INTRODUCTION
The tomato, Solanum lycopersicum L., occupies the first place in the list of world vegetable production of the FAO, with a cultivated area of 4,782,753 ha and a production of 177,042,359 tons (FAOSTAT, 2018). The records for Colombia indicate that in 2016, 644,642 tons were produced in 16,642 ha of crops (FAOSTAT, 2018). Among the diseases of economic importance in tomato is the vascular wilt, caused by Fusarium oxysporum f. sp. lycopersici (Sacc.) Snyder and Hansen (FOL) (Carrer et al., 2015), causing losses between 21 and 47% in crop yields at free exposure and under cover (Ramyabharathi et al., 2012; Enespa & Dwivedi, 2014). Despite its importance, Colombia still does not record data on losses caused by the disease (Vásquez-Ramírez & Castaño-Zapata, 2017).
Fol causes root and basal rot with deterioration of the stem, causing the wilting of tomato plants (Poussio et al., 2018). The control of the disease is carried out by planting resistant cultivars or by using chemical fungicides. However, these strategies have various limitations such as adaptation of the pathogen to new varieties, deleterious effects of chemical compounds on the beneficial microbiota of the soil, human and animal health, in-crease in production costs and environmental pollution (Hariprasad et al., 2011).
The search for new control alternatives that complement the genetic resistance in tomato is one of the current priorities in the management of the disease. In this sense, biological control is a strategy that can be integrated into current control schemes. Among the most researched microbial groups for their potential agricultural applications is the Plant Growth Promoting Rhizobacteria (PGPR). One of the characteristics that make this microbial group promising is the antagonistic effect that they express against pathogens (Gouda et al., 2017). The antagonism can be given by direct or indirect mechanisms or, in some cases, by a combination of both. The indirect mechanism occurs when bacteria can induce a systemic response in plants, through which enzymes such as peroxidases, phenyl ammonia, endoglucanases and chitinases are expressed in all plant tissues, allowing the plant to defend against pathogens (Kumar et al., 2015). In the mechanism of direct antagonism, bacteria produce compounds such as lytic enzymes (endoglucanases and chitinases), siderophores, antibiotics or volatile compounds that can inhibit the growth of the pathogen (Fridlender et al., 1993; Compant et al., 2005; Kumar et al., 2015), whose effects are evidenced through the inhibition of germination and sporulation of pathogens, competition for nutrients, or through parasitism or mycophagy (Leelasuphakul et al., 2008; Nivya, 2015).
The hyper-parasitic activity of the bacteria is due to the production of lytic enzymes such as hydrolases, whose action mechanism is to degrade the cell wall of pathogens (Shafi et al., 2017). The production of chitinases in Serratia plymuthica has been recorded to inhibit the germination of spores of Botrytis cinerea (Frankowski et al., 2001). The ability to secrete chitinases by Serratia marcescens and Streptomyces sp. is the most important mechanism for these microorganisms to act as antagonists against Sclerotium rolfsii (Ordentlich et al., 1988) and Fusarium oxysporum f. sp. cucumerinum (Kim et al., 2012), respectively. The production of extracellular chitinases and endoglucanases by Pseudomonas stutzeri has also been reported to lyse the mycelium of F. solani (Lim et al., 1991); however, chitinolytic activity is not the only mechanism in bacteria such as S. plymutica with antagonistic activity against Sclerotinia sclerotiorum and B. cinerea, since they are involved proteases and other bio-logical control mechanisms that have greater antagonistic effect (Kamensky et al., 2003. It has also been reported that the p 1.3-glucanases synthesized by Paenibacillus sp. and Streptomyces sp., lyse the cell walls of F. ox-ysporum f. sp. cucumerinum, Rhizoctonia solani, S. rolfsii and Pythium ultimum (Fridlender et al., 1993).
The exploration and exploitation of the biotechnological potential of autochthonous PGPR is an alternative in the development of bio-inputs that complement the nutrition of crops, the improvement of soils, the defense against pathogens and the reduction of the enormous environ mental impact represented by the indiscriminate use of biocides and agrochemicals, which will allow the establishment of sustainable agricultural systems that are efficient and environmentally friendly (Vejan et al., 2016).
The present study evaluated the antagonistic potential of isolates of PGPR recovered from autochthonous agroecosystem cultures, with the perspective of evaluating them in the induction of resistance and control of vascular wilt in tomato.
MATERIALS AND METHODS
Isolates and culture conditions
The isolates of the fungus F. oxysporum f.sp lycopersici were obtained from tomato crops with signs and symptoms of vascular wilt (M4N, M3MN, COMBIA). The pathogenicity of the fungi was verified through the postulates of Koch (Breitschwerdt et al., 2013). Regarding the PGPR, the Biological Research Group of the Catholic University of Manizales and the Food and Agro-Industry Group of the University of Caldas have 52 isolates obtained from different crops (carrot, tomato, sugar cane) which were used to determine their potential to promote growth in tomato and its antagonistic capacity against Fol as a primary screening. This screening allows to select the 10 best isolates based on the response to the antagonistic activity against Fol.
Dual tests
The PGPR were subjected to a first screening based on dual tests to determine their antagonistic potential against Fol. three isolates of Fol (M4N, M3MN, COM-BIA) were evaluated, with three repetitions per test.
The antagonistic activity was determined in PDA according to the methodology described by Rivera et al. (2012). In the center of a Petri dish containing the culture medium, a 5mm disc was inoculated with Fol mycelium and the bacterium was seeded by straight striation parallel to each side of the fungus (approximately 2 cm away). The bacterium was inoculated from a suspension of 1010 CFU / mL concentration. The cultures were incubated at 28 ± 2°C for 8 days, after which the fungal growth radius (R2) was measured, with which the Inhibitory Efficacy Index (IEI) was calculated (Asha et al., 2011) (Equation 1). The control was a Petri dish with inoculation of the fungus in the medium, in the absence of PGPR, in which the growth radius of Fol (R1) was determined.
The ten best isolates were selected, which presented an IEI higher than 0.7 against Fusarium oxysporum f.sp lycopersici; likewise, the isolation of Fol with the lower IEI was selected to continue its evaluation in the subsequent trials. The inhibition of Fol germination was evaluated with these isolates.
Inhibition of FOL sporulation and germination
Individual mixtures were prepared by triplicate in Potato Dextrose Broth (PDB) with each PGPR isolate (1010 cells / mL) and Fol (106 spores / mL). For determination of sporulation percentage inhibition (% ISp), FOL spores (Sp) were collected from a pure culture and co-cultured with bacteria selected above in PDB supplemented with Tween 80 to 0.05% v/v and spores (Sp) count were per-formed in Neubauer's chamber (Equation 2).
The percentage of Fol germination was determined in the prepared mixtures, evidenced through the formation of the germ tube. Three aliquots of 10 µL were placed in Petri dishes with water agar and incubated at 28 ± 2 °C for 48 hours (modified from Vélez et al., 1997). The percentage of inhibition of Fol germination (% IG) of each PGPR isolate was reported from the germinated spore (GSp) count in 100 spores evaluated (100 Sp). The results were compared with the readings obtained in the control of the fungal suspension without bacteria (Equation 3).
Index
From the 10 isolates evaluated, a second screening was carried out in order to select five isolates by applying a Selection Index (SI) calculated based on the variables: indoleacetic acid (IAA), acetylene reduction activity (ARA), solubilization efficiency of tricalcium phosphate at 24, 48 and 72 hours (EFT24, EFT48 and EFT72), percentage of Fol germination at 6 and 12 days (%IG6d and %IG12d) and finally by the percentage of Fol sporulation (%ISp) (Equation 3).
In the five isolates selected, the production of enzymes involved in direct antagonism mechanisms, such as the production of chitinases and 1.3-endoglucanase, were evaluated.
Evaluation of the production of chitinases qualitative method
The isolates were seeded in a medium prepared with chitin as the sole carbon source (20.0 g / L of agar, 4.0 g / L of colloidal chitin, 0.7 g / L of K2HPO4, 0.5 g / L of MgSO4.7H2O, 0.3 g / L of KH2PO4, 0.01 g / L of FeSO4.7H2O, 1.26 g / L of MnSO4, 0.001g / L of ZnSO4.7H2O, pH 7.2 ± 0.2). The degradation of chitin was determined by the appearance of hydrolysis halos in each colony (Rodas et al., 2009). The readings were made daily for 10 days.
The colloidal chitin used in the test was prepared by diluting 40 g of chitin in 400 mL of concentrated HCl and placed in constant agitation (150 rpm) for 2 hours, then 2 liters of cold distilled water were added and the mixture was stirred rapidly to facilitate the formation of the chitin precipitate. The resulting solution was filtered to obtain the colloidal chitin paste to which successive washes were made with distilled water (200mL) to eliminate acid residues. The paste obtained was sterilized for 15 min at 1 5 psi and stored at 4 °C until later use (Wu et al., 2009).
Chitinase activity
A colony of each isolate was seeded in triplicate in 500 µL of minimal medium with 1% colloidal chitin; the cultures were incubated at 30 °C at 150 rpm for 24 hours. The culture was centrifuged at 8000 rpm for 10 minutes and 10 µL of the supernatant were taken as the enzyme source for the enzymatic reaction. In the determination of the chitinase activity, 10 m± of the colloidal chitin sub-strate were added from a stock of 100 mg / mL in phosphate buffer (pH 6.0; 0.05 M). The reactions were incubated at 37 °C for 30 minutes and the activity expressed in mg / mL of glucose released in the reaction was deter-mined by the Miller method (1959) using the DNS rea-gent with measurements at 540 nm.
Activity 1,3 - endoglucanase
A colony of each isolate was seeded in triplicate in 500 m± of minimal medium with 1% glucan (Laminarin SigmaO SLBN-6272V); the cultures were incubated at 30 ° C at 1 50 rpm for 24 hours. The culture was centrifuged at 8000 rpm for 10 minutes and 10 m± of the supernatant were taken as the enzyme source for the enzymatic reaction. In the determination of the endoglucanase activity, 10 µL of the laminarin substrate were added from a stock of 100 mg / mL in acetate buffer (pH 5.0). The reactions were incubated at 37 °C for 30 minutes and the activity expressed in mg / mL of glucose released in the reaction was determined by the Miller method (1959) using the DNS reagent with measurements at 540 nm.
Statistical analysis
An analysis of variance was performed using the SAS GLM procedure (SAS Institute, Cary, USA) to determine the existence of significant differences between the treatments in the variables evaluated. The comparison of the averages was made with the Duncan test (p value <0.05).
RESULTS
Dual tests
The results of the IEI were mostly less than 70%; there-fore, the two best isolates were selected for each statistical group of Duncan, taking into account additionally their behavior in the production of indoleacetic acid (IAA) and the acetylene reduction activity (ARA) (data not shown) (Table 1). The selected isolates came from sugarcane (three) and tomato (seven) crops. As for the Fol fungus, the COMBIA isolate that belonged to the Duncan group with lower IEI was selected; additionally, it presented a greater sporulation in comparison with the isolation M3MN (Table 2).
Table 1 Isolates of PGPR selected by the IEI obtained in the dual tests.
Isolate PGPR | Microorganism | IEI* | Duncan** | Cultivar origin |
---|---|---|---|---|
GIBI139 | Serratia grimesii | 0.77 | A | Tomato |
GIBI137 | Serratia marcescens | 0.71 | B | Tomato |
GIBI419 | Burkholderia cepacia | 0.69 | B | Tomato |
GIBI031 | Cluconacetobacter sacchari | 0.63 | C | Sugar cañe |
GIBI014 | Cluconacetobacter sacchari | 0.63 | C | Sugar cañe |
GIBI130 | Burkholderia trópica | 0.54 | D | Tomato |
GIBI119 | Serratia marcescens | 0.51 | D,E | Tomato |
GIBI001 | Pantoea dispersa | 0.40 | L | Sugar cañe |
GIBI127 | Serratia marcescens | 0.18 | N | Tomato |
CIBUI 6 | Stenotrophomonas maltophilia | -C.07 | S | Tomato |
* Average value obtained at 6 and 12 days. ** Different letters indícate significant differences in the comparison of averages with the Duncan test (value of p <0.05).
Table 2 Index of Inhlbltory Efficacy of the Fol Isolates by the effect of the PGPR evaluated.
Isolate Fol | IEI | Duncan* |
---|---|---|
M4N | 0.44 | A |
COMBIA | 0.40 | B |
M3MN | 0.39 | B |
* Different letters indicate significant differences in the compari son of averages with the Duncan test (value of p <0.05).
Inhibition of Fol germination
The results obtained in the determination of the IG% of Fol (isolate COMBIA selected in the previous test) by the PGPR evaluated are shown in table 3, in which values between 29 and 60% were found. The isolate GIBI127 inhibited 59.29 ± 2.46% of the germination of the spores of Fol, standing out from the other isolates. The lowest percentage of IG was presented with the isolate GIBI001, which was statistically different from the others.
Table 3 Percentage of Inhibition of Fol Germination by the PGPR evaluated (24 hours of evaluation).
Isolate PGPR | %IG | Duncan* |
---|---|---|
GIBI014 | 50.34+5.89 | B |
GIBI137 | 51.73+3.92 | AS |
GIBI127 | 59.29+2.46 | A |
GIBI119 | 5322+2.37 | AB |
GIBI139 | 57.32+3.44 | AB |
GIBI130 | 54.0 8± 12.90 | AB |
GIBI031 | 49.97+1.78 | B |
GIBI416 | 31.92+2.81 | C |
GIBI419 | 51.43+14.82 | B |
GI3I001 | 29.94+8.37 | C |
* Different letters indicate significant differences in the comparison of averages with the Duncan test (value of p <0.05).
Selection Index
After the application of the Selection Index, the isolates GIBI014, 137, 139, 031 and 419 were chosen. In the SI, each variable had a percentage of representativeness as follows: IAA 12%, ARA 12%, efficiency of solubilization of tricalcium phosphate at 24, 48 and 72 hours with 2% for each time, percentage of Fol IG n at 6 and 12 days with 20 and 15% respectively and percentage of Sporulation of Fol with 35% (Table 4).
Table 4 PGPR Isolates chosen based on the Selection Index.
Isolate | SI |
---|---|
GIBI014 | 0.709 |
GIBI137 | 0.534 |
GIBI139 | 0.170 |
GIBI031 | 0.141 |
GIBI419 | -0.107 |
Enzyme production
In the qualitative evaluations for the determination of chitinase, no haloes of enzymatic activity of the evaluated bacteria were observed. The quantitative determination of the enzymatic activity was evidenced by the amount of sugar (glucose) released as a product of the reaction, with the isolates GIBI419 (Burkholderia cepacia) and GIBI031 (Gluconacetobacter sacchari) being the ones in which an increased production of chitinases was observed (0.37 mg / mL ± 0.02 and 0.36 mg / mL ± 0.02 respectively). These treatments were statistically different to the following group in which are included the isolates of Serratia marcescens, S. grimessi (GIBI137 and GIBI139), and G. sacchari (GIBI014), with activities of 0.30 mg / mL ± 0.01, 0.27 mg / mL ± 0.01 and 0.31 mg / mL ± 0.01, respectively. Significant statistical differences were found for the endoglucanase activity in all the isolates evaluated, with the isolation GIBI419 (Burkholderia cepacia) being the one in which the highest enzymatic activity was observed and GIBI031 the one in which the lowest activity was observed (Figure 2).
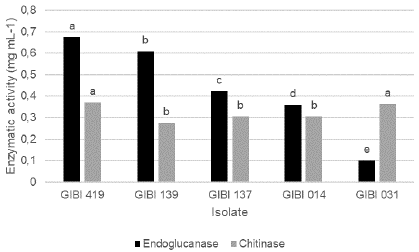
* Different letters indicate significant differences in the comparison of averages with the Duncan test (value of p <0.05).
Figure 2 Production of lytic enzymes endoglucanases and chitinases by the PGPR selected. Enzymatic activity measured as the mg / mL of sugar released in the reaction with DNS (dinitrosalicylic acid).
DISCUSSION
The pathogenic microorganisms that inhabit the rhizosphere cause disease and sometimes lead to total loss of cultures. For the biological control of these diseases, different microorganisms have been used, such as plant growth-promoting rhizobacteria (Raza et al., 2016). The PGPR require colonizing the root system, to perform an efficient biological control that favors plant growth. This has been inferred through the study of natural soil populations, which predominate in the rhizosphere with the ability to colonize young roots (Moreno et al., 2018). For these reasons, it is important to study native isolates to know their characteristics and identify their potential in the development of microbial inoculants either with individual microorganisms or in consortium.
The results obtained allowed to select three isolates from sugarcane and seven from tomato. The isolates belong to the genera Serratia (four), Burkholderia (two), Gluconacetobacter (two), Pantoea (one) and Stenotroph-omonas (one), being genera recognized in different studies for their potential as promoters of plant growth (Santoyo et al., 2016). For instance, the antagonism against Fusarium sp. has been evidenced in studies with Burkholderia cepacia in cultures of the pathogen originating from gladiolus (Toledo et al., 2002), rice and corn (Trujillo et al., 2007).
Table 5 Production of lytic enzymes from the five isolates selected by the SI.
Chitinases | Chitinases | Endoglucanases | |
---|---|---|---|
Isolate | Activity halo | mg/mL | mg/mL glucose |
GIBI014 | 0 A* | 0.31 ±0.01 B | 0.36 + 0.020 D |
GIBI031 | 0 A | 0.36 ± 0.02 A | 0.15 + 0.003 E |
GIBI139 | 0 A | 027 + 0.01 B | 0.60 + 0.004 B |
GIBI137 | 0 A | 0.30 + 0.01 B | 0.42 + 0.060 C |
GIBI419 | 0 A | 0.37 ± 0.02 A | 0.67 + 0.001 A |
Different letters indicate significant differences in the comparison of averages with the Duncan test (value of p <0.05).
The response in the IG% was better with the isolation GIBI127 (Figure 1), where the bacterium Serratia marcescens was recovered from a tomato crop, in contrast to the results obtained with Pantoea dispersa (GIBI001), which comes from sugarcane. Possibly the PGPR recovered from tomato could favor the antagonistic effect against the pathogen, being Fol a specific pathogen of the crop, in comparison with the bacteria isolated from sugarcane. This is a hypothesis that should be subjected to a specific test, since it has been recognized that, in the rhizosphere, the microbial community is affected by the exudates produced by the root of the plant; likewise, the species and diversity of the plants affect the composition and the role that the microbial community in the rhizosphere must fulfill (Glick, 2014; Schlatter et al., 2015; Raza et al., 2017). Additionally, it is important to note that the beneficial effect produced by certain species of PGPR cannot be generalized for all crops. It is recommended to carry out PGPR studies in different crops to determine the response according to the plant. In this manner, products with a combination of microorganism biocontrol agents can be formulated with an adequate scientific basis (Moreno et al., 2018). Despite of S. marcescens GIBI127 was one of the best bacteria for % IG and IAA production, this was not selected for the final five isolates. This can be explained with isolate performance in the index components such as phosphorous solubilization and ARA, that had a combined weight of 18%, and that was a high priority in this study because PGPR characteristics such as nitrogen fixation and phosphorous solubilization are important in order to develop a biocontroller and biofertilizer for FOL in a near future. Moreover, bacteria GIBI137 and GIBI139, that belongs to Serratia genus too, had shown similar behaviors in the other index components leading to take decision of not to include that isolate in downstream analysis.
Chitinase is the enzyme involved in the degradation processes of the cell walls of pathogenic fungi, whose main component is chitin. Many phytopathogens not only contain chitin in their cell wall but also may contain 1.3 glucan, and the enzyme involved for the degradation of this compound is called endoglucanase or laminase (Fridlender et al., 1993). Therefore, when confirming the endoglucanase activity in the five selected isolates, it can be inferred that the enzymes that are intervening in the cell wall degradation of Fol as a direct mechanism of antagonism are both chitinase and endoglucanase enzymes.
It has been reported that the inhibition of germination and sporulation can occur by the secretion of lytic enzymes or by the production of volatile compounds (Kai et al., 2007; Leelasuphakul et al., 2008). Pseudomonas stutzeri secretes chitinase enzymes and extracellular laminarinases to lyse the mycelium of F. solani (Lim et al., 1991). Chitinolytic activity seems to be less essential in bacteria such as S. plymutica in its antagonistic activity against Sclerotinia sclerotiorum and Botrytis cinerea in which proteases and other biological control mechanisms are involved (Kamensky et al., 2003). It has also been reported that the p 1.3-glucanases synthesized by Paenibacillus sp. and Streptomyces sp. lyse the cell walls of F. oxysporum f. sp. cucumerinum, R. solani, S. rolfsii and Pythium ultimum (Fridlender et al., 1993).
CONCLUSIONS
The success of microbial inoculants that allow a long-term reduction of the chemical products used for the reduction of vascular wilt disease caused by Fusarium oxysporum depends on the selection of efficient native strains in each type of soil, with the ability to colonize the rhizosphere without losing its biological activity in search of sustainable, efficient and environmentally friendly agriculture. Therefore, the five best isolates of PGPR were selected according to their antagonistic potential against Fol, under in vitro conditions, by means of dual tests and inhibition of germination, and by the best behavior for the promotion of plant growth, as candidates for the biotechnological development of bio-inputs.