The soybean [Glycine max (L.) Merrill] is the most important crop cultivated in Brazil. During the period 2015 - 2016, the country's total production was 95.63 million tons, produced on 33.17 million hectares (Embrapa, 2016). Nowadays, the soybean crop is largely cultivated and consumed worldwide, being an important agricultural product destined for animal feed and human food, thus highly demanded in the agrobusiness world (Conab, 2016).
High-quality seeds are necessary to obtain an optimal seedling population. High physiological quality soybean seeds will grow into plants with greater morphological and biochemical performance, thereby increasing the chance of better development and yield (Kolchinski et al., 2005). Brazilian agricultural researchers have developed new soybean cultivars with expressive yield potential, substantiating the crop importance in the international market and export trade.
In the agricultural production system, it is a challenge to uniformly distribute, in the soil, the nutrients that are required in small amounts by the plants. Seed treatments have enabled plants to have higher performance and full genetic potential achievement through the application of pesticides, biological products, plants stimulants, and inoculants, as well as the use of micronutrients, such as silicon (Daronco, 2013). In this sense, seed treatment might be an alternative to maximize agricultural yield (Oliveira et al., 2013), making it necessary the use of techniques directed to improve legume plants mineral nutrition.
The nutritional essentiality of silicon is controversial. Some researchers claim it is essential to plants, while others refer to it as a beneficial nutrient. Some studies concerning silicon have revealed increasing in the physical and structural resistance against biotic and abiotic factors in several species caused by an increase in the cell wall, middle lamella thickness, and rigidity, because of the application of silicon with phenolic compounds and pectins (Datnoff et al., 2007; Currie and Perry, 2007). Moreover, silicon might reduce aluminum toxicity, as well as other micronutrients toxicity, such as manganese, iron, and sodium (Castro and Crusciol, 2013).
The potassium silicate (K2SiO3) fertilization brings several benefits to the soybean crop. It makes leaves more upright, decreasing the plants self-shading. It also enhances the tolerance to lodging and the plants' resistance to several stresses through the accumulation of silicon in their tissues. Also, potassium influences stomatal activity and improve gaseous exchanges in plants while silicon can protect them from pathogens and phytophagous insects, boosting the plants' development and yield (Wise et al., 2007; Zelin et al., 2011).
Machado and Queiroz (2018) reported that the treatment of soybean seeds with silicon increases the vigor of the seeds under laboratory conditions, but the agronomic characteristics in the field were not influenced. Oliveira et al. (2015) also pointed out that the application of silicon does not impair the physiological quality of soybean seeds. According to these authors, the agronomic characteristics of the cultivars are not influenced by the doses of silicon, as well as the number of vegetables per plant. Foliar application of potassium silicate in maize, enhanced the plants' photosynthetic efficiency (Sousa et al., 2010). Ferreira (2008) verified positive effects on the photosynthesis, transpiration rate, stomatal conductance and internal CO2 concentration of cotton plants treated with sodium metasilicate solution. In this sense, the aim of this study was to evaluate the influence of silicon application via seeds on the initial growth of three soybean cultivars (Glycine max L.).
MATERIALS AND METHODS
The study was carried out from October to November 2016, at the greenhouse of the Center of Agricultural Sciences, in the Universidade Federal de Paraíba, located in the city of Areia, state of Paraíba, Brazil. According to the climate classification of Köppen-Geiger, the As' climate (hot and humid) is observed in the region, which presents a rainy season concentrated between March and July and an average annual rainfall of 1400 mm (Peel et al., 2007).
The experimental design was a randomized block, and the treatments were replicated three times and distributed in a 3×5 factorial scheme, composed by three soybean cultivars (C1 - FTR 1186 IPRO, C2 - FTR 1192 IPRO and C3 - FTR 3190 IPRO) and five silicon doses (0, 30, 60, 90 and 120 g per 100 kg of seeds). The silicon doses were applied via seeds according to the proposed by Oliveira et al. (2014). The silicon doses were prepared with liquid potassium silicate (K2SiO3), which presented the following composition: 130 g L-1 of SiO2 and 130 g L-1 of K2O. The potassium silicate was directly applied in 100 g seed in the following proportion: 0, 230, 460, 690, and 920 mL K2SiO3 100 kg seeds-1, equivalent to the silicon doses determined for the experiment. The seeds were immersed in the solution for 12 h.
The soil used was classified as Haplic Planossoil (Embrapa, 2014), collected in the 0-30 cm depth, from the lands of the Center of Agricultural Sciences, in the Universidade Federal de Paraíba. The physicochemical characteristics of the soil are shown in Table 1. The experimental units were 5-liter pots, where the soybeans were cultivated. The soybean seeds were treated with the fungicide thiophanate-methyl (5 g a.i. per 100 kg of seeds) and the insecticide fipronil (50 g a.i. per 100 kg of seeds). The fertilization was done according to the recommended to the soybean crop.
In each experimental unit, two seeds were sown at a depth of 1 cm. Following germination, plants were thinned to one plant per pot. The plants were irrigated whenever needed in order to maintain the soil at field capacity. The study was conducted until the V5 phenological stage of soy development, according to the classification of Fehr and Caviness (1977).
The emergence speed index (ESI), plant height (cm), leaf area (cm2 plant-1), stem diameter (mm), shoot dry mass (g), root length (cm), root diameter (mm), and root dry mass (g) were measured. Plant height, leaf area, and root length were measured using a millimeter ruler. Stem and root diameter were measured using a pachymeter. Forced air circulation oven and analytical balance (0.001) were used to determine shoot and root dry mass. The emergence speed index was calculated based on Maguire's formula (Maguire, 1962). The leaf area was measured according to the methodology described by Richter et al. (2014).
The data were submitted to ANOVA F-test. Tukey test, at 5% of significant level, was used for the soybean cultivars and polynomial regression analysis for the potassium silicate doses. Data analysis was performed using the SAS software (Cody, 2015).
RESULTS AND DISCUSSION
According to the initial development analyses, the emergence speed index presented no significant effect on the soybean cultivars submitted to different silicon doses. The average emergence speed index was 6.4% (Figure 1). This result might be related to the high-vigor soybean seeds that were used in this study, which led to a faster emergence. Some researchers registered similar results. Pereira et al. (2004) found that seed treatment with potassium silicate did not influence the emergence speed index of rice (Oryza sativa L.).
In irrigated rice, Vieira et al. (2011) observed a decrease in the emergence percentage and the emergence speed index when silicon doses are applied 30 d before sowing. This fact may be due to toxicity generated over extra high calcium silicate doses in the paddy field. These authors stated that highest emergence percentage and emergence speed index were observed in areas that did not receive any calcium silicate dose (0 t ha-1), with an emergence speed index of 3.3 and an emergence percentage of 77%.
Seed treatment with potassium silicate did not influence the soybean plants' height. However, differences were observed between the soybean cultivars (Figure 2). The FTR 1192 IPRO (C2) cultivar presented the highest height, differing only from the FTR 3190 IPRO (C3) cultivar, with mean values of 28.5 and 24.8 cm, respectively. The FTR 1186 IPRO (C1) cultivar average height was 26.7 cm. The FTR 1192 IPRO cultivar has a high potential for rooting and rusticity which may have favored its prominence concerning the FTR 3190 IPRO cultivar.
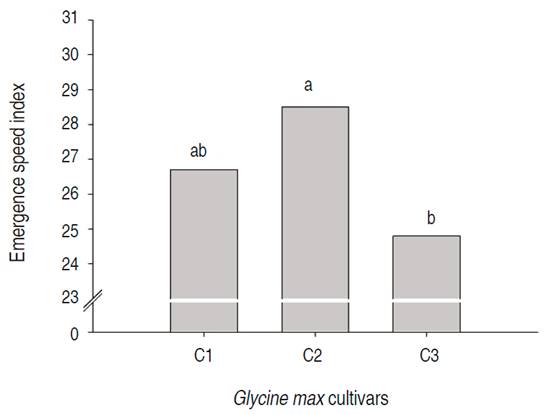
Figure 2 Plant height of three soybean cultivars submitted to silicon fertilization via seeds. Means followed by the same letter do not differ significantly (α=0.05)
Oliveira et al. (2015) studied the effect of seed treatment with different doses of silicon on the height of two soybean genotypes. The silicon treatment did not influence the height of BMX Turbo RR and NA 5909 RR genotypes while plants that received no silicon application reached greater heights. Still, Pereira Júnior (2008) registered higher heights in soybean plants treated with silicon, contrasting the results mentioned above. The BRS MG 68 cultivar presented average height of 91, 96 and 94 cm when treated with 400, 450 and 500 kg Si ha-1, respectively.
Regarding the leaf area, seed treatment with different doses of potassium silicate did not influence the soybean cultivars (Figure 3). According to Takahashi et al. (1990), legume plants usually accumulate low levels of silicon in its cellular structures because they consume silicon in favor of a transpiration flow, which is a slower process when it is compared to water absorption. Besides, silicon is not diffused in the symplastic pathway.
Comparable results to the ones found in this study were observed by Oliveira et al. (2015) that emphasize that the morphological characteristics of the soybean varieties BMX Turbo RR and NA 5909 RR are not influenced with the doses of silicon via seed treatment. Agarie et al. (1998) stated that silicon might be associated with leaf retention because of photosynthesis maintenance and chlorophyll distribution under stress conditions, such as high temperatures and low humidity. This remark might have influenced the greater leaf area and dry mass accumulation in the soybean plants submitted to silicon fertilization at the phenological stages mentioned above.
Silicon is deposited in the plants' structure through root or foliar uptake. It stabilizes in the form of hydrated amorphous silica (SiO2nH2O) with polysaccharides (cellulose and hemicellulose) on cell walls. The presence of silicon makes the leaves more upright, which enhances CO2 uptake, as well as solar radiation exposure. As a result, it might improve soybean photo-assimilates production, directly influencing the crops initial development and yield (Epstein, 1999).
Regarding the stem diameter, potassium silicate treatments did not influence the studied soybean cultivars (Figure 4). However, several authors registered higher stem diameters in maize plants submitted to silicon fertilization (Neri et al., 2009). These increases are probably related to the monocots plants ability to accumulate more silicon through an active uptake process substantially. The silicon accumulates in the lumen and intercellular spaces of Poaceae plants leaves and culms, such as maize and sugar cane (Epstein, 1999).
When studying the effect of micronutrients fertilization and seed inoculants on BRS 206 soybean cultivar, Souza et al. (2009) registered higher stem diameter values compared to the ones found in this study. However, no significant effect was observed between the applied treatments. The higher stem diameter values found by other researchers is related to the different soybean genotypes studied and different phenological stages evaluated.
Regarding the dry shoot mass, significant effect was observed for FTR 1192 IPRO (C2) cultivar, in response to the different doses of potassium silicate applied. The dry shoot mass of C2 cultivar adjusted to the quadratic model, presenting the higher value, approximately 2.1 g, when 120 g of silicon was applied (Figure 5). Because of the greater rooting, when compared with the others, the C2 variety responded better concerning the increase of dry mass. It may be linked to the fact that with a more developed root system the plant can absorb more nutrients and, as a result, develop better.
Sousa et al. (2010) verified significant variations in the dry culm mass of maize plants fertilized with K2SiO3. Soratto et al. (2012) and Figueiredo et al. (2007) reported higher shoot dry mass of strawberry and potato plants fertilized with silicon, respectively. Teodoro et al. (2015) studied the K2SiO3 foliar fertilization effect in the soybean cultivar 5DR615 and verified greater total dry mass production in the crop R6 phenological stage.
Regarding the root parameters, FTR 1186 IPRO (C1) cultivar root length and diameter linearly increased as the K2SiO3 doses increased Figures (6 and 7). The C1 cultivar average values for root length and diameter when treated with 120 g of silicon were 8.7 cm and 4.5 mm, respectively. The FTR 1192 IPRO and FTR 3190 IPRO cultivars root length and diameter presented no significant results in response to the silicon treatment.
The results mentioned above verified for the C1 cultivar might be related to the SiO2 accumulation by the roots. Silicon-non-accumulative species are unable to accumulate and translocate high levels of silicon in their shoots; thus they tend to concentrate this element in the root system, where most of it is deposited in the cell walls (Heine et al., 2005).
Soybean plants translocate low levels of silicon to the shoots, up to 30 mg kg-1. This substantiates the fact that in legume plants, such as soybean and bean plant, there is greater silica (SiO2) accumulation in the roots.
Silicon absorption is mediated by diffusion in soybean and bean plants, through the movement of mono-silicic acid from a high to a low concentration region. This transport process may require energy, due to the silicon's translocation across the water channel proteins, the aquaporins. In this process, silicon molecules retention by the suberin layer present in the root endodermis can occur, making the roots thicker (Raven, 2001).
Silicon is an important element for the soybeans root system, once enhanced nodulation and nitrogen fixation is observed in silicon fertilized soybean crops (Figueiras, 2007). However, some factors must be observed before applying silicon fertilization, such as the genetic characteristics of the cultivar, its ability to consume, translocate and accumulate the element, as well as the soil and environment conditions.
The silicon doses did not influence the roots' dry mass but the different soybean cultivars presented statistical differences among themselves. The FTR 1192 IPRO (C2) cultivar presented the highest root dry mass compared to the other studied cultivars (Figure 8). These results reveal that same species plants (G. max) genotypes, presented different root dry mass accumulation patterns.
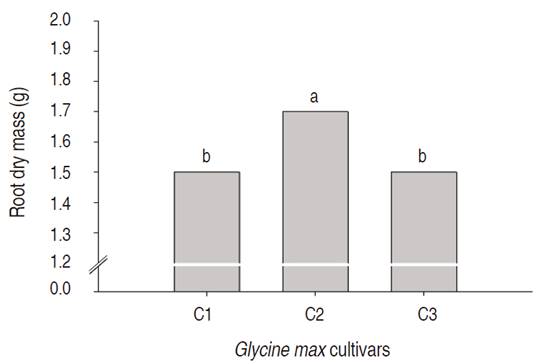
Figure 8 Root dry mass of soybean cultivars submitted to silicon fertilization via seeds. Means followed by the same letter do not differ significantly (α=0.05).
Paula et al. (2007) reported higher roots dry mass in fava d'anta plants (Demorphandra mollis Benth) submitted to potassium silicate fertilization. Still, Ribeiro et al. (2011) verified limited root dry mass accumulation in coffee plants submitted to silicon fertilization after 130 of treatment.
CONCLUSIONS
The treatment of soybean seeds with silicon did not positively influence the root dry mass of the FTR 1192 IPRO variety and the root length and root diameter of the FTR 1186 IPRO variety. The application of silicon did not influence the FTR 3190 IPRO cultivar. Regarding the plant height and dry root mass parameters, the FTR 1192 IPRO cultivar presented more precocity compared to the other genotypes.