climate, associated with an increase in temperature and evapotranspiration and with a decrease in humidity, there is a component of variability that is explained by changes in land use and other anthropogenic pressures, which is very evident in the Colombian Caribbean. For example, in the case of the CGSM, the damming and irrigation of water for crops are important factors that explain the increase in salinity rates and the massive degradation of mangroves, which has also been evidenced in other areas of the Caribbean (Jaramillo et al., 2018).
Climate change is expected to reduce annual precipitation in the central and northern regions of the Colombian Caribbean (Ideam et al., 2017), which will drastically increase water stress in coastal wetlands. In a future scenario (2050), the greatest reductions in precipitation in the Colombian Caribbean will be found in Puerto Colombia and Barranquilla (Atlántico), Manaure (La Guajira), and Sitionuevo (Magdalena). The mangroves associated with these municipalities, except for Sitionuevo, exhibited the highest risk indices in the Caribbean. A decrease in precipitation can lead to negative changes in freshwater flows and therefore reduce mangrove productivity, growth, seed survival, and changes in the associated species composition, favoring those more tolerant to salinity (Ellison, 2010). As precipitation decreases, mangrove coverage and diversity are likely to decrease, generating hypersaline, unvegetated flats (Snedaker, 1995; Lovelock et al., 2016). A recent and extensive loss of mangroves in northern Australia, for example, has been linked to drought conditions caused by climate change (Duke et al., 2017). Conversely, in the areas surrounding the Sierra Nevada de Santa Marta in the Colombian Caribbean, an increase in precipitation is expected by 2040, with values oscillating between 70 % and 80 % (as per the results from the analysis based on the GCM of WorldClim; Fick and Hijmans, 2017). This includes the mangroves in the municipalities of Santa Marta and Ciénaga (Magdalena) and Dibulla (La Guajira). An increase in precipitation may favor the expansion of the mangrove cover area, the diversity of mangrove zones, and the growth rates of some mangrove species (Field, 1995).
For the Colombian Caribbean, in a future scenario, the coastal waters of the municipalities of Juan de Acosta, Tubará, and Piojó (Atlántico) and Santa Catalina (Bolívar) will experience a 0.6 - 0.8 °C increase in SST. Since coastal and shallow areas are affected by increases in SST, mangroves are expected to be impacted by these changes, as they can cause thermal stress on the structure of mangrove roots and on seed establishment (Unesco, 1992), in addition to affecting photosynthesis (Andrews et al., 1984).
Though not an evaluated indicator, the intensification of storms in terms of frequency and intensity is strongly associated with the increase in SST due to climate change (Trenberth, 2005). This intensification represents an additional threat to mangroves. For example, significant storm impacts have resulted in massive mangrove mortality in the Caribbean over the last 50 years (Armentano et al., 1995). In the latest assessment carried out by Global Mangrove Watch, it was identified that major storms or hurricanes (e.g., Ida, Sandy) caused significant losses in mangrove cover. Although this is not a problem for the entire continental Caribbean, it is indeed for the insular Caribbean (Archipelago of San Andrés, Providencia, and Santa Catalina) and for the upper Guajira, where mangrove cover are reduced. These areas exhibit a similar risk level, with 5 - 19 hurricanes for every 100 years, as well as 10 - 29 tropical storms (https://www.nhc.noaa.gov/). For instance, on the island of Providencia (insular Colombian Caribbean), after the passage of hurricanes Eta and Iota between October and November 2020, a loss of foliage in mangrove forests was observed, with percentages of dead individuals reaching 46.4 % (Garcés-Ordoñez et al., 2021; Invemar, 2021).
Some models have suggested that an increase in the intensity of storms and hurricanes will cause a reduction in the average height of mangroves (Ning et al., 2003). Furthermore, the projected increases in the frequency of flooding events (Church et al., 2004), be it due to storms or changes in the mean sea level, could affect the health and composition of mangroves due to salinity, recruitment, and sediment balance changes (Gilman et al., 2006). Storm surges can also flood mangroves and, when combined with a sea level rise, can lead to their destruction. The flooding caused by increases in precipitation, storms, or relative sea level rise can result in decreased productivity, photosynthesis, and survival. For instance, the flooding of lenticels in aerial roots can cause a decrease in oxygen concentrations within the mangrove ecosystem, resulting in death (Ellison, 2010).
The sea level rise is the most significant climatic challenge faced by mangrove ecosystems (Field, 1995). Geological records indicate that past sea level fluctuations have created crises and opportunities for mangrove communities, which have either survived or expanded into various refuges (Field, 1995). Mangroves can adapt to sea level increases if it occurs slowly enough (Ellison and Stoddart, 1991), if there is an adequate space for expansion, and if other environmental conditions are met. Their success largely depends on the sediment production and accumulation. If their sedimentation rate exceeds that of the sea level rise, some mangroves will have an adaptive advantage over other communities that lack this characteristic. According to McKee and Vervaeke (2018) , to avoid submersion during sea level rise, mangroves can build vertical soil surfaces via the accumulation of inorganic sediments and organic matter, thus not being altered by the rise. However, McKee et al. (2021) found that some mangroves will not be able to adaptively respond to sea level rise rates and highlight that, in order to understand these dynamics, conclusions should not be based solely on surface accretion data, as this can lead to an inaccurate assessment of coastal vulnerability. Therefore, all movements of the land surface and subsoil must be considered to understand local sea level trends and to assess the risk of mangrove submersion.
Another adaptive advantage of mangroves will be their ability to migrate inland, where topography and the infrastructure associated with roads, dykes, crops, and navigation channels will play a crucial role, since they can hinder migration onto land. To understand the impact of rising sea levels on mangrove socioecosystems, the factors affecting the ecosystem balance must also be considered, such as the type of substrate, coastal erosion, tidal range, local tectonics, freshwater availability, sediments, and the salinity of both the water column and the soil.
As for sea level rise, climatic variability at the seasonal and interannual scales (e.g., changes in precipitation and in the frequency and intensity of storms) must also be considered, as it can exacerbate the factors that affect the mangrove’s response to rising sea levels. Although not all regions of Colombia will experience a similar sea level rise rate, the mangroves in low-relief areas with little sediment input will be the most vulnerable. In contrast, mangrove ecosystems with a broad supply of sediments and/or sufficient space to move inland will likely survive the projected sea level rise rates (McLeod and Salm, 2006). Under this scenario, it is more likely to find a better response in Pacific mangroves than in those of the Caribbean.
Mangrove species have demonstrated varying tolerances to changes in sea level, salinity, and storms, which is why they are collectively regarded as a resilient ecosystem. Resilience should be understood as a system’s ability to undergo, absorb, and respond to change and disturbance while maintaining its functions (Carpenter et al., 2001). Therefore, maintaining or increasing resilience in mangrove conservation plans requires an understanding of how mangroves respond to climate changes, of the factors that help them to survive these changes, and, consequently, of which mangroves have a greater adaptive capacity and thus better chances to survive these changes.
Among the Pacific mangroves, those located in the municipalities of Olaya Herrera and La Tola exhibited vulnerability and risk levels in the extreme category. These mangroves are of great importance because many of them are located in the Sanquianga National Natural Park (NNP), which houses the largest mangrove area in the Colombian Pacific and South America (Parques Nacionales Naturales, 2018). This area has undergone significant changes in recent decades due to the diversion of the Patía River towards the Sanquianga River, which started in 1972 and increased with the earthquake of 1979, causing the estuary to become a coastal deltaic system (Restrepo and Kettner, 2012). Despite the severe changes undergone by the system, Perea-Ardila and Murillo-Sandoval (2022) found that, over a 21-year period (1996 - 2017), the mangroves of the Sanquianga NNP experienced a net gain of 243.97 ha, although, from 2007 to 2017, this value was only 118.71 ha. These gains may be associated with the colonization of new lands and the migration of sandbanks, favoring the colonization of new mangrove areas. On the other hand, during the same period (1996 - 2017), the mangrove cover of the Sanquianga NNP experienced losses of 122.30 ha, mainly at the mouth of Guascama, south of the protected area. According to Murillo-Sandoval et al. (2022), for the 1984 - 2020 period, losses in the protected area have amounted to 500 ha, mainly recorded along the coastline. The strong discharge of the Patía River through the Patía-Sanquianga delta may be eroding the coastal and river edges, generating strong sedimentation processes that are causing a reduction in mangrove cover.
Given that one of the indicators that captured the most variability in the vulnerability and risk analyses for the Colombian Pacific was the change in mangrove cover due to coastline changes by 2040, the coastal edge erosion trend in the localities where the mangroves with the highest risk are located would be expected to continue or increase in the future. According to Bunting et al. (2022) , among the natural causes of mangrove loss, coastal erosion is the most common worldwide. However, some human factors can exacerbate erosion processes in many areas, e.g., the reduction or interruption of sediment supply from rivers or coastal areas. Additionally, the sea level rise caused by climate change can exacerbate coastal erosion in the littoral and lead to a retreat of the coastal edge (Giri and Long, 2016).
Interannual climatic variability, mainly associated with the El Niño Southern Oscillation (ENSO) cycle, in both its warm (El Niño) and cold (La Niña) phases, poses risks of unfavorable impacts for human and natural systems (Enfield, 2001). One example is the estuarine system, associated with large mangrove belts in the Colombian Pacific and Caribbean. According to González and Correa (2001) regarding a study in the San Juan River delta, precipitation, river discharge, and sea level rise on an interannual scale are associated with the ENSO cycle. The direct impact of these events produces significant differences between the minimum and maximum river discharges, and it causes regional sea level variations, with marked effects on the coastline of the delta (Restrepo and Kjerfve, 2000; Restrepo et al., 2002). Sea level increases, according to Morton et al. (2000) , could have been the cause for the flooding of El Choncho barrier island in 1998 and for the strong coastal erosion process that led to the retreat of the coastline, destroying natural coastal protection structures such as mangroves. However, it should be considered that the two ENSO phases (El Niño/La Niña) can affect mangroves differentially. During an El Niño event with scarce rainfall, high salinity and low freshwater inputs from rivers and rain can cause the loss of significant mangrove areas, particularly with areas of restricted tides and/or in dry regions (Hickey et al., 2021), such as the Colombian Caribbean. On the other hand, during a La Niña event with a significant increase in rainfall, a greater contribution of freshwater and sediments can favor the increase in cover. Nevertheless, a greater amount of water can cause the excessive flooding of mangroves, which could have effects similar to those of immersion under conditions of long-term sea level rise and lead to the loss of mangrove cover (Pelckmans et al., 2024).
Mangrove forests are threatened not only by climatic factors, but also by anthropogenic ones, which was evident in their significant contribution to the variability of the dataset of the human footprint index for the Colombian Caribbean. In the metric of this indicator’s calculation, the following variables are considered: land use, rural population density, distance to roads, distance to urban settlements, the fragmentation index, the biomass index relative to the original biomass, and time of intervention (Correa et al., 2020). Although mangroves are dynamic systems, which is reflected in their loss and gain processes, in recent decades, the losses caused by anthropogenic factors have significantly increased, and the Colombian Caribbean and Pacific are no exception. To this strong anthropogenic pressure, the pressures associated with climate change must be added, as they will exacerbate current pressures in the near future, constituting a significant threat to mangrove ecosystems (Giri et al., 2011).
Garcés-Ordoñez et al. (2023) found that, in the Mallorquín ciénaga in the Colombian Caribbean, the main mangrove degradation factors are the discharge of wastewater, unregulated garbage dumps, mangrove cutting, and urban expansion. Said discharges are impacting water and soil quality, while cutting and urban expansion have caused significant changes in the ecosystem, critically impacting the integrity of water, soil, and forests. Urban expansion is a threat that is particularly observed in the Caribbean, as it is the more developed of the two coasts of Colombia and boasts a greater number of population centers and inhabitants. For example, the most populous city in the Colombian Caribbean is Barranquilla, with an urban area of 154 km² and a population of 1.3 million, whereas Buenaventura, the main city on the Pacific coast, has an urban area of only 21.6 km² and a population of 323 000 (https://www.dane.gov.co). Urban expansion, along with the expansion of agricultural, livestock, and industrial frontiers in coastal areas, constitutes a serious threat to sensitive ecosystems like mangroves, as it not only implies a change in land use with consequent biodiversity degradation, but also occupies the areas that mangroves would require to expand in response to coastline changes and sea-level rises. According to Murillo-Sandoval et al. (2022) , the transition from dense mangroves to other types of vegetation is the most significant conversion that has affected mangrove cover in Colombia.
Although, in the Colombian Pacific, the human footprint index was not one of the main indicators that explained the risk index, human activities such as gold mining, logging, and the expansion of coca cultivation can severely affect mangrove cover. According to Kjerfve et al. (2001) , it is clear that anthropogenic impacts associated with coastal development have a significant synergistic effect on amplifying the impacts of climate change, mainly those related to coastal erosion. As a result of these disturbances, estuarine zones, which, being an ecotone, host a great diversity, can experience notable ecological changes.
A critical transversal aspect regarding the climate risk in Colombia’s mangroves has to do with mitigation. Like all plant systems on Earth, mangroves capture carbon from the atmosphere and store it in aerial biomass (leaves, trunks, and roots) and in the soil (blue carbon). Additionally, soil roots can trap organic matter suspended in the water during tidal flows, which is why the amount of carbon stored in mangrove ecosystems can be up to four times greater than that stored by other forests, making them indispensable allies in the goal of achieving a carbon-neutral world (Lovelock and Reef, 2020). Globally, mangroves store approximately 6.23 (±2.3) gigatons of carbon, equivalent to 22.86 gigatons of CO2, and 87 % of this amount is found in the soil. At a global level, five countries hold 50 % of the total carbon stored in mangroves: Indonesia, Brazil, Nigeria, Australia, and Mexico. Colombia is in a second group with the Philippines and Madagascar, which, despite not having the largest mangrove extents, do have significant carbon concentrations (Leal and Spalding, 2022), in addition to the tallest mangroves in the world (Simard et al., 2023). As for mitigation, the climate implications of mangrove loss, primarily in the long term, include a reduction in carbon storage in vegetation and soils (Macreadie et al., 2021), as well as a reduction in the capacity to sequester carbon through photosynthesis and in the capture of material transported by rivers and coastal currents (Li et al., 2018). An increase in CO2 levels can generate greater photosynthetic activity and, therefore, an increase in mangrove growth rates (Lovelock, et al., 2016). However, it has been found that this relationship occurs only at low salinities (Ball et al., 1997), and thus a world enriched with CO2 will not necessarily lead to mangrove cover growth. Therefore, it is necessary for these large amounts of carbon stored in mangroves to be conserved, in order to prevent potential future CO2 emissions due to ecosystem degradation or disappearance. To ensure the sustainability of mangroves, significant efforts will be necessary to stop cover losses, restore those with some degree of deterioration, and increase their protection. Recovering restorable areas can support carbon sequestration and ensure the maintenance of significant amounts of carbon stored in aerial biomass and in the soil.
CONCLUSIONS
The assessment of climate risk for mangrove socioecosystems in Colombia, one of the focus points of this work, facilitates the identification of adaptation strategies and of the places where it is critical to implement them, in order to be able to reduce the risks associated with climate change (IPCC, 2022). In the long term, decreasing emissions can contribute, in some cases, to reducing extreme climatic events. However, given that climate change is a global phenomenon, this reduction, on the local scale, would make a contribution of little significance to reducing the risk due to climate change in the territory. Therefore, adaptation alternatives leading to risk reduction must be based on diminishing mangrove vulnerability and risk. They should place a special focus on Caribbean coastal mangroves, mainly those in the central area of the littoral in the department of Atlántico, as they reported the highest vulnerability and risk indices.
The reduction of risks and vulnerability may encompass a broad spectrum of actions that vary as needed, but it should generally be in line with the socioeconomic and sectorial development agenda of the country, some of these measures may be specific, e.g., education regarding the risk due to climate change and its relationship with the risk of local climatic disasters or the improvement of early warning and response systems in the face of extreme climate events, or they could be as general as the implementation of restoration programs. The important thing is that the management of the risk due to climate change should be focused on reducing risk and vulnerability, as well as on increasing mangrove resilience against potential adverse impacts. Colombia harbors the greatest relatively intact mangrove extensions in the western hemisphere, and, for their conservation, constant monitoring is required in order to support management strategies considering hydrological, climatic, and socioeconomic factors. In this sense, these results constitute an input for climate management in the mangroves of Colombia, and they shall contribute to the National Mangrove Program. Likewise, they will be able to contribute to the National Mangrove Restoration Program, to the Nationally Determined Contribution (NDC) of Colombia, to the E2050 Long-Term Climate Strategy of Colombia, and to the departments and municipalities’ Territorial Plans of Adaptation to Climate Change.