INTRODUCTION
Fires, both those of natural and anthropogenic origin, are one of the most aggressive and sudden disturbances that can affect ecosystems, strongly depleting and modifying their structure and composition, and their impact can be reflected both locally and globally (Peña & Ulloa, 2017; Montiel et al. 2021; Smichowski et al. 2021; Díaz et al. 2023). Their manifestation, especially those of large magnitude, affect the natural evolution of ecosystems directly, and biogeochemical cycles, local climate and other variables indirectly (Crutzen & Andreade, 1990; Hoffmann et al. 2003). These can eliminate a large portion, or even all, of the protective vegetation cover, with the consequent loss of soil aggregates, greatly increasing soil erodability (Neary et al. 2005). In addition, they can cause a slower recovery of many plant species in different strata, generating greater accumulations of coarse fuels and increasing the severity of future fires (Lentile et al. 2006; Botella-Martínez & Fernández-Manso, 2017).
However, the consequences of this type of disturbance are not always negative, since in many regions it plays a key ecological role by contributing to ecosystem balance (Ferrelli, 2023; Kunst et al. 2015). In turn, in certain regions fire is used as a tool for vegetation management and clearing (Smichowski et al. 2021). As mentioned by Soares et al. (2021), in some cases sexual reproduction of plants is favored by enhancing post-fire flowering or facilitating fruit spreading, releasing seeds (Lamont et al. 2019), as long as they can survive the fire event (Cirne & Miranda, 2008).
The effects of fire on germination appear to differ among fire-prone habitats. A growing number of species from fire-prone neotropical ecosystems have shown little or no fire-related effect on increased germination; however, heat tolerance has been noted as a crucial trait for surviving fire, rather than rupture latency (Zupo et al. 2021). On the contrary, as the authors state, temperate ecosystems, mainly Mediterranean vegetation, usually present species that show fire-stimulated germination.
Following a fire, there are direct effects on the ecosystem that include loss of organic matter and changes in inorganic matter, as well as on the short and medium term survival potential of vegetation (Guillem-Cogollos et al. 2017). This damage linked to high burn severity can even affect instantaneous energy fluxes (Sánchez et al. 2009) and their resilience (Paula et al. 2009; Tessler et al. 2014), leading ecosystems to irreversible stages, causing a loss of environmental ecosystem services (Gómez-Sánchez et al. 2017). In areas where the severity has been low or even moderate, trees will remain alive but weakened, leaving them exposed to invasive organisms, which can lead to pest outbreaks and the entry of invasive species (Gibson & Negrón, 2009; Botella-Martínez & Fernández-Manso, 2017). On the other hand, they also have an impact on greenhouse gas concentrations, loss of animal biodiversity, as well as triggering damage to people, infrastructure and the economy (Omi, 2005; Chuvieco, 2008; Botella-Martínez & Fernández-Manso, 2017). For all these statements, it is important to consider that fires can also be natural and that they occur worldwide in a certain season and will continue to occur; therefore, their permanent monitoring and study are important.
In recent years, fires have been recurrently manifested in South America, and the Argentine territory is an example of this (Delegido et al. 2018; Smichowski et al. 2021). During 2020, fires were recorded in 22 of the 23 provinces that comprise the Argentine national territory (MAyDS, 2020). The province of Corrientes was affected by a large number of fire outbreaks distributed throughout the territory, with around 20 % of the peri-urban area of the city being burned (Smichowski et al. 2021).
Undoubtedly, these would be some of the examples that allow us to take back possible future scenarios associated with climate change, whose repercussions on a local scale have high levels of uncertainty. Therefore, it is essential to have accurate information obtained in the shortest possible time, if there is a need for measures to mitigate imminent socio-environmental risks. In this context, it is essential to have a mapping of burned areas to understand the trends over time in terms of the degree to which fires affect a given territory, as well as to facilitate their prevention (-Botella-Martínez & Fernández-Manso, 2017). Undoubtedly, this is a very relevant point in risk management, especially when trying to cover large extensions or where accessibility to certain locations is limited.
For several decades, fire ecologists and managers have used remote sensing, including photointerpretation, to geographically locate both old and active fires to obtain their frequency and extent (Díaz-Delgado & Pons, 1999). The various technologies developed in the field of spatial remote sensing currently allow rapid detection and quantification of the areas affected by fire (Mattar et al. 2012). The great spatial coverage capacity and high frequency of observation provided by satellite sensors, together with the speed with which data can be obtained, make them a valuable resource for fire detection and mapping (Chuvieco, 2008).
In this regard, perimeter delimitation of burned areas (Key & Benson, 2006), studies on vegetation recovery after a fire (Díaz-Delgado & Pons, 1999), establishment of the number of active fire hotspots (Díaz et al. 2023), are some of the possible applications offered by remote sensors for the analysis of fires in order to establish fire management strategies.
Within this range of applications of remote sensors to the study of fires, one of the main ones is related to the severity of burned areas, defined as the damage caused by the passage of fire expressed by the magnitude of the ecological change caused (Key & Benson, 2006). It is considered, in turn, as a descriptor of the environmental impact of fire and a determining factor in explaining ecosystem response (Lentile et al. 2006).
The post-fire severity level can be determined by several procedures, although remote sensing is the only method capable of providing acceptable estimates at a regional scale and in the required time (Martínez et al. 2017). Through the application of the dNBR index, based on the calculation of the NBR (Normalized Burn Ratio) before and after the fire, it is possible to distinguish which areas were the most and least affected by the fire (Key & Benson, 2006).
The response of the vegetation will depend on the severity of the fire on it (Frolking et al. 2009; Jin et al. 2012; Veraverbeke et al. 2013), which is a consequence of the intensity of the burn (temperature and duration of the fire). This factor, together with the environmental characteristics of the burned areas (vegetation type, soil chemical composition, meteorology and plant reproduction strategy) determine the resources affected and the foreseeable time during which these resources will not be available (Pausas, 2012). Their quantification is critical for the rehabilitation of burned areas, since, from the determination of the effect of fire, it is also possible to study the differences in regeneration after its manifestation. The more severe the calcination of biomass, the more severe the impact on ecosystem resilience, i.e., its capacity to return to its pre-disturbance state (De Santis & Chuvieco, 2007; Chen et al. 2011). On the other hand, this is also important in the control of hydrological and erosive risk (Robichaud et al. 2000).
On the basis of the aforementioned, it is important to have operational techniques that allow estimating the severity of fire impact and enable managers to better plan their post-fire actions. Therefore, the purpose of this work is to apply remote sensing tools to delimit the areas affected by the fires that occurred in the Iberá reserve during the first months of the year 2022 and to determine the different levels of severity.
MATERIALS AND METHODS
The Iberá macrosystem is one of the most important subtropical wetlands in the biosphere in terms of its extension and the species that inhabit it, both animal and plant. It comprises a complex water system composed of lentic and lotic environments, made up of small shallow lakes, marshy environments locally known as estuaries or glans, depending on their extension or permanence of their waters and even intricate river courses (Neiff, 2003).
Located in the upper basin south of the Paraná River and entirely within the province of Corrientes, it covers an area of 13,000 km2 (Neiff, 2001), making it the second largest wetland in South America after the Pantanal in Brazil. It is characterized by a humid subtropical climate, with average temperatures of around 21 °C, with maximum temperatures of over 40 °C and a windchill factor of 50 °C. Due to its extension, annual rainfall is in the order of 1,200 to 1,700 mm with an increasing gradient from SW to NE (Contreras & Ojeda, 2016). Although there is a significant reduction in rainfall amounts during the winter season, abundant precipitation is recorded from spring to autumn, with maximums in the latter, mainly in the months of April and May.
The Iberá macrosystem was declared a Provincial Natural Reserve in 1983 by Act 3771 (Honorable Cámara de Diputados, 1983), with the purpose of conserving the natural heritage of the Provincial State, which generated a paradigm shift between biodiversity conservation and economic production. It should be noted that, due to the large floodable extensions, Iberá was considered a region of unproductive lands, dominated by extensive cattle raising.
Within the Iberá Reserve (IR), there is the "Gran Parque Iberá" of 7,680 km2, of which 6,000 km2 belong to the province, while the remaining 1,680 km2 belong to the national government. Gran Parque Iberá was constituted in 2015 through the donation of land, both public and private; and from this moment on, this region had a strong growth in tourist activity, which is enabled throughout the year.
In this context and considering the difficulty to enter the IR, there are currently portals that are access routes to the different protected areas within the reserve. In this research we propose to work with the areas of Cambyretá, San Nicolás / San Alonso, Carambola and Rincón del Socorro, which are part of the Gran Parque Iberá and have different levels of management both provincial and national administration (Figure 1).
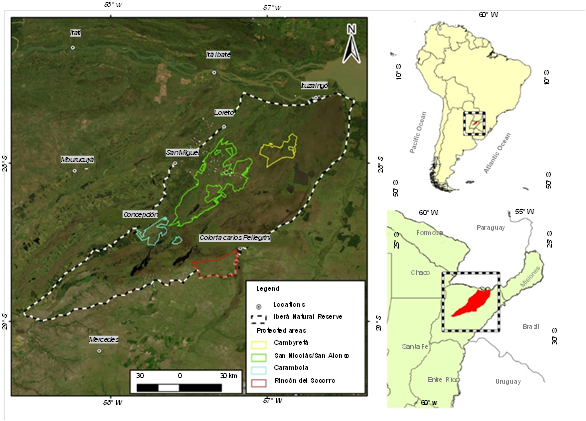
Figure 1 Location of the Iberá Natural Reserve and Portals (province of Corrientes, Rep. Argentina).
For the analysis of burned areas, Sentinel 2A images were used in the Google Earth Engine platform, which are orthorectified and corrected with surface level reflectance (https://developers.google.com/earth-engine/datasets/catalog/COPERNICUS_S2_SR_HARMONIZED). The pre-fire image (initial condition) corresponds to December 2021, while an image from the end of February 2022 was selected as the post-fire image. The dates were chosen based on the record of the beginning of the fires and their prolongation over time, which were obtained through optical analysis of raw images, journalistic information and information from key informants.
The NBR index was calculated on both images (Equation 1), which is obtained by operating on the NIR (Near infrared) bands, whose reflectivity decreases due to the disappearance of active vegetation, and the SWIR (Short-wave Infrared), in which reflectivity increases due to moisture loss, greater soil exposure and lower shadows cast by the vegetation (Escuin et al. 2008; Gómez-Sánchez et al. 2017; Guillem-Cogollos et al. 2017; Chuvieco, 2002).
The operation yields result between -1 and 1, and takes low values for areas affected by fires, while for unaffected areas it acquires high values (Key & Benson, 2006; Anaya et al. 2018). Once the NBR indexes were obtained for the pre- and post-fire images, the dNBR was calculated using equation 2.
The dNBR values are based on the temporal change in reflectance of pre- and post-fire images, correlated with the magnitude of environmental change caused by fire (Arellano Pérez et al. 2017). These were classified considering the criteria of Key & Benson (1999), where it is stated that negative values correspond to unaffected or recovered areas after the fire, while positive values correspond to burned areas. As the study consisted of analyzing burned areas, only values greater than 0,1 according to the classification proposed by Key & Benson (1999) were used (Table 1).
After obtaining the data, total burned areas and burned areas by severity levels were calculated for the Iberá Reserve as a whole and for the four portals, respectively Table 2.
RESULTS AND DISCUSSION
Using the combination of RGB = 12-8-3 Sentinel 2A bands, a first approximation of the affected areas within the Iberá Reserve was made, which allowed us to compare the pre-fire (Figure 2a) and post-fire (Figure 2b) situations. Undoubtedly, the most affected areas were found to the north of the study area, identified in reddish and brownish tones.
The calculation of the area burned without discriminating by severity level showed that 242,554.6 ha of the reserve were burned. Therefore, it was possible to state that in 40 days 19.4 % of the total area of the IR was burned, at a rate of 6,000 ha/day.
The results by protected areas showed that the most affected portal was Cambyretá (69 %), followed by San Nicolás/San Alonso (49.6 %), Carambola (37.3 %) and Rincón del Socorro (30.7 %), respectively. It should be noted that the portals are access areas to the reserve, which can be nationally or provincially administered.
In total, one out of every five hectares of the IR burned, while the value increased to one out of every two if we count only the portals under study. This means that the impact of fires was greater in the protected areas under study than in the reserve in general.
The severity results showed a predominance of moderately low to high values in the IR, with a low severity sector located in the northern center of the reserve (Figure 2c).
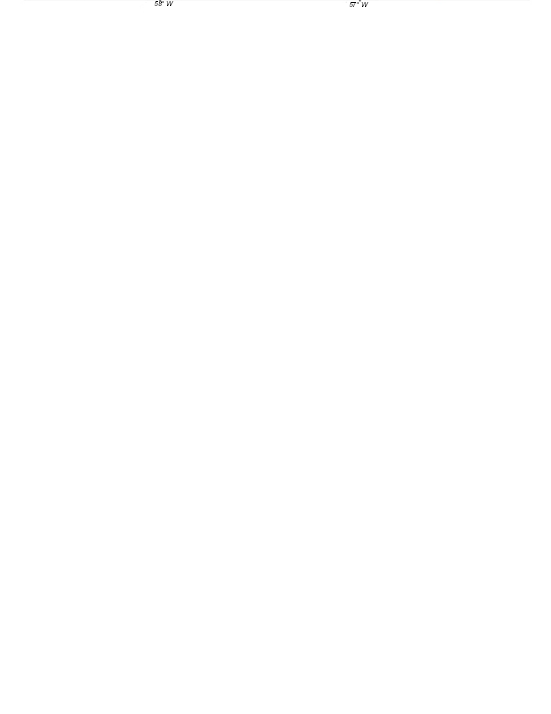
Figure 2 Comparison between pre-fire and post-fire images of Iberá Reserve during the first months of 2022. a) Pre-fire image; b) Post-fire image; c) Severity of burned areas
Figure 3 shows the absolute areas burned for each of the portals, the total sum of these and those corresponding to the Iberá Reserve (IR) as a whole. In all cases, moderate severity levels predominated, especially high moderate severity, except in Rincón del Socorro, where high severity is higher than low moderate severity.
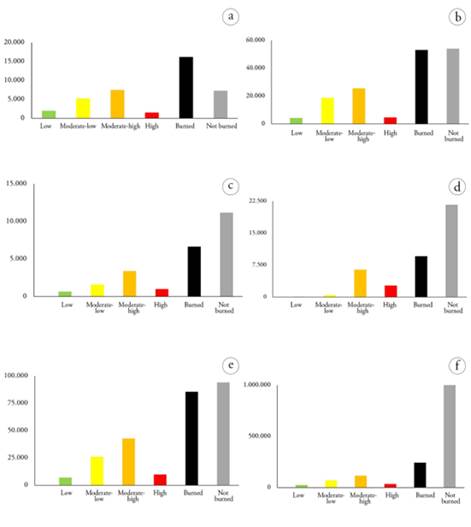
Figure 3 Areas burned according to severity level by portals, in the portals as a whole and Iberá Reserve between January and February 2022. a) Cambyretá; b) San Alonso/San Nicolás; c) Carambola; d) Rincón del Socorro; e) Sum of portals; f) Iberá Reserve.
Figure 4 shows the percentage of burned areas according to severity levels. The first thing that can be seen is the high levels of burn severity, since if the high severity and high moderate severity classes are added together, the percentage exceeds 50 % in all cases. At the same time, it was possible to note that in general, there was a pattern of severity that was repeated in all the areas analyzed.
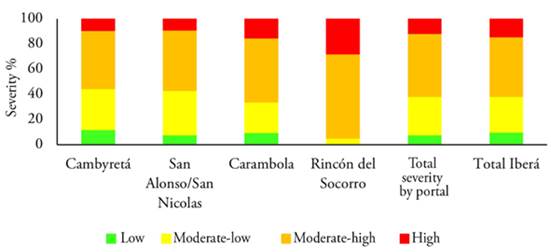
Figure 4 Percentage of burned areas by severity by portals and in the entire Iberá macrosystem (province of Corrientes, Republic of Argentina) between January and March, 2022.
Although Cambyretá was the most affected portal in terms of burned area, it was the second most affected in terms of percentage of high severity (9.2 %) and the least affected by high moderate severity. In addition, if the two highest severity levels are added, the result is 55.3 %, the lowest percentage of all the portals. On the other hand, the Rincón del Socorro portal has the highest severity levels (27.8 % high severity and 66.9 % high moderate severity) and the lowest affected area (30.7 %). This could indicate that, although Cambyretá was the most affected portal in terms of surface area, it is the least affected in terms of severity. Therefore, it could be inferred that it is possible that post-fire recovery is faster. On the other hand, the burned areas in Rincón del Socorro may take longer to recover from the disturbance, given that the severity was greater, perhaps requiring a greater fire management intervention.
The availability of dry fuel biomass is one of the factors related to fire expansion. However, within the ecosystem and natural cycles that can develop within wetlands, there is methanogenesis, which refers to the production of methane (Romero-Mariscal et al. 2023). According to Nicho Alvarado et al. (2021), this gas contributes 15 % of the greenhouse effect, which is mostly formed in wetlands. According to the authors, in most natural wetlands with neutral pH, methane would accumulate at high levels during anaerobic decomposition.
Regarding the burned areas, and setting aside the natural aspects mentioned above, the results showed that, proportionally, the most affected areas of the Iberá IR were those with greater human intervention. Whether due to greater development of urban infrastructure or agricultural and livestock activities, the interaction between society and nature is a determining factor in increasing the probability of fire occurrence.
However, the question could be raised as to how areas that are protected and intended for conservation have been affected. At this point, it is worth noting that most of the different portals were originally private property, and even today it is possible to detect livestock and forestry activity within them.
On the other hand, there are no buffer areas or transition spaces that in some way act as firebreaks or at least prevent direct contact between human development and the space to be conserved. Likewise, despite being an area that is protected or with greater restrictions in terms of the anthropic practices that are conducted there, it does not guarantee that fires will be avoided.
Vasco-lucio et al. (2022) state that fire severity, understood as the ecological change caused by this disturbance, can be assessed from direct observation of damage levels or from remote sensing techniques based on changes in the reflectivity of burned areas (Montorio Llovería et al. 2014). As opposed to visual estimation in the field, which is highly costly in time and money, remote observation provides information with a large spatial coverage, quickly and continuously (Patterson & Yool, 1998), so it is important and should be constant the search for indexes, models and techniques that allow the correct estimation of severity with spectral information (Montorio Llovería et al. 2014). In this regard, the use of Google Earth Engine has been notably extended in recent years, since it allows the processing required for the study of fire severity in a fast and efficient way (Anaya et al. 2018; Smichowski et al. 2022, Long et al. 2019; Wang et al. 2022).
In this platform it is possible to find the complete series of images from various satellite systems such as Landsat, Sentinel or MODIS in the cloud, which have geometric and radiometric corrections. This added to the possibility of making mosaics and spatial statistics with the application of simple scripts, decreases processing times significantly compared to traditional remote sensing (Perilla & Mas, 2020; Gorelick et al. 2017; Anaya et al. 2018).
Within the range of indexes developed, the Normalized Burn Ratio (NBR) has undoubtedly been the most widely used, as it comparatively improves the results obtained by other indexes (Epting et al. 2005; Hudak et al. 2007; Tanase et al. 2011). The NBR index presents good results in the evaluation of fire in general. However, it should be considered that it simply reports the state of the vegetation at the moment, regardless of whether the damage is caused specifically by fire or by other factors such as, for example, pests (Flores-Rodríguez et al. 2021). In addition, it is important to consider that burned areas reported with NBR can be confused with water bodies or bare rock areas (Heredia Laclaustra et al. 2003), and the presence of smoke in active foci also interferes with the detection of burned areas. In landscapes associated with the study area, it has been shown that dry wetland vegetation generates errors in the overestimation of burned areas (Smichowski et al. 2021), which makes it necessary to conduct careful controls of the results obtained.
These problems can be solved with the application of dNBR since, by comparing the situation before and after the fire, it improves the delimitation of the area effectively affected by the passage of fire. At the same time, it allows detecting the severity with which the fire acted in different places by measuring the magnitude of changes in surface cover (Key & Benson 2006). However, considering that in most cases fires occur in times of drought, many times the changes produced by water stress in the environment between the pre- and post-fire image are shown in the dNBR as mainly low severity levels (Anaya et al. 2018). On the other hand, changes in land use such as harvesting of agricultural or forest plots, clearing of forest patches or woodlands, opening of land for urban lots, or the presence of surface water can also be confused with areas affected by fire (Key & Benson 2006; Flores-Rodríguez et al. 2021). Many of these drawbacks can be solved by optical analysis perfomed by users, who must be trained to detect fire scars. This is why the combination of remote sensing tools with optical analysis in geographic information systems is of vital importance, as it can make up for some of the shortcomings of automatic processing (Chuvieco, 2008, Smichowski et al. 2021, Saucedo et al. 2023).
The use of Sentinel 2A imagery was appropriate. Although it has a better spatial, spectral and radiometric resolution than Landsat 8, the most significant for this case was the temporal resolution of 5 days compared to 16 days for Landsat. Undoubtedly, this makes it possible to obtain information closer in time to the event that needs to be monitored (Guerrero et al. 2017). This is important in the case of Iberá where most of the vegetation affected by disturbances is fast recovering.
Regarding the results obtained with the severity analysis, it is interesting to note that high severity degrees dominated, in general (Figure 4) which would evidence that mainly tree species were burned (López García, 2020; Kitzberger & Grosfeld, 2016). However, recent studies conducted in the area show that areas with streams and marshland vegetation, which is the predominant vegetation in the Iberá area, were burned (Saucedo et al. 2023). This may be due to the fact that wetland vegetation has high reflectance, therefore, the difference between the pre-fire and post-fire image is high and, consequently, it is classified as high severity. Another point in question, and as described above, the generation of methane by the decomposition of biomass generates greater heat intensity than the burning of the biomass itself. In this regard, it would be interesting to cross-check severity levels with a breakdown according to the different environments burned to better adjust the severity levels.
In summary, although the fires can be associated with the drought context, it is important to consider the factors that contributed to their spread, especially in wetlands. In this regard, low rainfall, high evaporation rates, the presence of dry biomass, the reduction of both the area covered and the water level of wetlands, plus conditions conducive to methane oxidation, could be the main natural triggers of fire outbreaks, which in turn would explain the differences in severity in the different portals studied.
In this regard, the use of Google Earth Engine has made it possible to optimize the time and resources needed to obtain information of great value, not only because of its veracity, but also because of its importance for the evaluation of the severity of the disaster. In conclusion, the use of this methodology constitutes a significant input in fire monitoring, which makes it a powerful tool for the planning of regulations or measures that contribute to the mitigation, management and early warning of fires.