Introduction
The goal of ecological restoration is recovery to achieve a historical state close to the previous ecosystem of a degraded area that has resulted from human perturbation or natural disturbance (Hobbs et al., 2006). The restoration mechanisms to recover plant composition are categorized according to the degree of human intervention (McAlpine et al., 2016). Dispersion (Shiels and Walker 2003) and seed rain from surrounding forests create the secondary forest in abandoned lands or pastures (Aide et al., 2000), a process known as passive restoration. Active restoration also uses native or exotic species to develop and extend forest plantation (Hall et al., 2011). This plantation could have productive goals such as extract wood or protect hydric resources (Paquette and Messier, 2010). The coverage of secondary forests and forest plantations has increased worldwide (FAO, 2001); there are 300 million hectares of secondary forest in America, whereas there were nearly 60 million estimated hectares of forest plantation in 2015 (FAO, 2015).
Litter decomposition is proposed as an indicator of forest recovery, where soil fauna could be relevant in driving the magnitude and stability of organic matter breakdown in a restoration system (Barreto da Silva et al., 2018). Litter decomposition maintains soil fertility through the transfer of nutrients from plants to soil and vice versa (Guendehou et al., 2014; Patricio et al., 2012; Silver et al., 2014) Moreover, litter maintains the microclimatic conditions of the soil, offers a habitat to invertebrates (Cajaiba et al., 2017; Cole et al., 2016), and participates in filtering and reserving water in the soil (Caldeira et al., 2013).
In addition, soil invertebrates affect litter decomposition through fragmentation that stimulates microorganism colonization (Cole et al., 2016, Fujii and Takeda, 2017), dispersion of fungi propagules (Lilleskov and Bruns, 2005), grazing on microorganisms (Crowther et al., 2012), transformation of litter into pellets stimulating microorganism activity (Coulis et al., 2009; Coulis et al., 2013; Lavelle and Spain, 2001), and increasing nitrogen mineralization (David, 2014). Little is known about biodiversity associated to litter decomposition in an ecosystem under restoration processes. Similarly, there is scarce knowledge on the role of decomposition and decomposer fauna in order to assess restoration efficiency, except for a study on restoration strategies in Costa Rica, where litter invertebrates differ among plantations, nucleation/islands, and natural regeneration; abundance, richness, and diversity are higher in nucleation (Cole et al., 2016).
Among invertebrates, myriapods could contribute to litter decomposition in a restoration ecosystem. Millipedes (Diplopoda) are “litter transformers” (Lavelle and Spain, 2001) that break down the litter, ingest fungi from leaf litter, increase bacterial community, create compact fecal pellets reducing the surface of decomposition, and contribute to soil nutrient dynamics (David and Gillon, 2002; David, 2014). Furthermore, centipedes have a top down effect on collembola, which greatly contributes to leaf litter decomposition (Gunther et al., 2014; Santonja et al., 2018). We designed a litter translocation experiment to assess the myriapod community associated to litter decomposition in a secondary forest (passive restoration) and an Andean alder plantation (active restoration). We selected the most common plant species of each site in order to conduct a decomposition experiment for four months and measured the myriapod richness and abundance. Our goal was to determine the myriapod community associated to leaf litter decomposition in a secondary forest and an Andean alder plantation, and to assess if the myriapod community shifts with leaf litter species, litter exposure time, and restoration strategy. We predicted that plant litter quality and restoration strategy could lead to shifts in litter conditions during the decomposition time period and, therefore, to variations in the myriapod community associated to this ecosystem process.
Materials and methods
Study área
Our study was conducted at the Reserva Natural Rio Blanco y Quebrada de Olivares located in Manizales, Caldas in the Colombian Central Andes (Sanin et al., 2006). The reserve has an area of 4,932 ha between 2,150 and 3,700 m asl, where there are secondary forests and plantations of Alnus acuminata (Kunth) (Fagales: Betulaceae). The area is characterized by an annual mean temperature of 19 ± 3 °C, and a relative humidity of 90%. The annual mean precipitation is 2,500 mm, with most rainfall in two wet seasons: April - May and October -November. In addition, the reserve supplies freshwater to nearby cities, so it is a relevant site for the region (Corpocaldas, 2010).
Design
We selected four plots of 20x20m separated from each other by 300m in a secondary forest (N 5° 4’31.08” - W 75° 26’13.5”; altitude: 2635 m) and four plots in an Andean alder plantation (N 5° 4’8” - W 75° 26’29”; 2467 m). We conducted a litter translocation experiment with the most abundant leaf litter in the Andean alder plantation and secondary forest, Alnus acuminata and Hedyosmum bonplandianum (Kunth) (Chloranthales: Chloranthaceae), respectively. The plant species are similar in initial leaf thickness and leaf area, but differ in leaf dry-matter content; H. bonplandianum has the lowest leaf dry-matter content (mean +/- sd; A. acuminata 660.37+/-209.60; H. bonplandianum 323.17 +/-105.93).
We used 128 decomposition leaf bags of 30 cm x 30 cm and 9 mm aperture to allow the entrance of micro, meso, and macrofauna, for a total of 64 bags per site. We added 5 g of dry plant species to each decomposition bag, according to each treatment. We collected 32 bags per site every 60 days for four months. The myriapods were separated manually from the leaf litter collected; individuals were preserved in 96% ethanol and identified to the morphospecies level using the specialized literature (Adis, 2002; Scheller and Adis, 1996; Shelley and Mercurio, 2005; Triplehorn and Johnson, 2005). We did not include juveniles in the analysis, due to difficulties in the taxonomic identification.
Statistical analysis
We used Generalized Linear Models (GLMs, Crawley, 2007) with restoration strategy, litter species, and litter exposure time as fixed factors, and myriapod richness or abundance as response using the Gaussian error distribution. The models were graphically checked for homogeneity of variance and error distribution. To compare the overall community structure of myriapods associated to litter decomposition among the litter plant species of each restoration strategy, we used a permutational multivariate analysis of variance (PERMANOVA), a non-parametric test that allows to investigate complex models including interactions (Anderson, 2017), with Bray Curtis as a dissimilarity measure and 999 permutations. For site and litter plant species, we used the SIMPER (Similarity percentage) procedure (Clarke, 1993) to identify the contribution of each morphospecies to the measures of BrayCurtis dissimilarity. This allowed us to identify the morphospecies that were most important in differentiating each restoration strategy or litter type from the others. The statistical analyses were conducted in R statistical program (R Core Team, 2018) with the CAR package (Fox and Weisberg, 2011) for the GLM and vegan (Oksanen et al., 2015) for the PERMANOVA analysis.
Results
During the leaf litter decomposition process, we found 275 myriapod individuals that belong to 39 morphospecies. Of the total species, 26 species belonged to millipedes (Diplopoda); Polydesmida was the most abundant and diverse order (Table 1). Our analysis showed that leaf litter myriapod richness and abundance were affected by the restoration strategy, but not by leaf litter species or litter exposure time. The Andean alder plantation showed higher myriapod richness and abundance associated to litter decomposition (Table 2, Figure 1).
Table 1 Taxonomy of leaf litter myriapods in a secondary forest and an Andean alder plantation of a protected area from Central Andes (Manizales, Colombia).
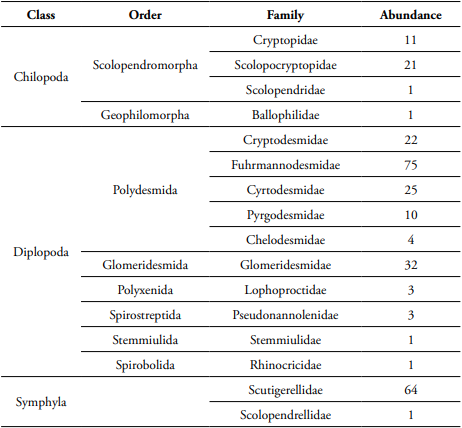
In addition, myriapod composition differed significantly between the restoration strategies and the leaf litter species (Table 2). For instance, Hanseniella sp. (Bagnall) (Symphyla: Scutigerellidae) was dominant in the Andean alder plantation and H. bonplandianum litter, whereas Fuhrmannodesmidae family was the most abundant family in the secondary forest and A. acuminata litter. The morphospecies of Glomeridesmidae and Criptopidae inhabited H. bonplandianum litter of the secondary forest, whereas Pseudonannolenidae, Stemmiulidae, and Cryptodesmidae were exclusive of A. acuminata in the secondary forest. Cryptodesmidae and the genus Newportia (Gervais) (Scolopendromorpha: Scolopocryptopidae) were only found in H. bonplandianum litter of Alder plantation. In contrast, Newportia sp., Scolopendrellopsis sp., and the families Cryptodesmidae, Lophoprotidae, Ballopilidae, Rhinocricidae, and Scolopendridae were only found in A. acuminata litter of Alder plantation.
Table 2 Generalized linear model (GLM) of restoration strategy, litter species, and litter exposure time effects on myriapod richness and abundance. PERMANOVA of restoration strategy, litter species, and litter exposure time effects on myriapod composition. F-value and P-value (in parenthesis with significant values highlighted in bold) are showed.
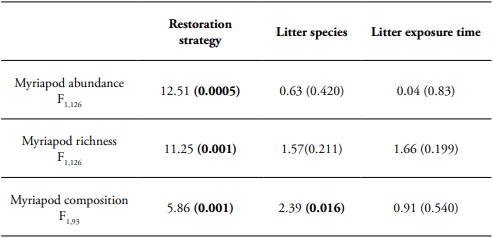
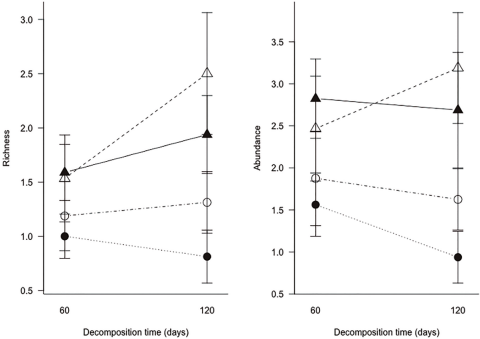
Figure 1 Myriapod richness and abundance of two litter species in a secondary forest and an Andean alder plantation of a protected area across litter exposure time. Secondary forest is represented by a circle, Andean alder plantation by a triangle, H. bonplandianum litter is shown in black, A. acuminata litter in white.
Discussion
In this study, we reported the myriapod richness and abundance associated with leaf litter decomposition in active and passive restoration strategies. Our results showed that classes Diplopoda, Chilopoda, and Symphyla colonized leaf litter of A. acuminata and H. bonplandianum in an Alder plantation and secondary forest. Restoration strategies lead to differences in myriapod richness and abundance, whereas exposure time and litter species do not show any effects. Myriapod composition shifted with restoration strategy and litter species.
We found myriapod families that had been reported in other studies, such as Scutigerellidae, Fuhrmannodesmidae, Chelodesmidae, Cryptodesmidae, and Pyrgodesmidae (Vohland and Schroth, 1999). Millipedes were the most abundance myriapods in both restoration strategies. Millipedes influence leaf litter decomposition (Irmler, 2000) through breakdown and transport of litter remains to a more favorable microhabitat for decomposition and to mobilize nutrients (Pramanik et al., 2001); moreover, millipedes influence microbial density and dispersion (David and Handa, 2010). Symphyla consume the microbial community, which affects leaf litter decomposition (Blakely et al., 2002).
Chilopoda are generalist predators, as they feed on Collembola, Diptera larvae, and Lumbricidae in the soil (Salmon et al., 2005). Cascading indirect effects of generalist predators on litter decomposition have been reported for spider species (Lensing and Wise, 2006). This same pattern could occur with centipedes in the Alder plantation and the secondary forest, which, by consuming springtails, could alter the effects of Collembola on litter microbial activity (Filser, 2002).
Myriapod richness, abundance, and composition shift with restoration strategy. Myriapod richness and abundance were greater in the alder plantation, millipedes were the most abundant and diverse. The same pattern has been found in plantations of native species (Terminalia amazonia and Vochysia guatemalensis) mixed with legume species, Erythrina poeppigiana and Inga edulis, in Costa Rica (Cole et al., 2016). It has also been observed in sites with different regeneration times in Brazil, where sites with low recovery time had high diplopod abundance (Frasson et al., 2016). This could reflect differences in plant composition between sites that could lead to variations in litterfall quality and, therefore, in the resources available for myriapod colonization, as reported for other soil invertebrates (Hansen and Coleman, 1998; Wardle et al., 2006).
Consumption preference of myriapods depends on leaf traits, such as toughness, thickness, and chemical characteristics or microbial activity (Carcamo et al., 2000; Coulis et al., 2013; David and Handa, 2010). The litter species used in this study differ in their initial litter quality, which leads to shifts in myriapod composition, but not in richness and abundance. The latter reflects myriapod species preference to litter species quality; for instance, morphospecies belong to Glomeridesmidae and Criptopidae colonized H. bonplandianum leaf litter. This litter was characterized by big leaves with low leaf dry-matter content, indicating a faster decomposition process and less resistance to physical hazards in comparison to A. acuminata. Those differences could lead to variations in the effect of myriapods on decomposition in response to litter species (Suzuki et al., 2013).
Decomposition time implies loss of leaf resource and changes in leaf traits, leading to shifts in fauna and fungal diversity and replacement of species (Anderson, 1985; Hasegawa and Takeda, 1993; Voříšková and PetrBaldrian, 2013). Despite this, decomposition time did not affect myriapod diversity and composition in the two restoration strategies, which implies that myriapod richness and abundance does not respond to shifts in the litter resource through time.
Restoration strategies produce shifts in the community of myriapods. The Alder plantation showed high myriapod diversity, in particular Diplopoda diversity, which contributes directly and indirectly to the decomposition process. Further research could be focused on assessing the top-down effect of myriapod predators, such as Chilopoda, on the decomposition process, as well as on assessing the litter preference of the most abundant species of millipedes in both restoration strategies.