Services on Demand
Journal
Article
Indicators
-
Cited by SciELO
-
Access statistics
Related links
-
Cited by Google
-
Similars in SciELO
-
Similars in Google
Share
Ingeniería y Universidad
Print version ISSN 0123-2126
Ing. Univ. vol.19 no.2 Bogotá July/Dec. 2015
https://doi.org/10.1114/javeriana.iyu19-2.pncs
Performance of Novel Contact Stabilization Activated Sludge System on Domestic Wastewater Treatment1
Desempeño de una nueva configuración del sistema de estabilización por contacto en el tratamiento de agua residual doméstica2
Andrés Felipe Torres Franco3
Nancy Vásquez Sarria4
Jenny Alexandra Rodríguez Victoria5
1Submitted on: December 11th, 2013. Acceptance on: January 29th, 2015. This article is derived from the research project Carbon and nitrogen removal in a contact stabilization activated sludge system supported by Universidad del Valle (Colombia) and The International Foundation for science IFS (Sweden), developed by the research group Estudio y Control de la Contaminación Ambiental from Universidad del Valle, Cali, Colombia.
2Fecha de recepción: 11 de diciembre de 2013. Fecha de aceptación: 29 de enero de 2015. Este artículo se deriva del proyecto de investigación denominado Reducción de materia orgánica carbonácea y nitrogenada en un sistema de lodos activados en la modalidad de estabilización por contacto financiado por la Universidad del Valle (Colombia) y la Fundación Internacional para la Ciencia IFS (Suecia), desarrollado por el grupo de investigación Estudio y Control de la Contaminación Ambiental de la Universidad del Valle, Cali, Colombia.
3Ingeniero sanitario. Magister en Ingeniería, Universidad del Valle, Colombia. E-mail: anfetorres@gmail.com
4Ingeniera sanitaria. Doctora en Ingeniería, Universidad del Valle, Colombia. E-mail: navasari@yahoo.com.mx
5Ingeniera sanitaria, Dra. Profesora Asociada, Facultad de Ingeniería, Universidad del Valle, Cali, Colombia. E-mail: jenny.rodriguez@correounivalle.edu.co
Para citar este artículo / To cite this article
A.F. Torres Franco, N. Vásquez Sarria, and J.A. Rodríguez Victoria, "Performance of novel contact stabilization activated sludge system or domestic wastewater treatment". Ing. Unv., vol. 19, no. 2, pp. 253-266, 2015. http://dx.doi.org/10.1114/javeriana.iyu19-2.pncs
Abstract
A pilot-scale study was conducted to evaluate a traditional contact stabilization activated sludge system (CSASC) and a modified CSAS (CSASM) treating domestic wastewater. The CSASC system was comprised of a contact reactor (CR), a stabilization reactor (SR) and a secondary settler (SS); the CSASM included a second CR, a second SS (CR2 and SS2), and a modified SR (SRM) divided into four zones: an attached-suspended growth zone which allowed the system to reach an average sludge retention time close to 36 d and favored the occurrence of nitrification; an anoxic zone for denitrification occurrence; an aerated suspended growth zone with a high presence of organic carbon; and an additional aerated suspended growth zone with a high ammonia concentrations environment. The CSASC's removal efficiencies of chemical oxygen demand (COD) and total ammonia nitrogen (TAN) were respectively 94±4 % and 53±12%; whereas CSASM's efficiencies were 88±7% for COD and 92±7% for TAN. Concentrations of TAN and NO3--N in the CSASM's final effluent were 14.3±5.2 and 5.0±2.9 mgXL-1; and 4.8±4.4 and 9.1±5.8 mgXL-1 in the CSASM's final effluent. Results demonstrated that the proposed configuration obtained higher nitrogen removal efficiencies than traditional CSAS.
Keywords: activated sludge; contact stabilization; organic carbon; nitrogen removal; domestic wastewater
Resumen
Usando una prueba a escala piloto se evaluó un sistema de estabilización por contacto convencional (CSASC) y uno modificado (CSASM), para el tratamiento de agua residual doméstica. El CSASC estuvo compuesto de un reactor de contacto (CR), un reactor de estabilización (SR) y un sedimentador secundario (SS); el CSASM incluyó la adición de un SR y un SS (CR2 y SS2) y la modificación del reactor de estabilización (SRM), en cuatro zonas: una de crecimiento adherido-suspendido, que permitió alcanzar un tiempo de retención celular promedio cercano a 36 d y que beneficia el desarrollo de la nitrificación; una zona anóxica para el desarrollo de la denitrificación; una zona aireada de crecimiento suspendido, con un ambiente rico en materia orgánica carbonácea, y otra con un ambiente rico en nitrógeno amoniacal total (NAT). El CSASC presentó eficiencias de reducción de química de oxígeno (DQO) y NAT de 94 ± 4 % y 53 ± 12 % respectivamente; mientras que el CSASM presentó eficiencias del 88 ± 7 %, y de 92 ±7 %, respectivamente. Las concentraciones promedio de NAT y N-NO3- en el efluente del CSASC fueron de 14,3 ± 5,2 y 5,0 ± 2,9 mgXL-1, respectivamente, y para el CSASM fueron de 4,8 ± 4,4 y 9,1 ± 5,8 mgXL-1, respectivamente. Estos resultados demostraron que la nueva configuración evaluada presentó mayores eficiencias de reducción de material nitrogenado.
Palabras clave: lodos activados; estabilización por contacto; materia orgánica carbonácea; remoción de nitrógeno; agua residual doméstica
Introduction
Municipal wastewater contains a variety of organic compounds of carbon and nitrogen. Nitrogen material is related not only to organic forms, but to inorganic forms, especially NH.+-N. High nitrogen concentrations in wastewater are associated with eutrophication, which affects freshwater quality. In consequence, conventional wastewater treatment plants aim to reduce concentrations of such compounds in final effluent through secondary and tertiary treatment processes.
Contact Stabilization Activated Sludge system (CSAS) is a variation of the conventional activated sludge system (Figure 1a), where two aerated tanks and a secondary settler are used to remove carbonaceous material concentrations incoming with influent wastewater. The process begins in the Contact Reactor (CR), where influent wastewater is mixed with recirculation sludge from the Stabilization Reactor (SR) to conform the mixed liquor (ML). After a short hydraulic retention time (HRT) in the CR, the ML is conduced to the Secondary Settler (SS), where separation of clarified effluent and sludge occurs. Separated sludge is recirculated to SR to start the process again after a HRT of 2-8 h. The process' conceptual base is to optimize adsorption of carbonaceous material over the floc structure during CR's HRT, which ranges between 0.5-1.5 h, whereas SR is conceived with a longer HRT to metabolize in there substrates previously adhered to floc structure [1]-[3].
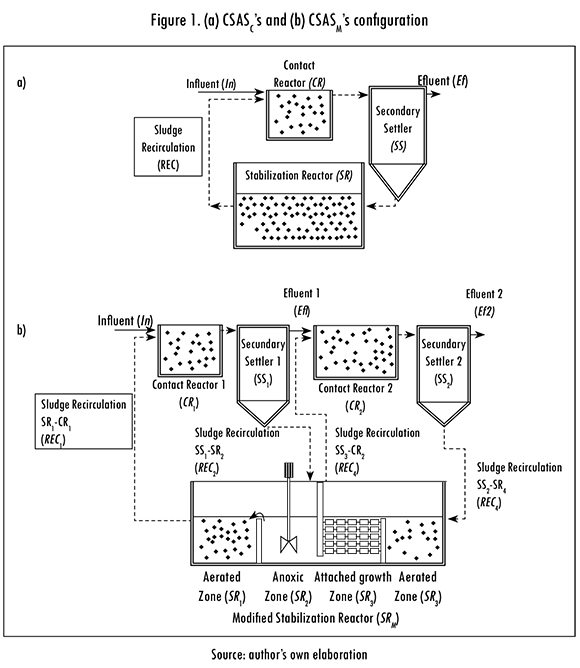
Different experiences have demonstrated the excellent performance of CSAS, with COD removal efficiencies almost always higher than 80% [4], [5], and even higher than 90% for BOD5 [1]. CSAS has been also used as a high-load process for maximizing sludge production in domestic wastewater pretreatment, obtaining COD removal efficiencies of 66±5% [6]. Nevertheless, even though nitrogen transformations are not intended to occur in CSAS, Alexander et al. [7] documented that a Sludge Retention Time (SRT) longer than 4d and high Recirculation Rates (R) favored nitrogen transformations in CSAS systems, whereas other papers have documented nitrification percentages in CSAS systems of 25% [5], 70% [4], and 77% [2]. Furthermore, denitrification, ammonifica-tion, and denitrification can take place in small proportions in CSAS systems [2], [4]. Sludge Retention Time (SRTC) is then a decisive variable to design and control CSAS systems. Eq. 1 shows the expression defined by Alexander et al. [7] for SRT estimation:

Where:
XSR: Volatile suspended solids in the sludge wasted from SR, mgVSSXL-1
QW: Waste flow (L.d-1)
The occurrence of nitrogen transformations in CSAS systems suggest that some modifications could be made to improve nitrogen removal without affecting organic carbon removal efficiencies. Anoxic zones with presence of high organic carbon concentrations can incentivize denitrification if there is an entrance of nitrate coming from nitrification in the SR, which can be stimulated trough hybrid suspended-attached systems [8]-[10]. Currently, recent works in CSAS have proposed system modifications to improve phosphorus removal [11], but no research has been carried out for a nitrogen removal optimization. Considering this background, this study evaluated a CSASC system and a CSASM in order to evaluate nitrogen removal efficiencies.
1. Materials and Methods
1.1. Experimental Unit
The CSAS system presented a typical configuration conformed by a contact reactor (CR), a stabilization reactor (SR), and a secondary settler (SS) (Figure 1a). The CSASM (Figure 1b) was divided into two stages: The first stage (Stage 1) was primary conceived for carbonaceous matter removal and included CR1 + (SS1 + SR1+2), whereas the main purpose of the second stage (Stage 2) was nitrogen removal and included CR2 + (SS2+SR3+4). The stabilization reactor was a unique unit with different compartments that could be described as follows: i) SR1 an aerated zone of suspended growth with a high presence of organic carbon; ii) SR2 an anoxic zone which favored denitrification; iii) SR3 an attached-suspended growth zone which favored nitrification and denitrification; and finally, iv) SR4 an additional aerated zone of suspended growth with a high ammonia concentrations measured as total ammonia nitrogen (TAN) for favored nitrification. The volumes of the treatment unit were 9.98 L for CR1, 36.68 L for CR2, 27.20 for SR1, 8.90 L for SR2, 8.75 for SR3, and 8.75L for SR4. Units were constructed on glass fiber and peristaltic pumps were used for recirculation lines. Aeration in CR1, CR2, SR1, and SR4 was supplied with a blower and bubble diffusers.
Waste Sludge from real-scale "Aguas del Sur" wastewater treatment plant (WWTP) was used for the inoculation of the systems. CSASC start-up phase was performed during 10 d through progressive affluent flow increments, from 50% to 100%. Those increments were conditioned to COD removal efficiencies higher than 60%. Once CSASC operation period was completed, the CSASM start-up phase was begun. Inoculum acclimation and start-up phase were performed during 15d. After this period, SR3+4 (separated from SR1+2 through a division screen) and SS2 were added as a second CSAS for CSASC's effluent post-treatment. After an additional 17 d, the division screen was removed, the four zones inside SRM were established and the CSASM evaluation period was started. System's influent (7n) was taken from "Aguas del Sur" WWTP after screening and then conduced to a primary clarifier with a HRT of 1 h.
1.2. System Control and Evaluation
Samples from mixed liquors, Influent (In), and effluent of CSASC (Ef) and CSASM (Ef2) were taken twice a week. COD, TAN, nitrates (NO3--N), phosphorus (PO4 3-P), pH, and total and bicarbonate alkalinity were measured for influent characterization. Volatile suspended solids (VSSML), pH, and dissolved oxygen (DO) were measured in mixed liquors of CR and SR in CSASC, and CR1, CR2, SR1, SR2, SR3, and SR in CSASM. In order to operate CSASc and CSASM, VSSLM, DO, pH, temperature, food-microorganisms ratio (F/M), and SRT were monitored. COD and TAN removal efficiencies were estimated from influent and effluents concentrations, where NO3-- N and alkalinity were also measured. All parameters were determined as indicated by [12].
Operational conditions evaluated in CSASC and CSASM during the operation were chosen from previous studies in CSAS systems. A SRT range between 6-10 d was chosen to secure nitrification. HRTCR of 0.84 h, HRTSR of 4.10 h, VSSMLCR of 1000 mgXL-1, and VSSMLSR of 2000 mgXL-1, DO concentration of 4.0 mgXL-1 [2], [5], [7]. Wasted sludge was taken from SR and SR1 in CSASC and CSASM respectively. Sludge retention time for CSASC (SRTC) was evaluated according to Eq. 2, according to solids flow in the CSASM, although a global SRT (SRTM-G) was totalized as shown before in Eq. 1; an internal SRT was also established for each stage. For Stage 1, suspended biomass in CR1, SR1, and SR2 and sludge wasted from SR1 define the SRTM-S1 as expressed in Eq. 2.

Where:
S1: biomass sampled from Stage 1
S1: QM, CR1*XCR1 + QM, SR1*XSR1 + QM, SR2*XSR2
QM: Qsampled in each reactor (LXd-1)
QW, SR1: Sludge waste flow from SR1 (LXd-1)
For Stage 2 suspended biomass in CR2, SR3, and SR4 and attached biomass in SR were considered to define SRTM-S2 (Eq. 3). According to CSASM configuration, of SRTM was defined as presented in Eq. 3, by considering attached and suspended biomass. Attached biomass was estimated using the mathematical model of Rit-tmann and McCarty [13] for attached biomass growth (aVJYbt-1), which has been employed in different works related with attac hed-suspended reactors [14], [15], [10]. Kinetic parameters registered in Table 1 and simplified expressions proposed by Fouad and Barghava [15] were used to estimate aVJYbt-1. According to low C/N conditions in SR3, ammonia oxidizing bacteria (AOB) predominated in attached growth and consequently, kinetic constants from AOB were employed to estimate aVJYbt-1. Values in Table 1 were taken from references where a particular estimation of each constant was made, similar physicochemical conditions to SR3 predominated, and low density polyurethane was employed for attached growth. Finally, for the estimation of SRTM-S2 it was considered that sludge flow from SR3 to SR4 constituted a sludge waste from Stage 2. Attached biomass concentration (Xf) was estimated by separating it from media using a Stomacher 400 mL Bag-mixer-400W Interscience®, at 7 strokes for 60s. Separated biomass was diluted in distilled water and VSS were measured finding a value of 7238 mgVSSXL-1.
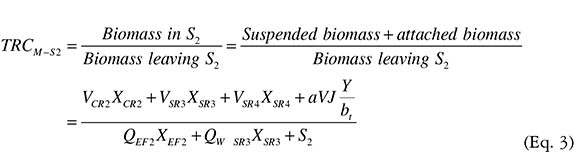
Where:
S2: biomass sampled from Stage 2
S2: (QMCR2 x XCR2) + (QMSR3 x XSR3) + (QMSR4 x XSR4)
QW,SR3: Sludge flow from SR3 to SR2 (L.d-1)
aVJYbt-1: Attached biomass expression
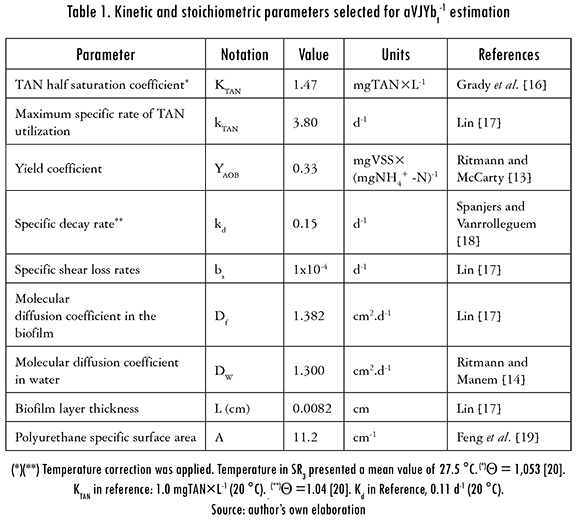
2. Results
2.1. Influent Characterization
After primary clarification, influent was transported to the evaluated systems. Previous research has demonstrated that primary clarifiers do not affect influent nitrogen concentrations and have a positive influence on CSAS's performance because they function as a barrier to fats, oils, and surfactants that affect secondary sludge quality [21]. Influent characteristics are presented in Table 2. Influent variation was conditioned by wastewater production dynamic in "Aguas del Sur" WWTP CSASM's influent was more concentrated than CSASC's, which was influenced by a rainy season. Measured values for both CSASC and CSASM were according to typical ranges of wastewater produced in Santiago de Cali, and guaranteed carbonaceous organic matter, nutrients, and alkalinity availability for biological processes. No influence of wastewater quality variations was detected in the performance of both, CSASC and CSASM, and no statistical differences were noted in influent TAN concentration.
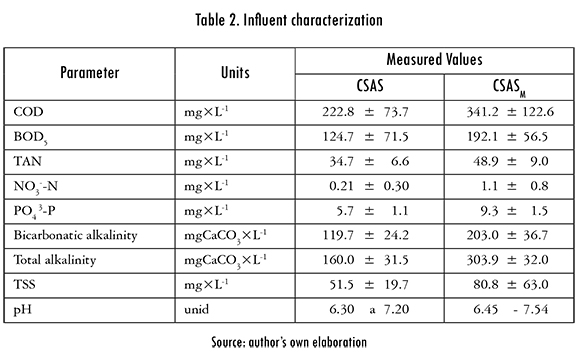
2.2. System Performance and Nitrogen Removal
Operational conditions in CSASC and CSASM are registered in Table 3. Obtained values were according referenced values for CSAS systems where nitrification took place. Additionally, temperature and pH ranges were optimum for nitrification occurrence (pH: 7.5 - 8.0 units, T: 28-36 oC [22]).
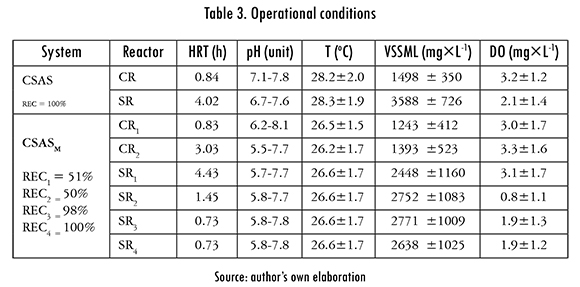
TAN and NO3-- N concentrations in final effluent of CSASC (Ef) were 14.3±5.2 mgTAN XL-1 and 5.0±2.9 mgNO3--N XL-1, whereas concentrations of in CSASM's final effluent (Ef2) were 4.8±4.4 mgTANXL-1 and 9.1±5.8 mgNO3--NXL-1, respectively. Figure 2 presents the variation in the nitrogen compounds concentrations of the final effluent. TAN removal efficiencies were respectively 53±12% and 92±7% for traditional (CSASC) and modified (CSASM) systems.
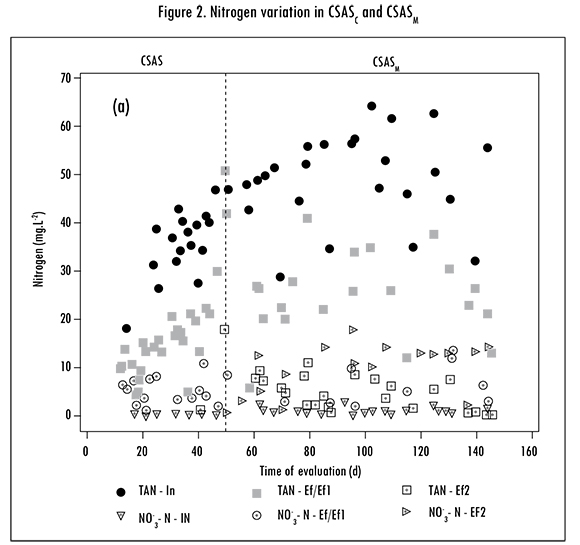
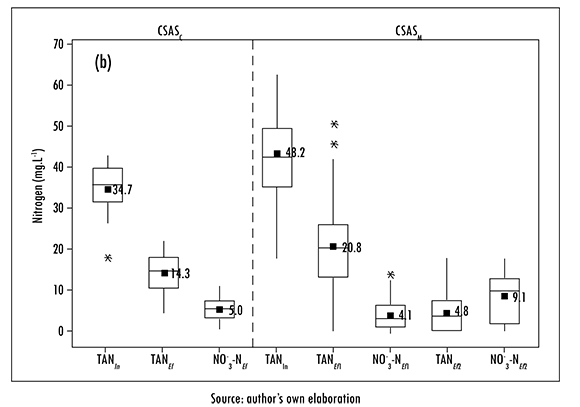
TAN removal efficiencies observed in the traditional system (CSASC) were similar to those previously reported in [1], [4]. Both TAN removal efficiencies and NO3--N concentrations in the final effluent evidenced that nitrification was the main TAN transformation process. Environmental conditions in the contact reactor (CR) and the stabilization reactor (SR) support this idea as an aerobic environment predominated (CR: 3.2±1.2 mgDOXL-1 and SR: 2.1±1.4 mgDOXL-1), inorganic carbon presence supported by total and bicarbonate alkalinity concentrations in influent, and a SRT high enough to enable nitrifier organism growth at process' temperatures. Even when NO3--N concentrations in CSASC's final effluent (Ef) were low, processes of NO3-- N removal like denitrification could have occurred only in low rates because the environmental conditions in CR and SR were predominantly aerobic, however, a possible presence of anoxic microzones and a complete anoxic environment in the bottom of the SS could have promoted incipient denitrification.
The higher TAN removal efficiencies exhibited by CSASM were related to Stage 2 performance. The establishment of high SRTM-S2 was a consequence of attached growth in SR3, which possibly favored the presence of nitrifiers and distribution in CSASM. The measured SRTM-S2 of 20.9±6.7 d which increased the SRTG values up to 36.2±14.2 d are coincident with those reported for attached-suspended growth reactors to favor nitrification performance [8], [23], [24]. The high nitrification rates compared with low NO3-- N concentrations in CSASM's final effluent suggest that processes of NO3--N removal actually took place in CSASM at a considerable rate, which is consequent with SR2's conditions. Low DO concentrations (0.7±1.0 mgXL-1) in the anoxic compartment (SR2) and the permanent entrance of carbonaceous organic matter incoming, with recirculation from SS1 and NO3-- N from the attached growth compartment (SR3), suggest that the main process of NO3-- N removal was denitrification.
An incipient denitrification took place in the bottom of CSASM's SS2. Some sludge resuspension events are evidence of denitrification in the aforementioned zone. Presence of carbonaceous matter from endogenous metabolism and NO3-- N produced in Stage 2, as well as DO absence were conditions that promoted denitrification. Small N2 bubbles produced by denitrification could have risen from the bottom of SS2, thus explaining sludge resuspension events.
Eventual NO3-N concentrations below 2 mgXL-1 measured in the final effluent (Ef2) are evidence that CSASM's conditions can be adjusted in order to optimize denitrification process limited by a high R value in REC3 (98%). Even when NO3-- N were produced in SR3 and SR4, the high R value in REC3 made that a high NO3-- N flow to the CR2 limited its availability in SR2 for denitrifi-cation. Whereas denitrification was affected due to the high R value in REC3, this condition had a positive influence in Stage 2 performance as it controlled biomass waste, determining the SRTM-S2 (20.9±6.7 d). This condition promoted nitrification and high VSSML concentrations that guaranteed the system's operational stability. Results suggest that R value could be adjusted in order to promote denitrification in SR2 and secure the system's operational stability.
Conclusions
TAN removal efficiencies observed for the modified CSAS system (CSASM) were significantly higher (92±7%) compared to traditional CSAS system (CSASC) and NO3-- N concentrations in the final effluent were considerably low (9.1±5.8 mgXL-1). This behavior suggests that the proposed modifications improved nitrogen removal processes in the CSASM. Operational conditions established in both CSASC and CSASM indicated that nitrification was the predominant TAN transformation process. Stage 2 helped CSASM to reach higher nitrification efficiencies in comparison with CSAS, where the main nitrogen removal process in Stage 1 was denitrification, which took place especially in the anoxic compartment (SR2) where adequate environmental conditions for its occurrence were established. Finally, the R value in REC3 must be carefully evaluated in order to allow an abundant flow of NO3-- N to SR2 by the time that operational stability concerning VSSML must be also guaranteed.
A strong relationship between REC3 and SRTM-S2 was found as REC3 controlled biomass waste from Stage 2. The R value of 98% for REC3 promoted a high SRTM-S2 average of 20.9-6.7 d and secured the system's operational stability in terms of SSVML concentrations, with values of 1243±412 mgXL-1 in CR1, 1393±523 mgXL-1 in CR2 and around 2500 mgXL1 in SRM's compartments.
References
[1] W Gujer and D. Jenkins, "The contact stabilization activated sludge process-oxygen utilization, sludge production and efficiency", Water Research, vol. 9, no. 5, pp. 553-560, 1975. [ Links ]
[2] W. Gujer and D. Jenkins, "A nitrification model for the contact stabilization activated sludge process", Water Research, vol. 9, no. 5, pp. 561-566, 1975. [ Links ]
[3] N. Z. Al-Mutairi, F. A. Al-Sharifi and S. B. Al-Shammari, "Evaluation study of a slaughterhouse wastewater treatment plant including contact-assisted activated sludge and DAF", Desalination, vol. 225, no. 1, pp. 167-175, 2008. [ Links ]
[4] R. Al-Sa'ed and O. Zimmo, "Process performance evaluation of the contact stabilisation system at Birzeit University", International Journal of Environment and Pollution, vol. 21, no. 5, pp. 511-518, 2004. [ Links ]
[5] S. N. Vásquez, V J. Rodríguez and L. IP Torres, "Comportamiento del nitrógeno en un sistema de lodos activados en la modalidad de estabilización por contacto tratando aguas residuales domésticas", Afinidad, vol. 67, no. 548, pp. 283-288, 2010. [ Links ]
[6] F. Meerburg, S. Vlaeminck, J. Vercamer and N. Boon, "Turn it up!: High-load Contact Stabilization (HiCS) is a valuable activated sludge process for maximizing sludge production from sewage", in Proc. 2nd IWA Specialist conference on EcoTechnologies for Sewage Treatment Plants (EcoSTP-2014), 2014. [ Links ]
[7] W V Alexander, G. Ekama and G. V R. Marais, "The activated sludge process part 2. Application of the general kinetic model to the contact stabilization process", Water Research, vol. 14, no. 12, pp. 1737-1747, 1980. [ Links ]
[8] C. W Randall and D. SEN, "Full-scale evaluation of an integrated fixed-film activated sludge (IFAS) process for enhanced nitrogen removal", Water Science and Technology, vol. 33, no. 12, pp. 155-162, 1996. [ Links ]
[9] M. Tizghadam, C. Dagot and M. Baudu, "Wastewater treatment in a hybrid activated sludge baffled reactor", Journal of Hazardous Materials, vol. 154, no. 1, pp. 550-557, 2008. [ Links ]
[10] A. K. Thalla, R. Bhargava and P Kumar, "Nitrification kinetics of activated sludge-biofilm system: A mathematical model", BioresourceTechnology, vol. 101, pp. 5827-5835, 2010. [ Links ]
[11] I. A. Hamdy, M. A. Mahmoud, S. Mohamed, O. Alaa and M. Mostafa, "The effects of modification for contact stabilization activated sludge on EBPR", HBRCJournal, vol. 10, no. 1, pp. 92-99, 2014. [ Links ]
[12] APHA. Standard methods for the examination of water and wastewater, 21st ed. Washington: APHA, American Water Works Assoc. and Water Environment Federation, 2005. [ Links ]
[13] B. E. Rittmann and P L. Mccarty, "Model of steady state biofilm kinetics", Biotechnology and Bioengineering, vol. 22, no. 11, pp. 2343-2357, 1980. [ Links ]
[14] B. E. Rittmann and J. A. Manem, "Development and experimental evaluation of a steady state, multispecies biofilm model", Biotechnology and Bioengineering, vol. 39, no. 9, pp. 914-922, 1992. [ Links ]
[15] M. Fouad and R. Bhargava, "A simplified model for the steady-state biofilm-activated sludge reactor", Journal of Environmental Management, vol. 74, no. 3, pp. 245-253, 2005. [ Links ]
[16] C. Grady, G. T. Daigger and H. Elm, "Biological wastewater treatment. Revised and expanded", Environmental Science and Pollution Control Series. Marcel Dekker, 1999. [ Links ]
[17] Y H. Lin, "Kinetics of nitrogen and carbon removal in a moving-fixed bed biofilm reactor", Applied Mathematical Modelling, vol. 32, no. 11, pp. 2360-2377, 2008. [ Links ]
[18] H. Spanjers and P Vanrolleghem, "Respirometry as a tool for rapid characterization of wastewater and activated sludge", Water Science and Technology, vol. 31, no. 2, pp. 105-114, 1995. [ Links ]
[19] Q. Feng, Y Wang, T. Wang, H. Zheng, L. Chu, C. Zhang and X. H. Xing, "Effects of packing rates of cubic-shaped polyurethane foam carriers on the microbial community and the removal of organics and nitrogen in moving bed biofilm reactors", Bioresource Technology, vol. 117, pp. 201-207, 2012. [ Links ]
[20] Metcalf and Eddy. Wastewater engineering: treatment and reuse. New York: McGraw-Hill, 2003. [ Links ]
[21] T. Mañunga, J. A. Rodríguez Victoria and P Torres Lozada, "Tratamiento de agua residual doméstica sin clarificación primaria en un sistema de lodos activados en la modalidad de estabilización por contacto". Revista Científica Ingeniería y Desarrollo, vol. 30, no. 2, pp. 246-260, 2012. [ Links ]
[22] N. Bernet and M. Spérandio, "Principles of nitrifying processes", in Environmental technologies to treat nitrogen pollution, 1st ed. London: IWA Publishing, 2009, pp. 23-37. [ Links ]
[23] F. Çeçen, E. Orak and P Gõkçin, "Nitrification studies on fertilizer wastewaters in activated sludge and biofilm reactors", Water Science and Technology, vol. 32, no. 12, pp. 141-148, 1995. [ Links ]
[24] J. Liu, B. Wang, W Li, C. Jin, X. Cao and L. Wang, "Removal of nitrogen from coal gasification and coke plant wastewaters in A/O submerged biofilm-activated sludge (SBF-AS) hybrid system", Water Science and Technology, vol. 34, no. 10, pp 17-24, 1996. [ Links ]